Quantum << KWON tuhm >> entanglement is a special kind of connection that can be shared by particles in quantum mechanics . Quantum mechanics is a branch of physics that describes the behavior of matter . It particularly describes the behavior of atomic and subatomic particles. Subatomic particles are particles smaller than atoms, such as electrons and photons . In quantum mechanics, subatomic particles exhibit certain behaviors that may seem strange or impossible. If two particles are entangled, for example, an action performed on one of the particles instantly affects the state of the other, even if they are separated by vast distances. Scientists and philosophers still debate what conclusions can be drawn from such quantum behavior.
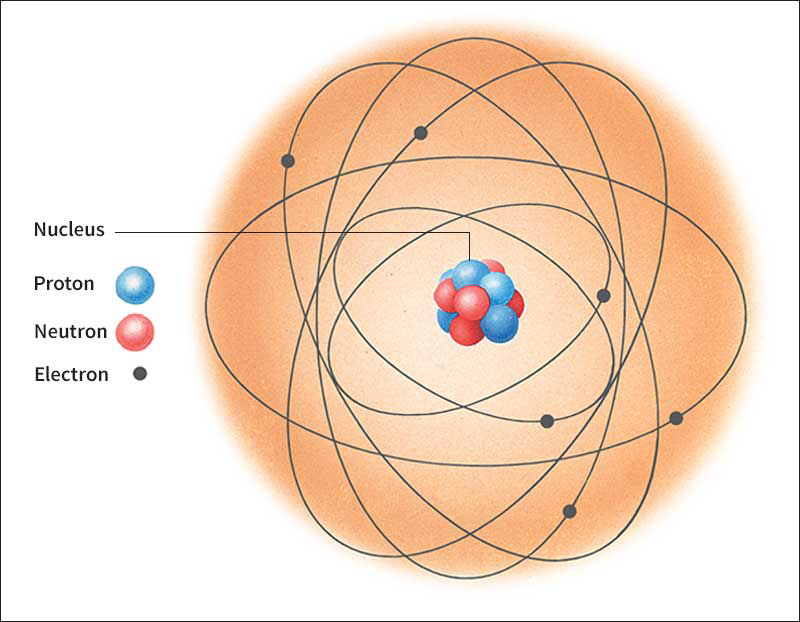
A quantum state describes how a particle will respond to measurements. In quantum mechanics, if a particle can be in one of two different states, then it can also be in a superposition of those two states. For example, the spin (internal rotation) of an electron can be either “up” or “down” relative to a particular direction. So, by the laws of quantum mechanics, it can also be in a superposition of “up” and “down.” A superposition of “up” and “down” is usually thought of as physically distinct from “up” or “down”; both “up” and “down”; and neither “up” nor “down.” In other words, a superposition is a unique physical state that has no counterpart in our everyday experience of reality.
Two quantum particles can be in a superposition such that only the relation between them is definite. For example, two electrons can be in a superposition of both electrons having an “up” spin and both electrons having a “down” spin. The particles may be known to have their spins pointing in the same direction, even if the spin of each particle on its own is not determined. This condition is the characteristic feature of quantum entanglement. Particles that share this condition are said to be entangled.
The EPR thought experiment.
In 1935, the physicists Albert Einstein , Boris Podolsky, and Nathan Rosen published an article that became known as the EPR (Einstein-Podolsky-Rosen) paper. In it, they introduced a thought experiment in which two particles are entangled. In 1951, the American physicist David Bohm reformulated the argument in terms of electron spin. Both the EPR and Bohm versions of the argument involve making one of two different measurements on each of the particles.
A simplified version of the argument goes like this: Suppose two electrons are in a superposition such that their spins are either both “up” or both “down” relative to a particular direction. Over time, the particles travel far apart. According to standard quantum mechanics, neither electron has a definite spin when they are in this superposition. However, if the spin of the first particle is measured, it will be found to have a spin of “up” or “down.” Suppose the measurement finds the particle’s spin to be “up.” At this point, we can predict that a measurement of the second electron must also yield a spin of “up,” since we know that both spins point in the same direction. It is as if the second electron suddenly acquires a definite spin once the first particle is measured. This effect seems to act instantaneously across space, no matter the distance between the particles.
This instantaneous effect can be avoided if we assume that the outcome of the spin measurement on the second electron is pre-determined even before the first electron is measured, even though this is not true in standard quantum mechanics. The original EPR version of the argument involves entangling the positions and speeds of the two electrons. The EPR paper showed that in order to avoid such instantaneous effects, the second electron would have to have both a definite position and a definite speed, which is not allowed in quantum mechanics. See Quantum mechanics (The uncertainty principle) .
Importantly, the result of the spin measurement on the first electron is always completely random and not controllable by the experimenter. Thus, this effect cannot be used, for example, to transmit a message instantaneously.
Einstein established in his theory of special relativity that nothing can travel between two points faster than the speed of light. Light travels at 186,282 miles (299,792 kilometers) per second. This principle applies to forces and the transmission of information, as well as to particles and rays of light. As a result, distant particles cannot have a direct, instantaneous effect on one another. In physics, this idea is known as the principle of locality.
To Einstein, the idea that distant quantum particles could instantly affect each other constituted an absurd violation of the principle of locality. The EPR paper argued, therefore, that quantum mechanics must be incomplete. It argued that a complete theory of quantum mechanics would enable physicists to better predict the properties of such particles as those described in the EPR thought experiment.
The Austrian physicist Erwin Schrödinger responded to the EPR paper by writing two papers in 1935. In his papers, Schrödinger described the particles discussed in the EPR paper as being “entangled,” coining the term. Einstein, skeptical of the idea, famously described the effects of entanglement as “spooky action at a distance.”
Bell’s theorem.
In 1964, the Northern Irish physicist John Stewart Bell wrote a paper titled “On the Einstein Podolsky Rosen Paradox.” In it, he presented a mathematical argument that became known as Bell’s theorem or Bell’s inequality. It was based on Bohm’s version of the EPR argument, involving two electrons with entangled spins.
Bell’s theorem revealed that any possible completion of quantum mechanics would also violate the principle of locality. Measuring the spin of one particle affects the other entangled particle instantly, no matter how far apart the two particles are from each other. In other words, it is not just that our mathematical description of quantum mechanics appears to violate the principle of locality. Rather, the experimental predictions of quantum mechanics themselves are in conflict with locality.
Physicists have experimentally tested Bell’s theorem many times. Three experiments performed in 2015 provided the strongest support to date for Bell’s theorem. However, scientists continue to debate the meaning of the theorem and possible explanations for quantum entanglement.
Applications.
Beginning in the 1980’s, scientists realized that quantum entanglement and superposition could be used to create a quantum computer . Such a computer could calculate and process information in new ways that are believed to be faster than classical computers for certain problems. In classical computing, information is encoded in bits. Each bit is represented by a binary digit, a 1 or 0. A quantum computer, in contrast, uses the quantum bit, or qubit (pronounced KYOO buht) as its basic unit of information. A qubit can take on the value of 1 or 0, but it can also exist in a superposition of both values. It has been shown that the qubits must be entangled in order for quantum computers to outperform classical computers.
Scientists and engineers are considering more potential applications of quantum entanglement. For example, cryptographers (experts in codes and ciphers) hope to use quantum entanglement to send and receive information securely and privately. In 1992, physicists showed that entanglement could be used to move the state of a quantum particle from one laboratory to another without having to transmit the particle itself. This effect is known as quantum teleportation.