Jupiter is the largest planet in our solar system. It has a mass (amount of matter) that is greater than the masses of all the other planets in the solar system added together. Astronomers call Jupiter a gas giant because the planet consists mostly of hydrogen and helium, and has no solid surface. Jupiter is named for the king of the gods in Roman mythology.
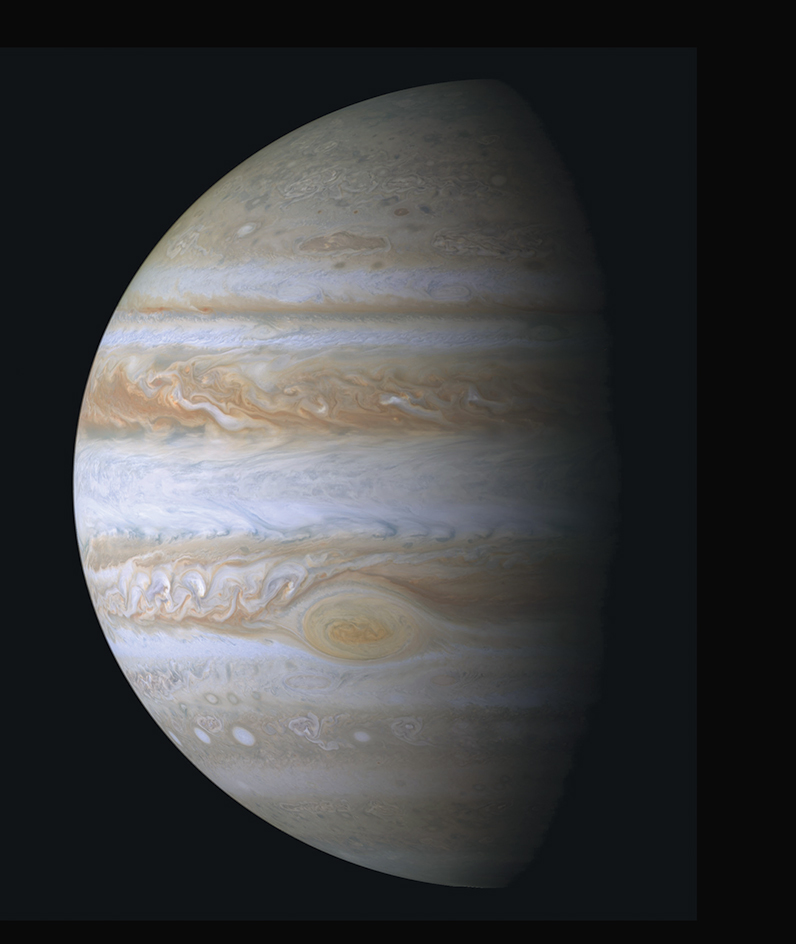
Jupiter ranks among the brightest objects in the night sky. Usually, only the moon and Venus appear brighter. When seen with the unaided eye, Jupiter has a pale orange color. Viewed through a telescope or in images taken by spacecraft, Jupiter appears as a globe covered with swirling, brightly colored clouds of brown, orange, white, and yellow.
Jupiter lies at the center of a system of cosmic objects so vast and diverse that it resembles a miniature solar system. The planet has 16 moons that measure at least 6 miles (10 kilometers) in diameter and dozens of smaller satellites. Four faint rings of dust particles encircle the planet. Jupiter also has a strong magnetic field. A magnetic field is the area around a magnet in which its influence can be detected. Jupiter’s magnetic field extends beyond the planet throughout a huge region of space called the magnetosphere. Astronomers sometimes refer to the planet together with its rings, satellites, and magnetosphere as the Jovian system.
Astronomers have made detailed observations of Jupiter for centuries. It was one of the first planets studied by the famous Italian astronomer Galileo in the early 1600’s. Beginning in the 1970’s, several spacecraft have explored the Jovian system in great detail.
Characteristics of Jupiter
Astronomers determine Jupiter’s characteristics using observations made through telescopes and by spacecraft. They study these characteristics to learn about the planet’s structure and origin.
Orbit and rotation.
Like all the planets in our solar system, Jupiter travels around the sun in an elliptical (oval-shaped) orbit. Jupiter’s orbit is nearly circular. It lies about five times as far from the sun as Earth’s orbit does. The average distance between the sun and Jupiter measures around 484 million miles (779 million kilometers). Jupiter’s orbit is tilted by 1.3 degrees from the ecliptic plane, the imaginary plane that contains Earth’s orbit. Jupiter takes nearly 12 Earth years to orbit the sun.
Jupiter rotates faster than any other planet, taking about 9 hours 56 minutes to turn completely on its axis. This is the length of a day on Jupiter. The planet’s rapid spinning causes it to bulge slightly at the equator. Its diameter at the equator measures 88,846 miles (142,984 kilometers), while the distance between its geographic poles—the ends of its axis—measures only 83,082 miles (133,708 kilometers).
Like Earth’s axis, Jupiter’s axis is not perpendicular to the planet’s orbital plane, the imaginary plane that contains its orbit. Jupiter’s axis lies tilted from the perpendicular by 3.1 degrees. As a result of this tilt and its motion about the sun, Jupiter, like Earth, has seasons.
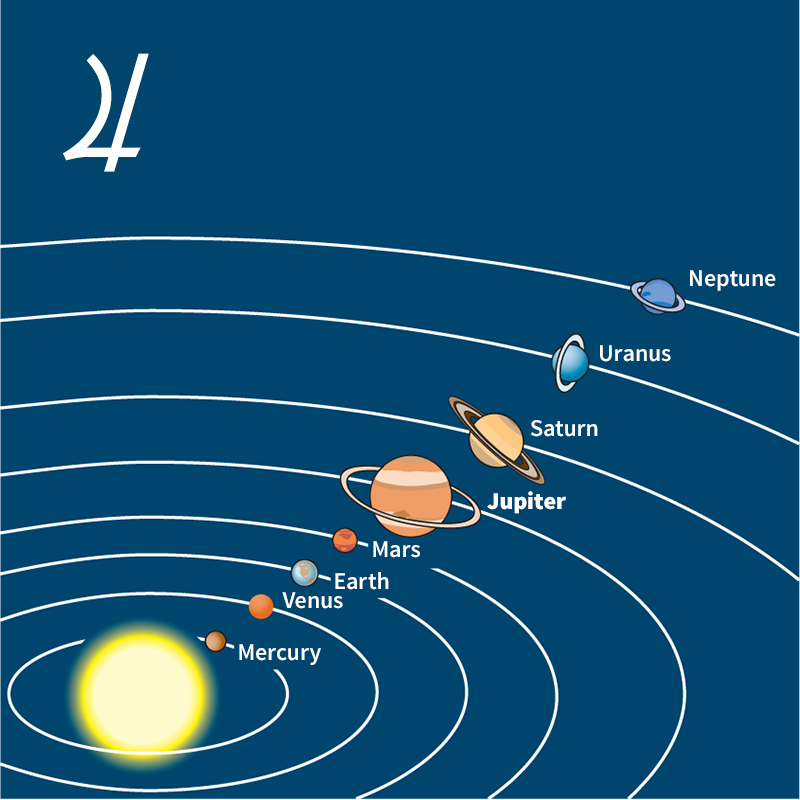
Mass and density.
Jupiter’s mass is about 318 times as great as the mass of Earth, but about 1,000 times smaller than that of the sun. Jupiter’s average density is about 1.3 times as great as the density of water at room temperature. This density is much lower than the density of Earth. Jupiter’s low density indicates that it is composed mostly of light elements rather than rock.
Chemical composition.
Jupiter’s elemental composition resembles that of the sun. The planet consists mostly of hydrogen (chemical symbol, H) and helium (He). It also contains small amounts of heavier elements, including oxygen (O), carbon (C), nitrogen (N), sulfur (S), and many others. In general, Jupiter has a higher concentration of heavy elements than does the sun.
Most of the elements in Jupiter’s atmosphere consist of atoms linked together in molecules. Molecules that have been detected in Jupiter’s atmosphere include molecular hydrogen (H2), water (H2O), ammonia (NH3), methane (CH4), and hydrogen sulfide (H2S). Smaller amounts of other molecules form in chemical reactions in the atmosphere. These include ethane (C2H6), ethene (C2H4), ethyne (C2H2, also called acetylene), hydrogen cyanide (HCN), and other compounds. Helium exists as individual atoms in Jupiter’s atmosphere.
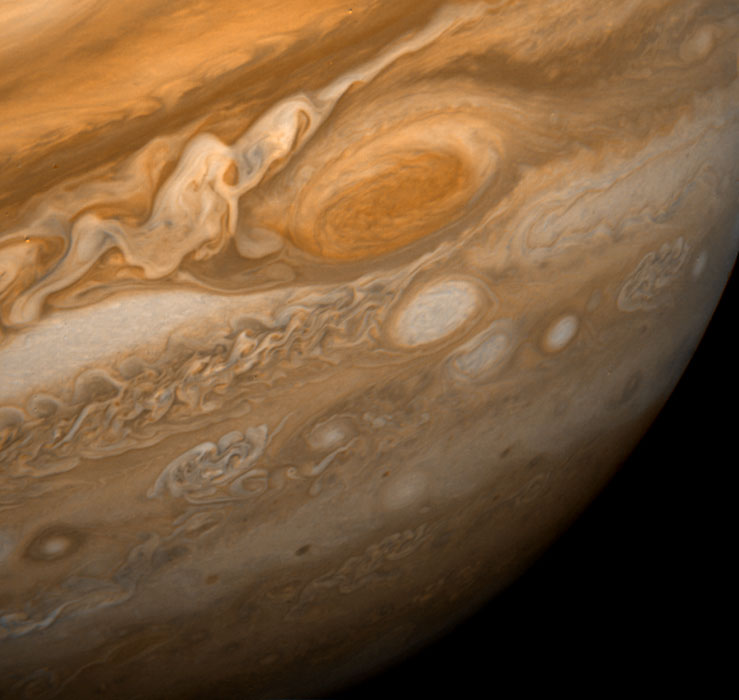
Temperature.
The actual temperature of Jupiter varies with altitude and location, but because of its great distance from the sun, Jupiter is much colder than Earth. Scientists have different ways of discussing the temperature of gaseous planets like Jupiter. One common approach gives the average temperature for an elevation that corresponds to a certain amount of pressure. At an altitude corresponding to 1 bar of pressure, Jupiter averages -162 °F (-108 °C). The bar is a unit of pressure in the metric system, equal to 100 kilopascals.
The amount of energy delivered in a certain period of time is called power. The power that Earth radiates equals the power it absorbs from the sun. Jupiter, however, radiates about twice as much power as it absorbs from the sun. This indicates that some of Jupiter’s energy comes from a source other than the sun. The energy may be heat left over from Jupiter’s formation. It might also come from heat created as the planet slowly contracts under the influence of gravity. Although Jupiter gives off some of its own energy, astronomers do not consider it a star because no nuclear reactions occur in its interior.
Magnetic field.
Jupiter’s magnetic field is mainly dipolar—that is, Jupiter has a magnetic north and south pole like the poles on a bar magnet. Physicists describe the overall strength of such a field using a measure called the magnetic dipole moment. Jupiter’s magnetic dipole moment measures about 20,000 times as strong as that of Earth. Like Earth’s magnetic field, Jupiter’s field lies tilted by about 10 degrees from the planet’s axis of rotation. Jupiter’s magnetic poles are aligned opposite those of Earth. A compass needle that points north on Earth would point south on Jupiter.
Jupiter’s magnetic field traps electrically charged particles, such as electrons and ions (charged atoms or groups of atoms). As a result, the magnetosphere contains a hot, low-density plasma, a form of matter made up of charged particles. The plasma is concentrated in a thin disk near the planet’s equator. It comes from Jupiter’s moons, especially Io. Io has active volcanoes that eject much sulfur and oxygen into the magnetosphere. The hot plasma can damage the optics and electronics of spacecraft operating in the Jovian system. The magnetosphere also deflects the solar wind around the Jovian system. The solar wind is a continuous flow of charged particles from the sun.
Telescopes on Earth can detect the glowing ions in Jupiter’s magnetosphere. The ions also produce visible effects when they enter Jupiter’s atmosphere near the poles. There, collisions between the charged particles and the atmosphere create bands and streamers of light called auroras. Jupiter’s auroras glow brighter than those of any other planet in the solar system.
Radio emissions.
Astronomers discovered Jupiter’s magnetic field in 1955 when they detected radio waves emitted (given off) by the planet. The radio emissions result from the movement of electrons in Jupiter’s magnetic field. Some electrons travel through the field in a spiral path at high speeds. Electrons that move in this way emit radio waves in a process called synchrotron radiation. Observations show that synchrotron radiation creates some of Jupiter’s radio emissions. Other emissions result from electrons moving between Io and Jupiter and from electrons moving within the atoms of Jupiter’s atmosphere.
Jupiter’s radio emissions vary in strength in a pattern that repeats about every 9 hours 55 minutes. Astronomers think the planet’s magnetic field takes this long to complete one rotation. They also use this value for Jupiter’s rotation period because the planet lacks solid features that can be used to measure its rotation.
Structure of Jupiter
Astronomers know more about Jupiter’s atmosphere than they do about the planet’s interior because the atmosphere is the part of the planet that we can see. The pressure of the atmosphere grows steadily as altitude decreases until the atmosphere merges gradually into the interior. Astronomers use observations of the planet and their knowledge of chemistry and physics to determine what its interior might be like.
Atmosphere.
Temperatures in the uppermost parts of Jupiter’s atmosphere measure about 1500 °F (800 °C). Throughout the upper atmosphere, temperatures drop as altitude decreases. Temperatures reach their lowest in a region where the atmospheric pressure equals about one-fifth the pressure at the surface of Earth. This area, called the tropopause, separates Jupiter’s upper atmosphere from its lower atmosphere. Below the tropopause, temperatures begin to increase approaching the planet’s interior.
Jupiter’s colorfully swirled appearance comes from clouds in its lower atmosphere. Different compounds there condense to form clouds at different altitudes, creating layers of clouds with various chemical compositions. The uppermost clouds consist primarily of ammonia ice. These clouds make up most of what we see when we look at Jupiter. Pure ammonia ice is colorless. The clouds’ colors result from tiny amounts of impurities called chromophores. Astronomers do not know for sure what chromophores are. They may include organic (carbon-based) molecules, sulfur compounds, or phosphorus compounds.
Astronomers think that below the ammonia clouds there is a second cloud layer made up of ammonium hydrosulfide, which forms when ammonia and hydrogen sulfide condense together. A cloud layer composed of water ice may lie deeper still. At greater pressures, far beyond where light can penetrate, the atmosphere may contain clouds of iron or silicates, compounds of metals, silicon, and oxygen that form rocks on Earth.
Zones and belts.
Alternating bands of light and dark clouds cover Jupiter’s atmosphere. Astronomers refer to the wider, brighter bands as zones and to the darker, narrower bands as belts. The zones and belts result from wind patterns in Jupiter’s lower atmosphere.
In the zones, winds blow from the west at speeds that reach up to 400 miles (650 kilometers) per hour near the equator. Winds in the belts blow from the east at slightly lower speeds. The zones appear bright because they contain high-altitude clouds that reflect much sunlight. The clouds in the belts lie at somewhat lower altitudes. Their darker appearance probably results from a higher concentration of chromophores.
Jupiter’s alternating east and west winds result from convection currents. These currents are movements of the atmosphere created by the rising of warm gases and the falling of cooler gases. Because Jupiter’s internal energy heats the atmosphere unevenly, warm gases rise in certain places and cool gases descend in others. This rise and fall creates convection currents.
Jupiter’s rapid rotation bends the convection currents into patterns that stretch east and west around the entire planet. This effect of rotation, known as the Coriolis effect, also creates wind patterns on Earth. On Earth, the patterns vary because oceans, continents, and mountain ranges interfere with the circulation of the atmosphere. On Jupiter, which lacks a solid surface or other obstacles, the east and west winds remain remarkably stable. As a result, while individual bands continuously undergo small changes, the overall pattern of zones and belts has remained unchanged in the hundreds of years since people first observed Jupiter through telescopes.
Ovals.
Jupiter’s atmosphere displays many features that are oval or circular in shape. The most prominent of these is a vast, reddish oval called the Great Red Spot.
The Great Red Spot extends about 7,450 miles (12,000 kilometers) from north to south. The spot’s width from east to west, which is slowly shrinking, measured about 10,500 miles (17,000 kilometers) in the early 2000’s. The spot’s circumference is larger than that of Earth. The spot travels around Jupiter with the wind at about 22 degrees of latitude south of the equator. As with the other clouds on Jupiter, astronomers do not know exactly what causes the spot’s reddish color.
The English scientist Robert Hooke first observed a large spot in Jupiter’s atmosphere in 1664. Astronomers first recorded the Great Red Spot’s precise form and position in 1831. Since then, the spot has remained near the same latitude. Images taken by the two Voyager spacecraft in 1979 revealed that the spot is a swirling cloud of gas that takes about seven days to complete one full rotation. Wind speeds at the outer edges of the spot reach up to 425 miles (685 kilometers) per hour.
Unlike the winds in hurricanes on Earth, which swirl around a low-pressure region, winds in the Great Red Spot and in other long-lived ovals in Jupiter’s atmosphere swirl around areas of high pressure. Scientists call these weather systems anticyclones.
Other oval features in Jupiter’s atmosphere include white ovals. White ovals are much smaller and less stable than the Great Red Spot. They tend to lie on the edges of zones but frequently move around the planet.
In the late 1990’s and early 2000’s, three white ovals merged to form a larger oval that later took on a reddish color. The new spot, often called the Little Red Spot, measured roughly half the size of the Great Red Spot.
Weather.
In addition to these longer-lived atmospheric patterns, such as zones, belts, and ovals, Jupiter has storms and other active weather. Astronomers first saw flashes of lightning on Jupiter’s night side in images from the Voyager spacecraft. Later, the Galileo craft observed numerous lightning flashes and tracked the movement of clouds from night to day. The New Horizons spacecraft saw lightning near Jupiter’s poles. Astronomers determined that the lightning flashes originate in small cloud plumes that resemble thunderheads on Earth. The lightning flashes on Jupiter are much more powerful than those on Earth.
Interior.
Below the clouds, Jupiter’s pressure, temperature, and density increase until the atmosphere gradually blends into the fluid interior. Eventually, the hydrogen and helium that make up most of the planet become more like a liquid than a gas.
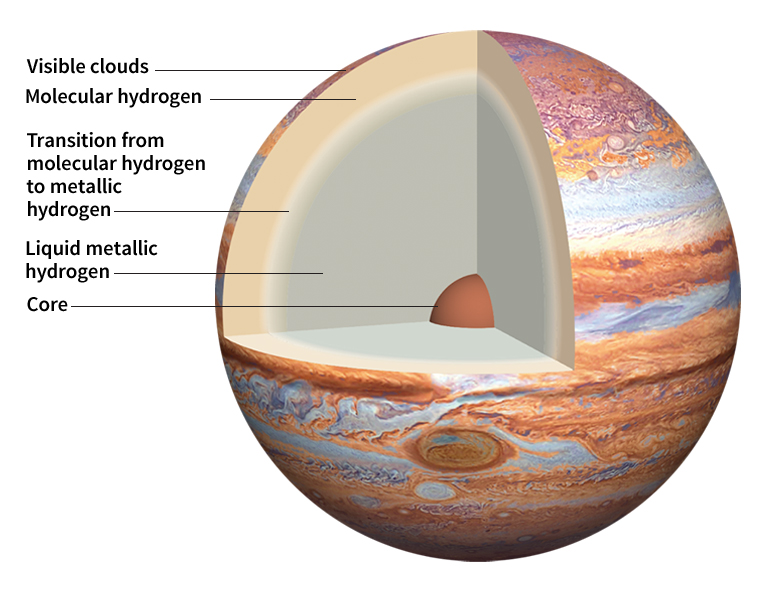
About 6,000 miles (10,000 kilometers) below the clouds, the pressure becomes 1 million times as great as the atmospheric pressure at Earth’s surface. At this depth, hydrogen atoms begin to break down, with the electrons becoming separated from their nuclei. The separated nuclei and electrons compose an unusual form of hydrogen called liquid metallic hydrogen that can conduct electricity like an ordinary metal. Liquid metallic hydrogen makes up most of Jupiter’s mass. Scientists believe that electric currents flowing through this hydrogen generate the planet’s magnetic field.
Core.
The region near Jupiter’s center is difficult to probe. The pressure there equals about 70 million times the pressure at Earth’s surface. Astronomers estimate the temperature of Jupiter’s center to be around 45,000 °F (25,000 °C).
Most scientific models suggest that Jupiter has a dense core made up of substances that, under less severe conditions, would form rock and ice. They estimate the mass of the core to be about 10 to 15 times the mass of Earth. The rock-forming material in the core may include iron and silicates. The ice-forming material may include oxygen, carbon, and nitrogen. Other models indicate that Jupiter has no distinct core. Instead, they suggest that liquid metallic hydrogen merges gradually with heavier elements near the planet’s center.
Satellites and rings
Astronomers have identified at least 95 satellites of Jupiter. Of these, 57 have names. The planet probably has more small moons that have yet to be discovered. Jupiter’s satellites can be divided into three groups: (1) the Galilean satellites; (2) the inner satellites; and (3) the outer satellites. The inner satellites are closely related to Jupiter’s system of rings.
Galilean satellites.
Astronomers call Jupiter’s four largest moons the Galilean satellites because Galileo discovered them. In order of increasing distance from the planet, they are Io, Europa, Ganymede, and Callisto.
Io,
the innermost Galilean satellite, ranks as the most geologically active body in the solar system. It has many volcanoes that frequently erupt sulfur dioxide gas. Most of the gas condenses and falls back to Io as ice, but some sulfur and oxygen ions escape into Jupiter’s magnetosphere. Io’s eruptions result from the gravitational pulls of Jupiter, Europa, and Ganymede. These forces pull Io in different directions, squeezing the moon’s interior and causing it to heat up.
Europa
also shows evidence of geological activity caused by the same forces that squeeze Io. Europa’s icy surface features a broken network of fissures (narrow cracks). The fissures may indicate that the moon’s icy crust rests atop deep oceans of liquid water or slushy water ice.
Ganymede
ranks as Jupiter’s largest moon and the largest moon in the solar system. Ganymede is also the only moon in the solar system known to generate its own magnetic field. Its surface features large light and dark regions. The dark regions contain more impact craters than the light regions do. The light regions typically exhibit many grooves and ridges.
Callisto,
the outermost Galilean satellite, is one of the most heavily cratered bodies in the solar system. Impact craters uniformly cover Callisto’s surface.
Inner satellites.
The moons with orbits that lie inside those of the Galilean satellites are known as Jupiter’s inner satellites. In order of increasing distance from the planet, they are Metis, Adrastea, Amalthea, and Thebe.
Compared to the large, round Galilean satellites, the inner satellites are small and irregular in shape. They range from 10 to 104 miles (16 to 167 kilometers) in average diameter. Amalthea is the largest, followed by Thebe, Metis, and Adrastea. Their surfaces all appear dark and red and have many craters from collisions. Astronomers have measured the average density of Amalthea, which is about the same as the density of water on Earth’s surface. Amalthea must be remarkably porous (filled with tiny holes) to have such a low density.
Outer satellites.
Jupiter also has a large number of small, irregular satellites orbiting well beyond the Galilean satellites. Astronomers have discovered dozens of these outer satellites, but their actual number is probably higher. Jupiter’s gravitation can also trap other bodies for a time, making them temporary satellites.
Himalia ranks as the largest of Jupiter’s outer satellites, followed by Elara, Pasiphae, Carme, Sinope, Lysithea, Ananke, and Leda. Ananke, Carme, Pasiphae, and Sinope have retrograde orbits—that is, they orbit in a direction opposite to that of the other satellites and the direction of Jupiter’s rotation. Elara, Himalia, Leda, and Lysithea orbit in the same direction that Jupiter rotates. Unlike the orbits of the inner satellites and the Galilean satellites, the orbits of some outer satellites are tilted by many degrees from Jupiter’s equatorial plane, the imaginary plane that contains the planet’s equator.
Rings.
Jupiter’s four rings consist of fine dust particles, all circling the planet on individual orbits. The rings all lie close to the planet’s equator and are sometimes mistaken for a single ring. Compared to Saturn’s rings, Jupiter’s rings are smaller and fainter, and contain much less mass. In fact, astronomers could not confirm that Jupiter’s rings existed until the two Voyager spacecraft observed them close-up in 1979. Astronomers call the brightest ring the main ring. Its outer edge corresponds to the orbit of Adrastea. A fainter ring called the halo ring lies inside the orbit of Metis. Two faint rings called the gossamer rings lie outside the main ring. Their outer edges correspond to the orbits of Amalthea and Thebe.
Astronomers think that the rings result from collisions between the inner satellites and tiny particles called micrometeoroids. These collisions eject some dust into the space around the moons. The dust particles orbit Jupiter as they fall toward the planet, forming the rings.
Formation of Jupiter
As the largest planet in our solar system, Jupiter plays a central role in our ideas about how the solar system formed. Jupiter and the sun share a similar composition. They likely formed at the same time from the solar nebula. The solar nebula was a giant rotating cloud of gas and dust.
The solar system began to take shape as the solar nebula collapsed under the influence of gravity. As the nebula contracted, its central region heated up while the outer regions remained cool. Around what is now Jupiter’s orbit, temperatures became cold enough for water vapor to freeze into ice crystals.
According to the most widely held theory, ice and other solid material slowly gathered together to form what is now the core of Jupiter. The core grew as it attracted more material from nearby regions. As the core gained mass, its gravitational pull became stronger. Eventually, the core’s gravitational pull became strong enough to capture hydrogen and helium, which were abundant in the solar nebula. For this reason, Jupiter today consists mostly of hydrogen and helium.
Astronomers use the term accretion to refer to the process by which tiny particles accumulate to form giant planets. When two particles collide, they may stick together to form a larger particle. As the process continues, larger and larger bodies collide. This process happens in different locations, creating many large objects called planetesimals. Eventually, many of the planetesimals near what is now Jupiter’s orbit combined to form the planet. Some of the other planetesimals may have formed some of Jupiter’s satellites, while still others may have escaped to great distances and become comets
History of Jupiter study
Jupiter was known to the ancient astronomers, who tracked the planet’s motion across the night sky. Astronomers first studied Jupiter through telescopes in the early 1600’s. In 1610, Galileo discovered what later became known as the Galilean satellites. At the time, many people believed that every cosmic body revolved around Earth. The discovery of moons orbiting another planet helped convince Galileo and others that Earth was not at the center of the universe.
In the late 1960’s, astronomers chose Jupiter as the target of the first spacecraft mission to the outer solar system. They launched the craft, called Pioneer 10, in 1972. On Dec. 3, 1973, Pioneer 10 became the first spacecraft to visit Jupiter when it passed within about 81,000 miles (130,000 kilometers) of the planet’s cloud tops. Pioneer 11 (later renamed Pioneer-Saturn) flew by Jupiter on Dec. 2, 1974. The two Pioneer spacecraft captured images of Jupiter and its moons. They sent back data on the planet’s gravitational pull, magnetic field, radiation belts, atmosphere, and the plasma in its magnetosphere.
Astronomers used data from the Pioneer missions to design the more ambitious Voyager missions. The spacecraft Voyager 1 flew by Jupiter at a distance of about 174,000 miles (280,000 kilometers) on March 5, 1979. Voyager 2 passed within about 449,000 miles (722,000 kilometers) of the planet on July 9, 1979. The Voyager missions revolutionized our understanding of Jupiter. They discovered Jupiter’s intense aurora and numerous features of the planet’s atmosphere. They also confirmed the existence of Jupiter’s rings. The two Voyager craft captured the first close-up views of many of the planet’s moons, revealing them to be much more active and varied than astronomers had expected.
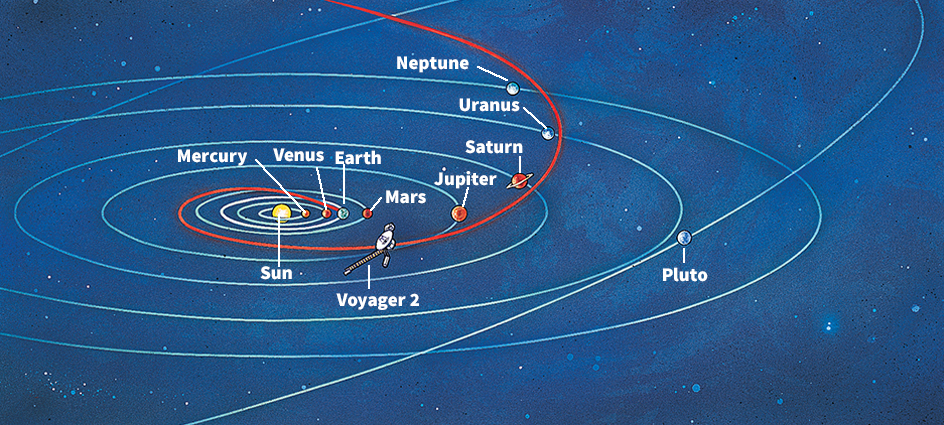
The Ulysses spacecraft, designed primarily to study the sun, passed within about 235,000 miles (378,000 kilometers) of Jupiter on Feb. 8, 1992. Its instruments measured Jupiter’s radio emissions as well as the plasma, dust, and other particles in the Jovian system. Ulysses gathered more data on Jupiter’s magnetosphere when it revisited the planet from late 2003 to early 2004.
In 1993, the American astronomers Carolyn and Eugene Shoemaker and the Canadian-born astronomer David H. Levy discovered a comet passing near Jupiter. Astronomers soon realized that the comet, called Shoemaker-Levy 9, had been captured by Jupiter’s gravity and broken into 21 or more fragments. Astronomers from around the world watched as most of the fragments collided with Jupiter over a period of several days in July 1994.
The Galileo spacecraft became the first craft to orbit Jupiter when it arrived at the planet on Dec. 7, 1995. The same day, a probe Galileo had released months earlier entered Jupiter’s atmosphere. The probe made the first precise measurements of the atmosphere’s helium, ammonia, and many other substances. It also recorded the speed of the winds below the cloud tops. The probe surprisingly detected few clouds and little water vapor, but astronomers think that the probe entered an area that was not typical of the rest of the atmosphere.
The main Galileo spacecraft continued to orbit Jupiter for eight years, making numerous important discoveries about the Jovian system. It detected massive thunderstorms in Jupiter’s atmosphere. It discovered that Ganymede has a magnetic field and found evidence that an ocean of water or soft ice lies beneath Europa’s surface. Galileo also studied volcanoes on Io, determining that their eruptions were powered by hot, magnesium -rich silicates from deep in Io’s interior.
The Cassini spacecraft, designed to study Saturn, flew by Jupiter at a distance of nearly 6 million miles (10 million kilometers) from the planet’s cloud tops in December 2000. The Galileo spacecraft was still in orbit around Jupiter at that time, allowing astronomers to conduct coordinated observations of the planet from two different locations. The data gathered were used to study Jupiter’s moons, its magnetosphere, and the weather systems in its atmosphere.
In 2007, the New Horizons spacecraft passed by Jupiter at a distance of 1.4 million miles (2.3 million kilometers) to gain speed on its voyage to Pluto and a distant region of the solar system called the Kuiper belt. New Horizons took images of Jupiter’s Little Red Spot, recorded a volcanic eruption on Io, and became the first craft to travel down the “tail” of Jupiter’s magnetosphere.
In 2009, an Australian amateur astronomer named Anthony Wesley noticed a large discoloration on the surface of Jupiter. After astronomers examined the “spot”, they determined it to be a remnant of an asteroid or comet impact, the first one observed since Shoemaker-Levy 9 in 1994.
In 2016, the Juno space probe entered into orbit around Jupiter. Juno carries instruments to study the planet’s atmosphere and internal structure and map its magnetic field. Scientists hope information gathered by the craft will provide insights into Jupiter’s formation.
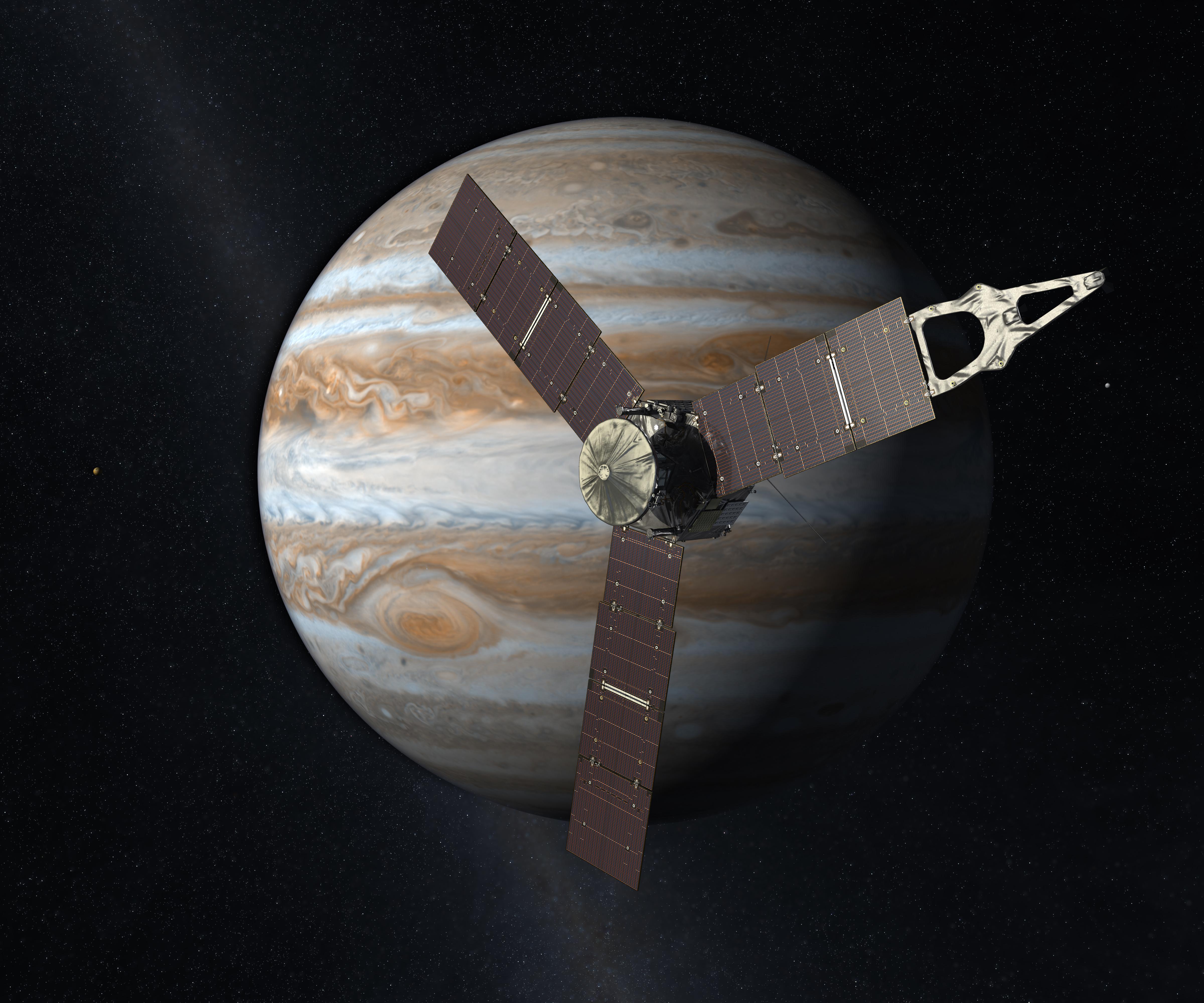