Superconductivity is the ability of some materials to conduct electric current without resistance at extremely low temperatures. These materials, called superconductors, have many useful properties. Electric current can flow through a superconductor without loss of energy. Superconductors can also produce powerful magnetic fields, some more than 400,000 times stronger than Earth’s magnetic field. A magnetic field is the region around a magnetic object or electric current in which its magnetic force can be detected. The Dutch physicist Heike Kamerlingh Onnes discovered superconductivity in 1911.
Superconductors include many metallic elements, compounds, and alloys (combinations of two or more metals). Some copper oxides (compounds that contain copper and oxygen) and organic (carbon-based) materials can also superconduct. A superconductor loses its resistance below a certain temperature, called the critical temperature. Many metallic superconductors have critical temperatures near absolute zero (0 K or –273.15 °C). Mercury, for example, has a critical temperature of about 4 K (–269 °C). Scientists achieve such low temperatures using expensive cooling equipment with liquid helium as a refrigerant.
Researchers have developed superconductors that work at higher temperatures. One metallic superconductor used in industry, an alloy of niobium and tin, has a critical temperature near 18 K (–255 °C). Some copper oxide ceramics have higher critical temperatures. Yttrium-barium-copper oxide superconducts below about 93 K (–180 °C), and mercury-barium-calcium-copper oxide below 133 K (–140 °C). Liquid nitrogen, which is cheaper and easier to handle than liquid helium, can cool these materials to their critical temperature. A superconducting compound of hydrogen and sulfur, created by squeezing hydrogen sulfide gas under enormous pressure, has the highest known critical temperature, nearly 203 K (–70 °C).
A theory explaining one type of superconductivity was proposed in the mid-1950’s by three American physicists—John Bardeen, Leon Cooper, and John Robert Schrieffer. Their theory is called the BCS theory, from the initials of their last names. Normal conductors consist of positive ions (charged atoms) surrounded by free-moving electrons that can carry electric current. These electrons scatter off the positive ions, creating resistance. In the BCS theory, superconductivity occurs when the electrons stop behaving as individual particles and begin to move in a more organized way.
The organized state consists of electron pairs called Cooper pairs, named for Leon Cooper. Normally, negatively charged electrons repel each other. In superconductivity, a passing electron gently tugs nearby positive ions closer together, leaving behind a trail of slight positive charge. This short-lived charge attracts a second electron toward the first, forming a Cooper pair. When the electrons in a metal are linked as Cooper pairs, they no longer scatter off the positive ions. As a result, they move through the material without resistance.
The BCS theory describes superconductivity in metallic elements and alloys and most compounds. Scientists have not yet developed a model that explains superconductivity in copper oxides and some other compounds.
The need for expensive, bulky cooling equipment limits the use of superconductors. However, they are used in magnetic resonance imaging (MRI) machines, which employ magnetic fields to produce images of tissue inside the body. Physics laboratories use superconducting magnets in devices called particle accelerators that enable scientists to study tiny bits of matter. Scientists and engineers are working to develop cheaper high-temperature superconductors for use in efficient power lines, high-speed electronic devices, and extremely sensitive magnetic field detectors.
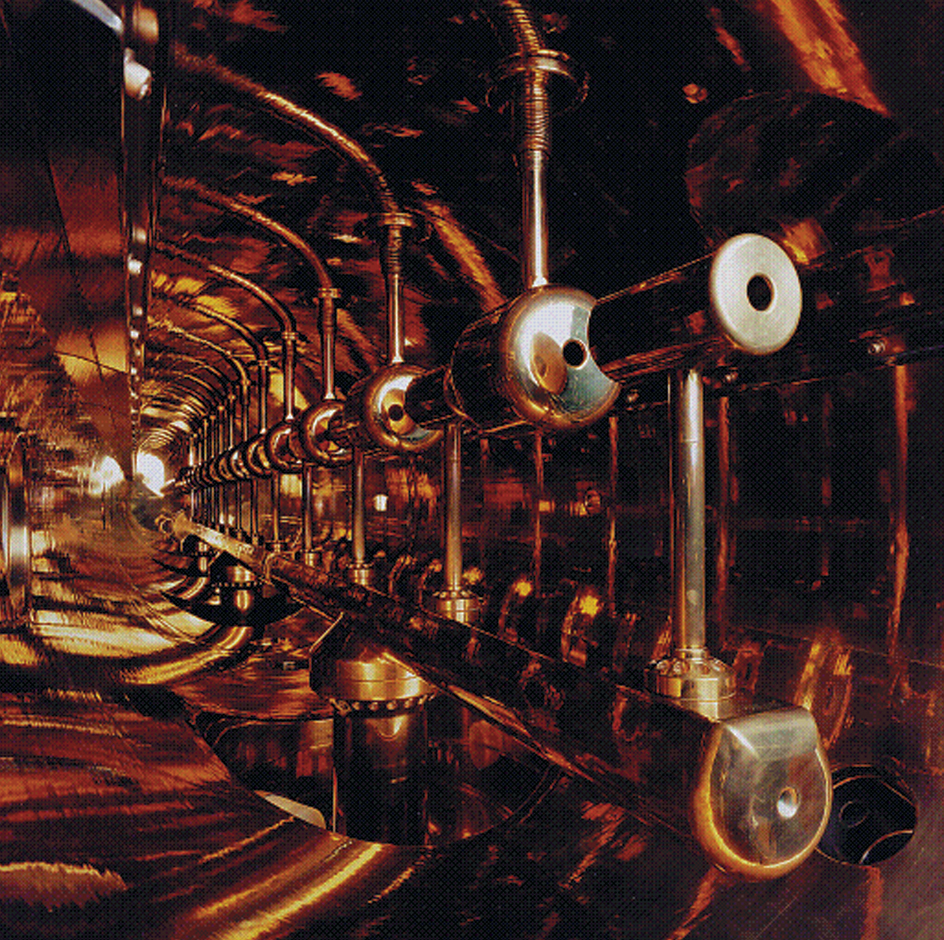