Particle accelerator is a device that speeds up the movement of tiny bits of matter. These particles are either ions (electrically charged atoms) or electrically charged subatomic particles, objects that are smaller than an atom. The particles travel through an accelerator in a narrow beam. In accelerating the beam, the machine increases the particles’ energy of motion.
Uses of accelerators.
Physicists use accelerators to discover and study subatomic particles and the forces that govern them. The accelerator forces beams of subatomic particles such as positively charged protons or negatively charged electrons to strike a stationary target or to collide with another beam that is moving in the opposite direction. The resulting impacts break particles away from the target or cause other subatomic particles to form. Devices called particle detectors near the points of impact provide information about particles that fly away from these points.
Other scientists use accelerators to create and study atoms of chemical elements that are heavier than uranium, the heaviest natural element. In this case, nuclei (singular, nucleus) in beams of ions combine with target nuclei to form heavy elements. The nucleus is the dense, core region of an atom, consisting of protons and electrically neutral neutrons.
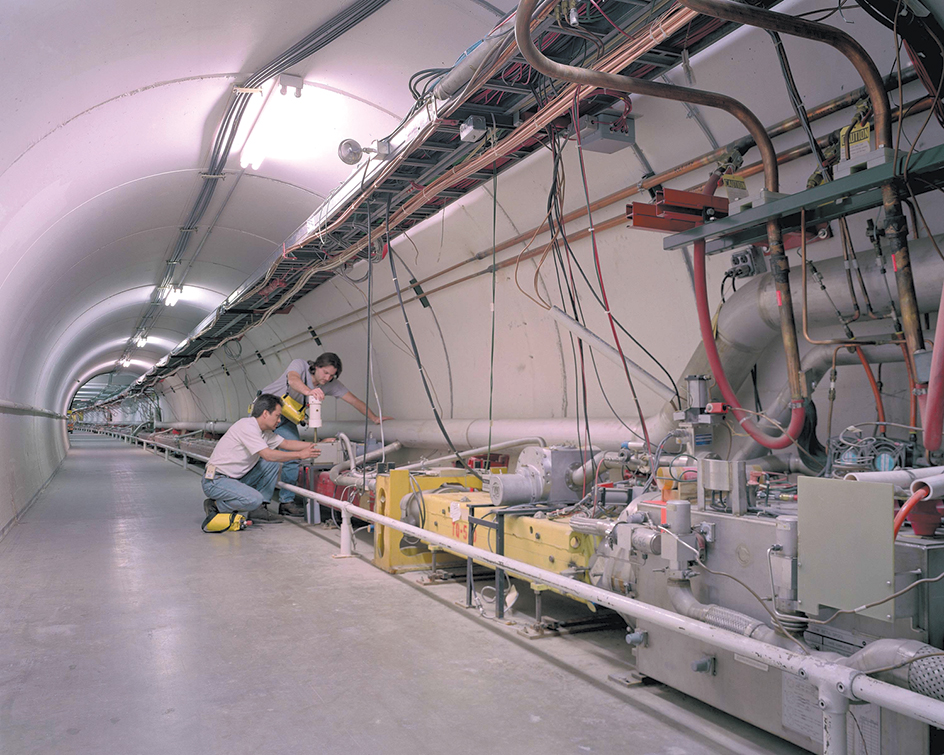
Still other researchers accelerate beams to probe the structure of the atomic nucleus. Because some accelerator experiments break away pieces of nuclei, the machines are sometimes called atom smashers.
Researchers in industry and other areas use intense X rays produced by special electron accelerators. A beam of electrons sends out X rays when it passes through a magnetic field, a region of space in which a moving electric charge is acted on by magnetic force. One use of these rays is to produce transistors on experimental computer chips.

Physicians use particle beams to destroy tissue in cancerous tumors. Doctors also use X rays generated by accelerators to diagnose and treat cancer.
How accelerators work.
Accelerators use electric force to speed up electrically charged particles. To prevent loss of energy from collisions with air molecules, the particles travel through pipes from which almost all the air has been pumped.
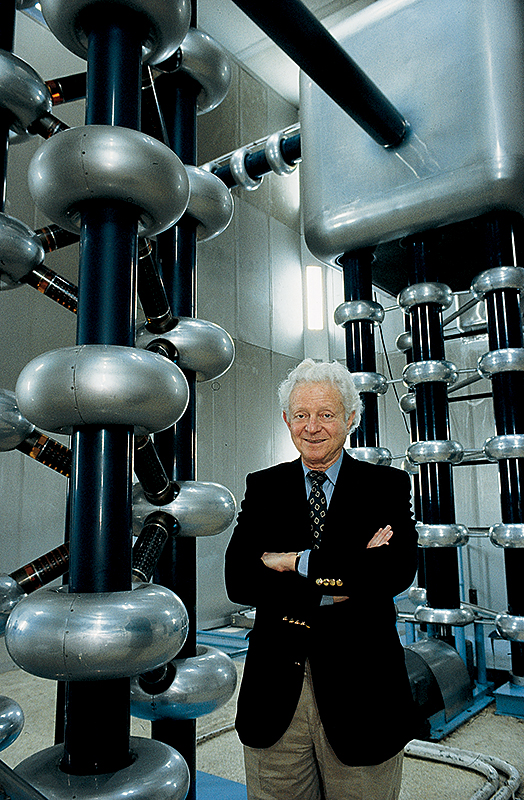
Physicists measure the energy of accelerated particles in electronvolts (eV). One electronvolt is the amount of energy gained by an electron as it moves freely through a potential difference of 1 volt. Accelerators can produce particles with energy in the range of thousands of electronvolts (keV), millions of electronvolts (MeV), billions of electronvolts (GeV), or trillions of electronvolts (TeV).
Accelerators have two basic shapes. In a linear accelerator, the beam travels in a straight line. In a circular accelerator, the particles travel in a nearly circular path. This article describes the main kinds of accelerators.
The Cockcroft-Walton accelerator,
a linear machine, was the first accelerator to break up atomic nuclei. Physicists John D. Cockcroft of the United Kingdom and Ernest T. S. Walton of Ireland invented this machine in 1929. In 1932, they used it to accelerate protons to 500 keV and break up nuclei. Today’s machines speed up protons, electrons, and ions. Most of them serve as beam sources for more powerful accelerators.
A Cockcroft-Walton accelerator has a long pipe with an electrode (electric terminal) at one end. This electrode receives a high voltage of the same kind—positive or negative—as the particles to be accelerated. At the other end is an electrode whose voltage is zero. This electrode has a hole through its center and is connected to the first electrode by a tube that carries the beam.
The machine releases the particles to be accelerated near the charged electrode. The voltage in this electrode repels these particles. The particles fly through the tube and pass through the hole. A Cockcroft-Walton accelerator can boost particles to about 750 keV.
The Van de Graaff generator
can accelerate protons, electrons, and ions to about 15 MeV. United States physicist Robert J. Van de Graaff built his first high energy accelerator in 1931. Hundreds of such accelerators have been built, more than all other types combined.
The machine has a hollow metal sphere mounted at one end of a pipe. A belt runs over a pulley beside a source of electric charge and a pulley next to the sphere. The source puts a charge on the belt. A metallic brush transfers the charge to the sphere. When the charge on the sphere builds to a certain strength, particles that have the same kind of charge—positive or negative—are released near the sphere’s inner surface. These particles plunge to the other end of the machine, pass through a hole, and strike a target.
The tandem Van de Graaff generator
has a sphere at the center of a long pipe. The sphere receives a positive charge. This charge attracts negative ions that are inserted at one end of the pipe. When the ions reach the center, they pass through a thin target that removes two electrons from some of them. The loss of electrons makes these ions positive, so the sphere repels them toward the other end of the pipe. They gain additional energy as they fly to the end of the pipe. A tandem machine can produce ions with twice the energy of a single Van de Graaff generator.
The standing-wave linear accelerator
boosts protons to energies up to 200 MeV by generating an alternating electric field. The field is part of an electromagnetic wave produced by vacuum tubes called klystrons. The wave, called a standing wave, extends the length of a horizontal pipe and vibrates across the pipe’s width.
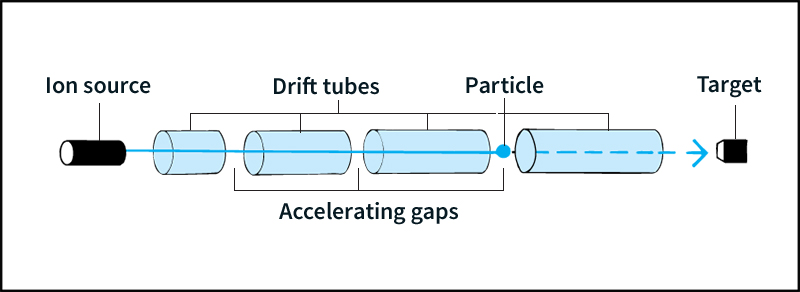
Along the length of the pipe, the field alternates between positive and negative. When the field is positive, it accelerates the protons in the desired direction. But when it is negative, it tends to accelerate them in the opposite direction.
The field does not actually accelerate protons the “wrong way,” however, because the accelerator contains metal drift tubes that block the field. The tubes are installed end-to-end the length of the pipe, with gaps between them. The vibration of the wave is timed so that the protons are inside the tubes when the field would accelerate the protons the “wrong way.”
The traveling-wave linear accelerator
speeds up electrons. A wave generated by klystrons travels the length of a horizontal pipe. The electrons travel with the wave’s negative field, gaining energy as they go—much as a surfer rides an ocean wave. The electrons move so quickly that the positive field cannot catch up with them, so the accelerator needs no drift tubes.
The longest linear accelerator in the world is a traveling-wave device at Stanford Linear Accelerator Center (SLAC) in Palo Alto, California. This machine, which began operating in 1966, is 2 miles (3.2 kilometers) long. In the late 1980’s, SLAC added a circular structure to one end of the machine, enabling it to operate as a two-beam collider. In this case, the machine accelerates a beam of electrons and a beam of positrons, each with an energy of up to 50 GeV. A positron is a form of antimatter—a positively charged counterpart of an electron. In a head-on collision of a matter particle and its antimatter counterpart, all the mass in the two particles turns into energy. An instant later, most of the energy turns into new particles.
The cyclotron
is the simplest circular accelerator. The American physicist Ernest O. Lawrence invented the cyclotron in 1930. He received the 1939 Nobel Prize in physics for this achievement.
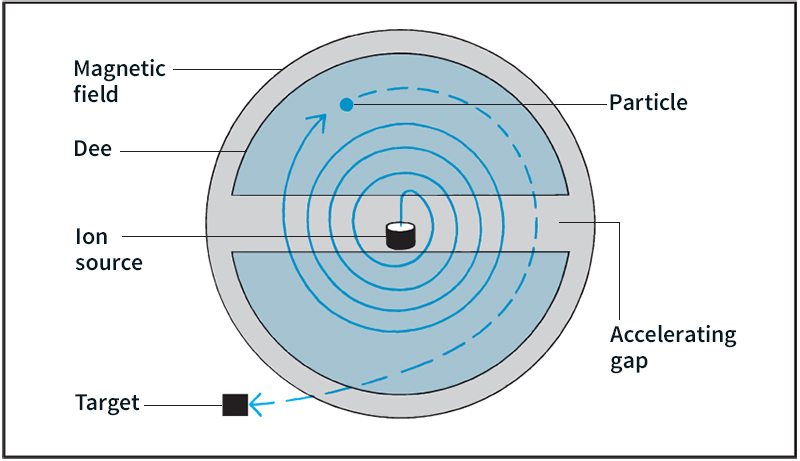
In a cyclotron, the particles travel in a vacuum chamber between the poles of an electromagnet. The chamber also contains two hollow, D-shaped electrodes, mounted with their straight sides facing each other and a gap between them. The cyclotron generates an alternating electric field across the gap.
Charged particles are released at the center of the machine. The electric field accelerates them into one of the electrodes. The electromagnet generates a magnetic field that makes the particles follow a spiral path leading back to the gap. By the time they arrive, the electric field has reversed itself, so the particles are accelerated across the gap in the opposite direction. Again, the particles follow a spiral path and are accelerated across the gap. This process continues until the particles hit a target or spiral out of the cyclotron.
The energy of particles in a cyclotron is limited by the diameter of the magnet and the strength of the magnetic field. The largest cyclotron ever built could accelerate protons to 720 MeV.
The synchrotron
reaches higher energies by guiding particles along a racetrack-like path of alternating straight and curved sections. At each curved section, one or more electromagnets bends the beam through only a relatively small arc. The particles follow the same path over and over. At one or more of the straight sections, the particles pass through a linear accelerator that gives them a boost. As the particles gain speed, the synchrotron automatically increases the magnetic field to hold them on course. The machine also increases the electric field’s rate of vibration—the frequency of the electromagnetic wave—to match the higher speed.
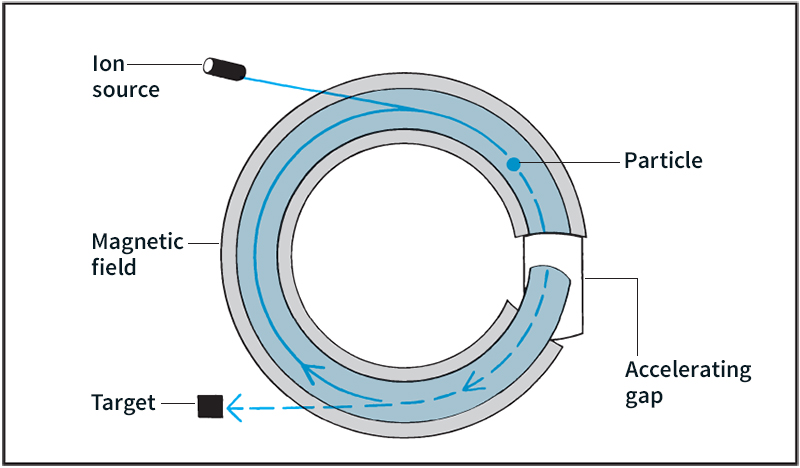
The world’s most powerful accelerator operates at the CERN research center near Geneva, Switzerland. The tunnel measures 17 miles (27 kilometers) around. It housed an accelerator called the Large Electron-Positron (LEP) collider when it began operating in 1989. In 1996, CERN scientists changed its name to LEP2 to reflect a major increase in beam energy. LEP2 collided electrons and positrons at energies up to 104.5 GeV per beam.
CERN shut down LEP2 in 2000 so that construction could begin on another accelerator in the same tunnel. The new machine, the Large Hadron Collider (LHC), was designed to collide two beams of protons at energies up to 7 TeV per beam. The LHC was completed in 2008, but technical problems caused the machine to be shut down for another year. In late 2009, after repairs were completed, the LHC produced a beam of protons of 1.18 TeV, making it the most powerful accelerator in the world. In early 2010, scientists at CERN collided two beams of protons at a record 7 TeV. After shutting down for two years to undergo further upgrades, the LHC produced collisions of 13 TeV in 2015. In 2019, CERN announced plans to build the Future Circular Collider (FCC)—a super collider that would be even more powerful than the LHC.
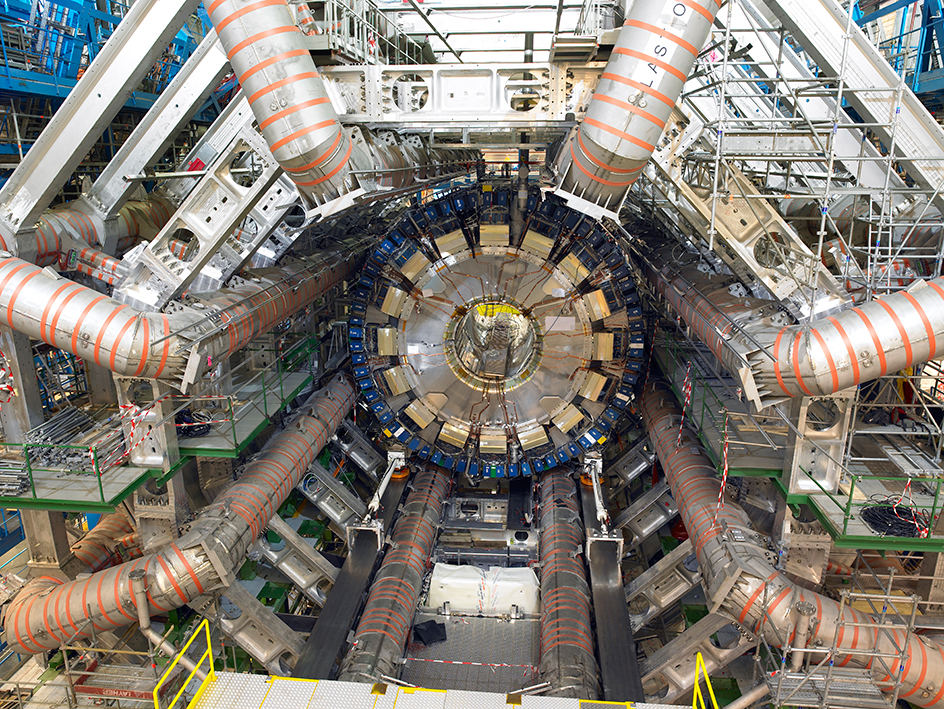
In 2000, a machine called the Relativistic Heavy Ion Collider (RHIC) began operating at Brookhaven National Laboratory on Long Island, New York. The RHIC accelerates gold ions through two tubes that are 2.4 miles (3.9 kilometers) in circumference. The machine operates at energies up to 100 GeV per proton or neutron. The collision of gold ions by the RHIC can produce temperatures around 4 trillion K, more than 250,000 times hotter than the center of the sun. One kelvin (K) equals one Celsius degree above absolute zero (–273.15 °C).
The world’s most powerful accelerator before the LHC was the Tevatron at the Fermi National Accelerator Laboratory (Fermilab) in Batavia, Illinois. This machine lies in a tunnel that measures 39/10 miles (6.3 kilometers) in circumference. In 1972, its first year of operation, the machine accelerated protons to 400 GeV. Beginning in 1987, it collided beams of protons with beams of antiprotons, antimatter counterparts of protons. Each beam reached an energy of about 980 GeV. The U.S. Department of Energy shut down the Tevatron in 2011.