Subatomic particle is a unit of matter smaller than an atom. Subatomic particles include the three major particles found in atoms: (1) protons, which carry a positive electric charge; (2) negatively charged electrons; and (3) electrically neutral neutrons. Protons and neutrons form the nucleus of an atom. Electrons whirl about the nucleus.
Electrons and several other subatomic particles are elementary particles—that is, they are not made up of smaller units of matter. Protons and neutrons are composite particles. They are made up of elementary particles called quarks.
Some subatomic particles do not occur naturally in atoms. Rather, they are produced as a result of collisions involving at least one particle that is moving with an extremely large amount of energy—either a cosmic ray or a particle whose energy has been greatly boosted in a device called a particle accelerator.
For every type of ordinary subatomic particle, there also exists an antiparticle. An antiparticle has the same mass (amount of matter) as its corresponding particle but is opposite in electric charge or certain other properties. For example, the antiparticle of the electron is the positron, which carries a positive electric charge. The antiparticle of the proton is the antiproton, which is negatively charged. If a particle and its antiparticle collide, they destroy each other, releasing energy. When an extremely large amount of energy is released, much of it is quickly converted into particles and antiparticles. See Antimatter.
All particles also have properties of waves, including frequency (rate of vibration) and wavelength (distance between successive wave crests). For information on the wave properties of particles, see Quantum mechanics.
Fundamental forces
Subatomic particles interact with one another mainly through three fundamental forces. These forces, from the strongest to the weakest, are (1) the strong nuclear force, also called the strong interaction; (2) electromagnetism; and (3) the weak nuclear force, also called the weak interaction. A fourth fundamental force, gravity, is relatively unimportant for subatomic particles because it is much weaker than the other three forces.
The strong nuclear force binds protons and neutrons in an atomic nucleus. It also holds together the quarks that make up protons and neutrons. Electromagnetism includes electric and magnetic forces. It holds molecules together and keeps electrons in orbit around an atomic nucleus. The weak nuclear force causes one form of radioactive decay, in which, for example, a neutron changes into a proton in the atomic nucleus and emits (gives off) an electron and an electrically neutral particle called an antineutrino.
The strong and weak forces act over only extremely short distances—one trillionth of a millimeter or less. Electromagnetism can act over any distance.
Kinds of subatomic particles
Physicists distinguish between elementary particles and composite particles. They also classify subatomic particles according to such properties as electric charge, mass, and spin. Spin is a measure of the internal rotation of a particle.
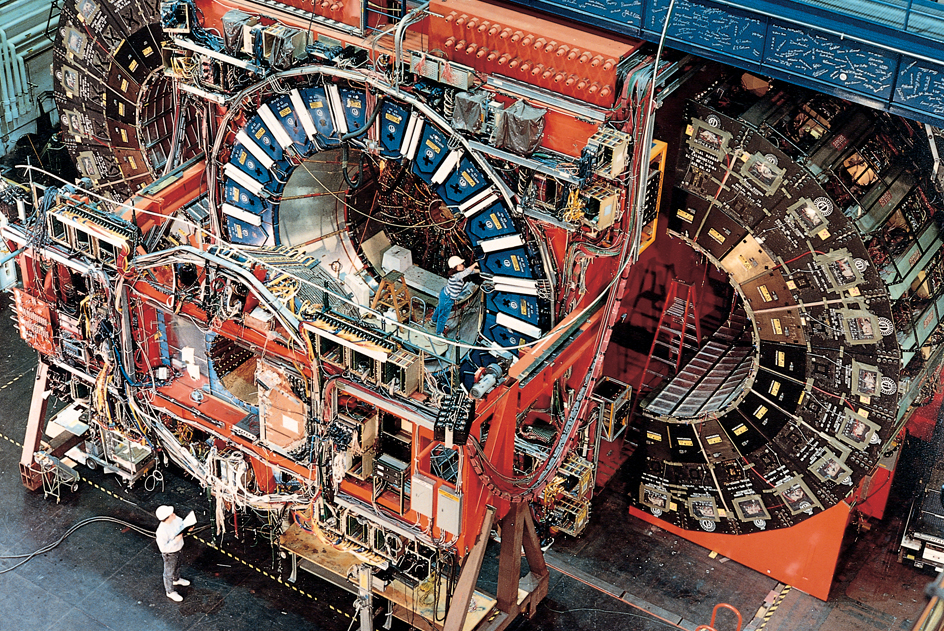
Elementary particles.
Scientists divide elementary particles into three classes: (1) leptons, (2) quarks, and (3) fundamental bosons.
Leptons.
There are six known leptons. These particles are electrons, muons, taus, and three kinds of neutrinos. All leptons have a 1/2 unit of spin. Particles with half-integer spins (1/2 , 3/2, 5/2, and so forth) are called fermions. Each electron, muon, and tau has one unit of negative electric charge.
An electron is an extremely light particle. The mass of an electron is 0.0000000000000000000000000009 gram. That numeral is a decimal point followed by 27 zeros and a 9, or, in scientific notation, 9 x 10-28 (see Scientific notation).
However, scientists usually measure the mass of subatomic particles in terms of equivalent energy. They do so by applying Einstein’s equation E = mc-squared (E=mc2), where E represents the energy, m is mass, and c-squared (c2) is the speed of light multiplied by itself. For the unit of energy, they commonly use 1 million electronvolts, abbreviated MeV, or 1 billion electronvolts (GeV). One electronvolt is the amount of energy gained by an electron as it moves freely through a potential difference of 1 volt (see Volt). In terms of energy, the mass of an electron is 0.51 MeV. A muon is 207 times as massive as an electron; a tau, 3,477 times as massive as an electron.
Neutrinos have no electric charge, but they do have a tiny amount of mass. Their mass has so far proved too small to measure. Leptons have no measurable size; physicists describe them as “pointlike.” See Lepton.
Quarks.
There are six known quarks. They are known as up, down, charm, strange, bottom, and top. Physicists are almost certain there are no more to be found.
Scientists often refer to quarks by the first letter of the names of these particles. For example, a down quark is called a d quark, or simply a d.
The u, c, and t quarks have 2/3 unit of positive electric charge. The d, s, and b quarks have 1/3 unit of negative electric charge. Like leptons, quarks have a 1/2 unit of spin. However, quarks interact by means of the strong force, but leptons do not. The strong force binds quarks together with such strength that they are never found as free particles. They are found only in composite particles known as hadrons. See Hadron.
The lightest quark is the u, whose mass is about 5 MeV. The heaviest is the t. Physicists believe that this particle’s mass is about 175 GeV. Quarks, like electrons, are described as “pointlike.” See Quark.
Fundamental bosons,
also called gauge bosons, make up the third class of elementary particles. Fundamental bosons transmit the fundamental forces, and they have no known smaller parts. All bosons have a whole number value of spin (0, 1, or 2).
A fundamental boson called a photon transmits the electromagnetic force. Thus, all forms of electromagnetic radiation—including visible light—consist of photons. According to the theory of electromagnetism, electrically charged particles interact with one another by exchanging (giving off and absorbing) photons. A photon has no mass, and is pointlike.
The Z boson, which is electrically neutral, and two W bosons, one with a positive charge and one with a negative charge, carry the weak force. Each W boson has a mass of 80 GeV. The mass of the Z boson is 91 GeV.
Eight bosons called gluons transmit the strong force. These bosons “glue” the quarks together to form hadrons. The quarks interact with one another by exchanging gluons. Gluons are always bound in hadrons. Gluons have no mass.
Physicists believe the gravitational force is transmitted by a particle called the graviton. However, the gravitational force is so weak that this particle has never been observed. See Boson; Gluon; Photon.
Composite particles.
Quarks make up composite particles called hadrons. Up to five quarks may be bound into a hadron, though such particles are extremely rare. The two main types of hadrons are baryons and mesons.
Baryons
are composed of three quarks. The most familiar baryons are the proton, composed of two u quarks and a d quark, and the neutron, composed of two d quarks and a u quark.
All atoms of the same chemical element have the same number of protons. Each proton carries one unit of positive electric charge. The mass of a proton is about 938 MeV, or about 1,836 times the mass of an electron.
A neutron has no net electric charge because the charges of its quarks cancel one another out. A neutron’s mass is 940 MeV. Protons and neutrons are about 10-12 millimeter across. See Neutron; Proton.
Experimenters have observed more than 100 kinds of baryons besides protons and neutrons. These other baryons have more mass than protons and neutrons, and they decay quickly. A subatomic particle decays by releasing energy and turning into one or more other particles. See Baryon.
Mesons
are composed of a quark and an antiquark. For example, the positively charged pi meson is composed of a u quark and a d antiquark. All mesons decay quickly and are produced only in particle collisions. Mesons are also classified as bosons because they have a whole value number of spin. Experimenters have observed about 150 kinds of mesons. See Meson.
Theories of forces
Scientists have developed several theories of forces to explain natural phenomena and to predict the outcome of experiments. Researchers are now trying to develop a single theory for all the forces.
Early theories.
The earliest successful theories have become known as classical theories. These theories apply only to objects larger than atoms and molecules. The first such theory was a theory of gravity formulated by the English scientist Isaac Newton in the 1600’s. It was refined by the German-born physicist Albert Einstein in 1915 in his general theory of relativity. In the 1860’s, James Clerk Maxwell, a Scottish physicist and mathematician, developed another classical theory, the theory of electromagnetism.
During the early 1900’s, scientists found that classical theories could not explain the inner workings of atoms. By the 1920’s, they had developed the theory of quantum mechanics to help them understand the physics of such minute objects as atoms. Since then, theories of forces have had to be consistent with the theory of quantum mechanics and with Einstein’s special theory of relativity—published in 1905—to be acceptable to the scientific community. As a result, such theories are often called relativistic quantum theories. See Relativity.
The Standard Model.
Beginning in the 1940’s, physicists developed successful relativistic quantum explanations for electromagnetism, the weak force, and the strong force. All three have the same basic mathematical structure and are known as gauge theories. These three explanations are now considered one collective theory called the Standard Model. Gravity is the only fundamental force that does not have a satisfactory quantum explanation.
The relativistic quantum theory of electromagnetism is called quantum electrodynamics, or QED. This theory was developed during the 1940’s by physicists Richard P. Feynman and Julian S. Schwinger of the United States and Sin-Itiro Tomonaga of Japan.
The electromagnetic and weak forces are closely related. The electroweak theory describes their relationship. It was completed in the early 1970’s by physicists Sheldon L. Glashow and Steven Weinberg of the United States and Abdus Salam of Pakistan.
The theory of the strong interaction, called quantum chromodynamics, or QCD, was proposed by the American physicists David J. Gross and Frank A. Wilczek in 1973, and independently by the American physicist H. David Politzer in 1974. This theory predicted the existence of gluons.
One of the main differences among the three theories concerns the number and types of charges involved in the three forces. Electromagnetism has only one charge, the familiar electric charge. The weak and strong forces have associated with them certain properties that are believed to be similar to, but not exactly the same as, electric charges. The weak force has two such “charges,” called weak isospin, and the strong force has three, fancifully called color. These color “charges” have no relationship to actual colors.
Most gauge theories require that the gauge bosons be massless particles, and photons and gluons meet this requirement. However, the weak gauge bosons, the W and Z particles, are extremely massive. As a result, scientists have concluded that the gauge theory of electroweak interactions can be correct only if there exists another particle, called a Higgs boson, to contribute mass to the W’s and Z. On July 4, 2012, scientists at CERN in Geneva, Switzerland, announced significant evidence of a particle believed to be the Higgs boson. See Standard Model Higgs boson.
Theoretical research
on particles and forces is aimed at the development of a single theory that explains all four fundamental forces. This theory will also have to explain why the leptons and quarks have their particular masses.
In addition, the theory must explain why there are three “families” of subatomic particles. Each family contains a neutral lepton, a negatively charged lepton, a quark with an electric charge of + 2/3, and a quark whose charge is – 1/3.
The first family consists of the electron neutrino, the electron, and the u and d quarks, respectively. All the material around us is made up of only members of the first family.
In the second family are the muon neutrino, the muon, and the c and s quarks. The third family consists of the tau neutrino, the tau, and the t and b quarks.
The members of the second and third families seem to be identical to those of the first family, except for mass. Each member of the second family has more mass than the corresponding member of the first family. Each member of the third family has more mass than the corresponding member of the second family.
One group of theories, called grand unified theories, or GUT’s, describe electroweak and strong forces as aspects of a single force. However, these theories do not deal with gravity. See Grand unified theories.
Another group of theories, superstring theories, attempt to unify all four fundamental forces, including gravity, into a single force. According to these theories, objects called strings underlie all matter and forces. String length is only about 10-32 millimeter.