Exoplanet is a planet that orbits a star other than the sun. The term exoplanet is short for extrasolar planet. Our galaxy, the Milky Way, probably has at least 100 billion and as many as 1 trillion exoplanets. Astronomers search for and study exoplanets to learn more about how planets form. Exoplanets also serve as places to search for extraterrestrial life (life beyond Earth).
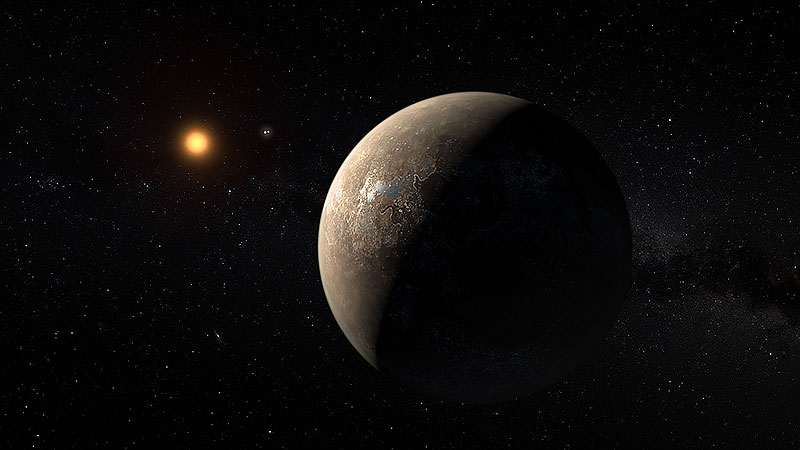
Physical characteristics
Astronomers have found exoplanets with a wide variety of characteristics. Many of these discoveries are so extreme that they challenge our ideas about how planetary systems form and how typical our solar system is.
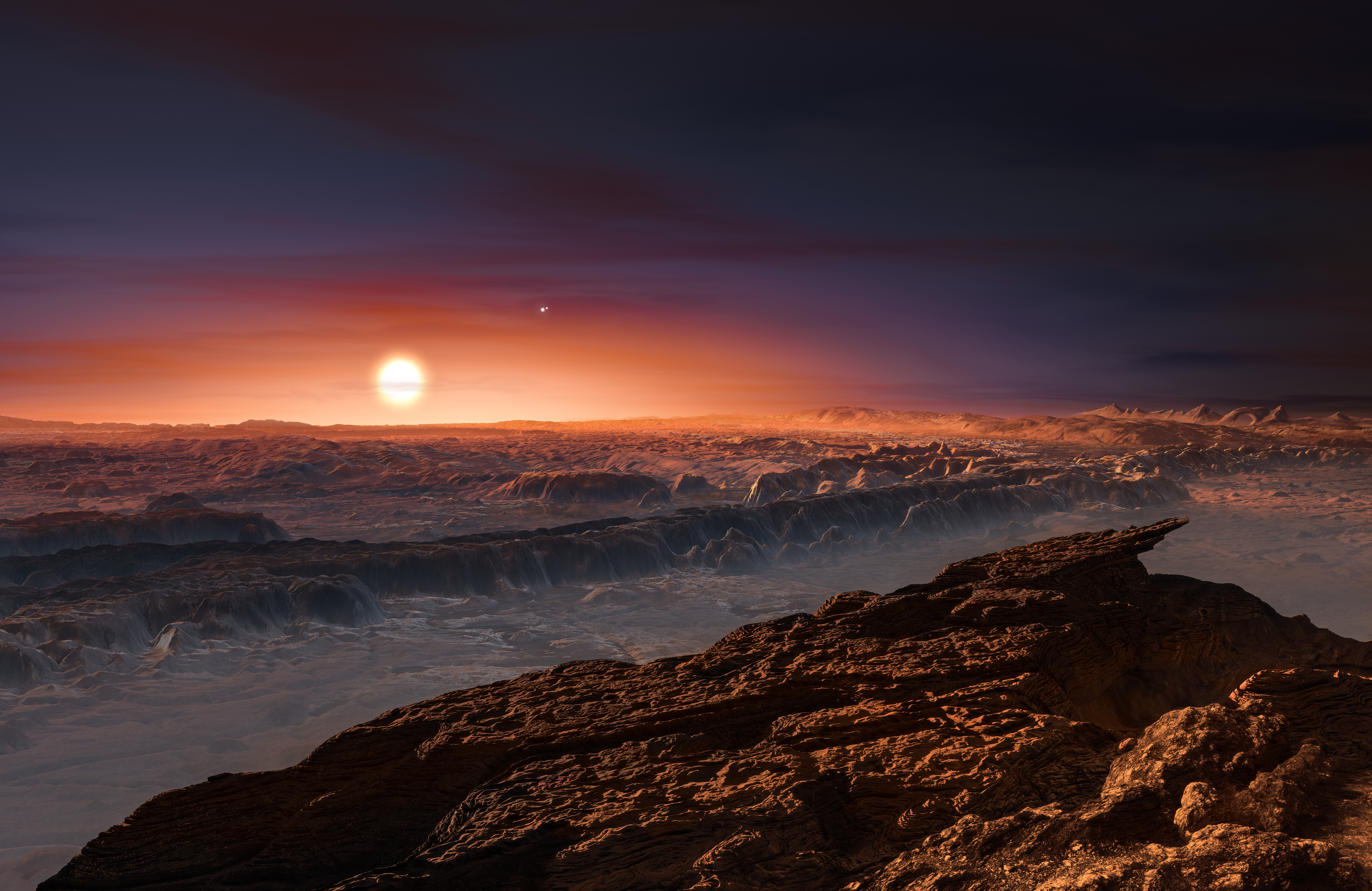
Mass.
Exoplanets can be as small as about 250 miles (400 kilometers) in diameter, depending on their composition (makeup). At this size, a body’s mass may be great enough for its own gravitational pull to compact it into a round ball. Bodies made of ice can become round at a smaller size than can bodies made of rock. Exoplanets can also be many times larger than Jupiter, the largest planet in our solar system. An exoplanet can have a mass up to 13 times that of Jupiter. Beyond this mass, any body smaller than a star is generally classified as a brown dwarf.
The less massive an exoplanet is, the more difficult it is to detect. For this reason, astronomers have confirmed the existence of fewer low-mass exoplanets. Kepler 37b is one of the smallest known exoplanets in terms of both diameter and mass. It is larger than Earth’s moon but smaller than the planet Mercury.
Size and density.
Astronomers must know the size of a mid-mass exoplanet to determine if it is a gas giant similar to Uranus or Neptune, or a terrestrial planet similar to Earth or Venus. Terrestrial planets consist mostly of rock and metal. The density of an exoplanet is related to its mass and size. If two exoplanets have the same mass, the one with the greater diameter will have the lower density. An exoplanet with a larger diameter—and therefore a lower density—is more likely to be a gas giant. An exoplanet with a smaller diameter, and therefore a higher density, is more likely to be a terrestrial planet. Some gas giant exoplanets appear to be far less dense than any of the planets in our solar system. Scientists do not yet completely understand why these “puffy” exoplanets have such low densities.
Orbital characteristics
In our solar system, planets orbit the sun as close as 36 million miles (58 million kilometers) and as far as 2.8 billion miles (4.3 billion kilometers). By comparison, many known exoplanets orbit extremely close to their parent stars. For example, seven exoplanets orbit the small, cool star TRAPPIST-1, and even the most distant of them has an orbit far smaller than that of Mercury, the planet closest to our sun.
A planet’s orbital period is the time it takes the planet to completely revolve around its parent star. Earth, for example, has an orbital period of one year. The closer a planet is to the parent star, the shorter its orbital period. Many exoplanets are so close to their parent stars that they have an orbital period that is just days—or even just hours—long.
Astronomers usually detect an exoplanet through the effect of its movement on the light of the parent star. This fact makes exoplanets with shorter orbital periods easier to discover, in part because they enable astronomers to observe the effects of many orbital cycles in a reasonable amount of time. However, astronomers have found some exoplanets with extremely long orbital periods. A few known exoplanets take more than 100,000 Earth years to orbit their parent stars.
Planets orbit their parent stars in elliptical orbits. An ellipse is a geometric figure with the shape of a flattened hoop. In such an orbit, a planet is closer to its parent star at one part of its orbit and farther away at another. The elliptical orbits of many exoplanets differ little from a perfect circle. But many others have highly elliptical orbits, plunging toward their parent stars before spending many Earth years far away. For example, an exoplanet called 16 Cygni b follows an elliptical path that takes it alternately closer to its star than Venus is to our sun and then farther from its star than Mars is from our sun. Other exoplanets have been discovered in nearly circular orbits, but retrograde (moving in the opposite direction) to the parent star’s spin and nearly perpendicular to the star’s axis of rotation.
Planetary systems
A star or stars and the exoplanets in orbit around them are together called a planetary system or extrasolar system. Most extrasolar systems that have been discovered consist of a star and a single exoplanet. But others contain two or more exoplanets. Several known extrasolar systems have six or more exoplanets. At least one has eight exoplanets, as many as the number of planets in our solar system.
Stars do not always occur on their own, as does our sun. Many points of light visible in the night sky are actually binary stars, two stars orbiting their common center of mass. Such binary stars can, in turn, orbit other stars or even other binary stars. Astronomers have discovered exoplanets in two-star systems and in four-star systems. Exoplanets also form around stars that make up groups of stars called star clusters. However, gravitational interactions with nearby stars eject many such exoplanets into space. Such free-floating planetary bodies are called rogue planets.
Our sun is just one type of star. Exoplanets can be found around many kinds of stars, from dwarf stars to giant stars. Some astronomers think that exoplanets are not likely to exist around supergiant stars, because these stars have lifespans too short to allow for the development of exoplanets.
Exoplanets could theoretically exist around black holes, though none have been found. Such exoplanets could form from the debris of a supergiant star’s supernova (stellar explosion) and orbit its collapsed core—the black hole. Astronomers also think that planets could form around the supermassive black holes found at the center of most galaxies, such as the Milky Way galaxy’s Sagittarius A*.
Types of exoplanets
Exoplanets come in nearly every shape and size. Many of the exoplanets discovered have characteristics unlike anything in our solar system. Some of the common types of exoplanets include (1) hot Jupiters, (2) lava worlds and chthonian exoplanets, and (3) super-Earths and mini-Neptunes.
Hot Jupiters.
Some gas giant exoplanets are much closer to their parent star than Mercury is to our sun. Astronomers refer to such planets as hot Jupiters because of their size and relatively high temperature, resulting from their nearness to the star. In our solar system, all the giant planets are at much greater distances from the sun. The existence of hot Jupiters has caused astronomers to question standard theories on where giant planets form and whether such planets can migrate inward after their formation.
Lava worlds and chthonian exoplanets.
Earth-sized exoplanets have also been discovered close to the stars they orbit. Some are so close that the exoplanet’s surface temperature must be extremely high. The surfaces of these exoplanets should be an ocean of magma, and their atmospheres should consist of vaporized rock. Some may have been hot Jupiters whose thick atmospheres were stripped away by the powerful stellar wind of the parent star, leaving only a rocky core. Stellar wind is a flow of charged particles given off by a star. Astronomers call such hypothetical remnants of hot Jupiters chthonian exoplanets. Chthonian is an ancient Greek term referring to the underworld.
Super-Earths and mini-Neptunes.
Many exoplanets are larger than Earth—the largest terrestrial planet in our solar system—but smaller than Neptune, the smallest gas giant in our solar system. Terrestrial exoplanets of this size are called super-Earths. Many of them probably have a thick atmosphere like that of Earth or Venus, but some may have a thin atmosphere or none at all. Other exoplanets of this size may have a smaller rocky or metallic core wrapped in thick layers of clouds. Such exoplanets are called mini-Neptunes. Life as we know it would be more likely to exist on super-Earths than on mini-Neptunes. Therefore, differentiating the two through observation and determining how they form is important in the search for alien life. Some astronomers think that some mini-Neptunes may in fact be rocky super-Earths whose atmospheres have been expanded by the heat of their parent stars.
Detecting and confirming exoplanets
Astronomers use several methods to search for exoplanets. The four most common methods make use of: (1) direct imaging, (2) gravitational microlensing, (3) radial velocity, and (4) transits. Once a measurement is taken that appears to indicate the presence of a planet around a distant star, scientists refer to the planet as an exoplanet candidate. To confirm the existence of an exoplanet, astronomers must detect a candidate’s signature using a second method. Astronomers must also determine the size and the mass of the object.
Direct imaging.
Astronomers can use ground-based or orbiting telescopes to photograph the area around a dim star over the course of months or years. Photographs can only produce extremely low-resolution photos of objects as distant as exoplanets. Resolution is a measure of the ability of an image to show detail. However, using the direct imaging technique over time, astronomers may gather enough data to draw conclusions about an exoplanet’s basic features, such as the makeup of cloud bands in its atmosphere.
The great distance of exoplanets and their nearness to bright stars makes it extremely difficult to image them directly. Astronomers have detected fewer than 100 exoplanets using direct imaging.
Gravitational microlensing.
A star passing between Earth and another star can act like a magnifying lens. The gravitational pull of the foreground star bends and focuses light passing near it, causing the background star to appear brighter. This effect is called gravitational microlensing. Astronomers can detect planets orbiting the foreground star by observing changes in the light received from the background star over months or years. Astronomers have detected over 100 exoplanets by using gravitational microlensing.
Radial velocity.
In the radial velocity method, astronomers detect tiny, regular changes in the light coming from a star over a long period. The changes in light can be caused by the gravitational pull of an orbiting planet.
Astronomers use a technique called spectroscopy to detect these changes. Spectroscopy involves breaking down light into its component colors, called a spectrum. Every star shines with a unique spectrum that depends upon the star’s chemical composition and temperature. Chemical elements in the star absorb light at very precise colors, leaving dark bands in the spectrum called spectral absorption lines. Spectral absorption lines can shift position slightly as the pull of an orbiting planet tugs a star alternately toward and away from Earth.
The shifts in a star’s spectral absorption lines are due to the Doppler effect. The Doppler effect is the change in frequency of sound, light, or other waves caused by the relative motion of the wave’s source. The spectral absorption lines shift slightly toward the color blue as the star is tugged closer to Earth. They shift slightly toward red as the star is pulled farther away. Such changes can not only reveal the presence of an exoplanet, but they can also be used to determine the exoplanet’s mass and orbital period. Astronomers have discovered hundreds of exoplanets using the radial velocity method. Loading the player...
Doppler effect
Transit.
A small fraction of stars are oriented in such a way that an orbiting planet will pass in front of the star, as seen from Earth. Such an event is called a transit. A transiting exoplanet blocks some of the light from the parent star, causing it to dim slightly. Astronomers can measure the size of such a planet by the amount of dimming it causes. The time between transits reveals the orbital period of the exoplanet. Astronomers can use the orbital period to deduce the exoplanet’s distance from the star and its mass. This technique, known as the transit method, has been used to discover thousands of exoplanets.
Validating exoplanets.
The transit method has enabled astronomers to quickly identify candidate exoplanets. In fact, so many exoplanet candidates have been identified using the transit method that astronomers lack the resources to confirm them all. Thousands of such candidates have not yet been confirmed. Instead of confirming them, astronomers can validate (provide evidence for) some exoplanet candidates by using less strict criteria. Astronomers consider an exoplanet candidate validated if another telescope records the candidate by using the same observational method. A candidate is also considered to be validated if the chance of the observation being caused by some other effect is less than 1 percent. Such validation is called probabilistic validation. Astronomers and mathematicians use special algorithms (mathematical procedures) and computer programs that make use of artificial intelligence techniques to perform probabilistic validations.
Exoplanets and the search for alien life
One of the most important reasons to study exoplanets is to search for alien life. Life could have arisen in conditions completely different from those found on Earth. But it is difficult to determine what those conditions might be. For this reason, many astrobiologists focus on planets with Earthlike conditions. Astrobiologists are scientists who specialize in the search for life in space.
Habitable zones.
Liquid water is necessary for life as we know it to exist. Close to a star, temperatures are so high that water can exist only as a vapor. Far away from a star, water remains frozen as ice. Around each star, however, there exists a range of possible orbits in which liquid water could exist on the surface of an exoplanet. Scientists call this region around a star its habitable zone. The size of a star’s habitable zone and the zone’s distance from the star depend on the star’s type. For example, a type of relatively cool, small star called a red dwarf has a habitable zone that is very close to it. Hotter stars will have habitable zones farther away.
Size and atmosphere.
Even within the habitable zone, liquid water will evaporate when exposed to vacuum (airless) conditions. Therefore, an exoplanet within its star’s habitable zone must also have a sufficiently thick atmosphere to be considered potentially habitable. The ability of a planet to hold on to an atmosphere depends on its mass and on its distance from its parent star, as well as on the parent star’s type and activity level. For example, a planet much smaller than Earth would not have enough gravitational pull to prevent the stellar wind from stripping away most of its atmosphere shortly after its formation. On the other hand, exoplanets about 1 1/2 times Earth’s mass would likely retain a thick envelope of hydrogen and helium, like the gas giants of our solar system. Therefore, the planets most likely to be habitable have approximately the same mass as Earth.
Other considerations.
Certain stars are more volatile than others, emitting periodic bursts of radiation harmful to life as we know it. Such bursts could periodically sterilize exoplanets within the star’s habitable zone, preventing life from gaining a foothold.
Scientists are learning that oceans of liquid water exist beneath the icy or rocky surfaces of many moons and dwarf planets in our solar system, including Callisto, Ceres, Enceladus, Europa, Ganymede, Mimas, Pluto, Titan, and Triton. If these oceans are found to be capable of harboring life, that would greatly change scientists’ ideas about a star’s habitable zone.
History of study
Philosophers and theologians have speculated on the existence of distant worlds—including ones that might harbor life—throughout the ages. But before scientists learned more about the sun, the solar system, and the other stars, these ideas remained little more than guesses.
In 1584, the Italian philosopher Giordano Bruno suggested that the stars are suns with orbiting planets of their own. He thought that some of those planets or their moons might be inhabited. In the 1800’s, the Italian priest and astronomer Angelo Secchi studied the spectroscopy of stars. Comparing their spectra to that of the sun, he helped confirm that the sun was a star. This insight opened the possibility that other stars could in fact have planets orbiting them as well. Due to the extreme distances of the stars and the difficulty of studying areas near them, however, exoplanets eluded discovery for over another century.
The first exoplanets were discovered in 1991. They were three terrestrial-sized planets orbiting the pulsar PSR B1257+12, in the constellation Virgo. Pulsars are rapidly rotating dead stars that have collapsed to a diameter as small as 12 miles (20 kilometers).
In March 2009, the United States National Aeronautics and Space Administration (NASA) launched a space-based telescope called Kepler designed to search for exoplanets. Over two separate missions from 2009 to 2018, Kepler discovered thousands of exoplanets.
In 2016, astronomers discovered an exoplanet orbiting within the habitable zone of its star. That star, Proxima Centauri, is the star nearest to the sun. It is 4.2 light-years from Earth. A light-year is the distance light travels in a year, about 5.88 trillion miles (9.46 trillion kilometers). Astronomers have determined that the exoplanet, called Proxima Centauri b, is slightly larger than Earth and probably has a rocky surface.
In 2017, astronomers announced that they had detected seven Earth-sized exoplanets orbiting the red dwarf star TRAPPIST-1, about 40 light-years from Earth. Three of these exoplanets orbit within the star’s habitable zone. Precise density measurements suggest that those three exoplanets have oceans of water deeper than Earth’s oceans. Loading the player...
Kepler spacecraft
In 2018, astronomers using the European Southern Observatory (ESO) Very Large Telescope (VLT) captured an image of a new planet forming around the distant star PDS 70. The planet, named PDS 70b, can be seen clearing a path through a disk of material from which it formed around its parent star. Astronomers determined that the planet is about as close to its parent star as Uranus is to our sun. PDS 70b is a gas giant, several times more massive than Jupiter. In addition, the planet is heated by its rapid accumulation of disk material, making its surface more than three times hotter than any planet in our solar system.
The VLT searches for exoplanets and planet-forming disks around nearby stars using a technique known as high-contrast imaging. This technique makes use of a coronagraph, a circular shade that blocks out the extremely bright light of the central star. Astronomers then use special data-processing techniques to image planetary companions orbiting the star. The ESO results are especially important for helping scientists understand the early steps in the formation of giant planets.
In December 2019, the European Space Agency (ESA) launched the Characterising Exoplanet Satellite, abbreviated CHEOPS. The satellite was designed to use the transit method in observing nearby stars that are known to host exoplanets. CHEOPS studies exoplanets larger than Earth but smaller than Neptune to determine their potential habitability.
The James Webb Space Telescope, a space-based observatory, was launched in 2021. The telescope studies the atmospheres of exoplanets to search for the chemical signatures of life.