Aerodynamics, << `air` oh dy NAM ihks, >> is the study of forces that act on an object as it moves through air or some other fluid. Aerodynamic forces act on airplanes and all other objects that fly through the air. These forces also act on automobiles and other objects that move partly through the air and partly along a solid surface. In addition, aerodynamic forces act on ships, which move partly through the air and partly through water. Such forces even act on buildings due to the wind that blows around them. Scientists, engineers, and architects study aerodynamics to learn to design vehicles and structures.
Principles of aerodynamics
There are two basic aerodynamic forces—lift and drag. Both result from a transfer of force from a fluid to the surface of a solid object. The force from the fluid creates a pressure and a shear stress on the surface.
Pressure is force per unit area, with the force applied perpendicular to the surface. Pressure can be measured in pounds per square inch in the system of units customarily used in the United States. The pounds are a measure of the force, and the square inch is the unit of surface area. In the metric system, a common unit of pressure is the kilopascal. One kilopascal equals a force of 0.1 newton on an area of 1 square centimeter. One pound per square inch equals about 6.9 kilopascals.
Shear stress is also force per unit area, but this force is applied along the surface. Shear stress occurs in a fluid due to the fluid’s viscosity, its internal friction that resists motion. Friction occurs in a fluid whenever one layer of the fluid slides over another layer. Because of friction, the layers resist sliding.
The total aerodynamic force transferred from the fluid to the surface is a result of the pressure and the shear stress acting over the entire surface. Lift and drag are components (parts) of the total aerodynamic force. Lift is the component that is perpendicular to the direction of motion of the object. In the case of an airplane that is flying horizontally, the lift is applied in the upward direction. Drag is the component that pushes in the direction opposite that of the object’s motion. Thus, drag opposes the motion of the object.
Lift
keeps an airplane in the air by balancing the weight of the plane. This aerodynamic force is created along a wing by the motion of the wing through the air.
Lift can be analyzed in terms of the motion of an airfoil (cross section of a wing) through the air. The motion creates lift by producing a difference in air pressure. The air pressure on the lower surface of the airfoil becomes greater than the air pressure on the upper surface.
The pressure difference is a result of a difference in the speed of the air flowing along the two surfaces. According to a principle discovered by Daniel Bernoulli, a Swiss mathematician, the pressure of a fluid increases as the speed of the fluid decreases. The air pressure on the lower surface of the airfoil is greater because the air flows more slowly along that surface.
Lift can occur when the airflows along the top and bottom surfaces are unsymmetrical (unbalanced). Unsymmetrical flow is a result of one or both of the following factors: (1) the camber (curved shape) of the airfoil and (2) the angle of attack (angle at which the airflow meets the airfoil). A typical airfoil has a rounded leading (front) edge and a sharp trailing (rear) edge. As the air approaches the leading edge, it splits to go around the airfoil. The air that travels along the top accelerates as it goes around the highly curved leading edge. As a result, the speed of the air on the upper surface is greater.
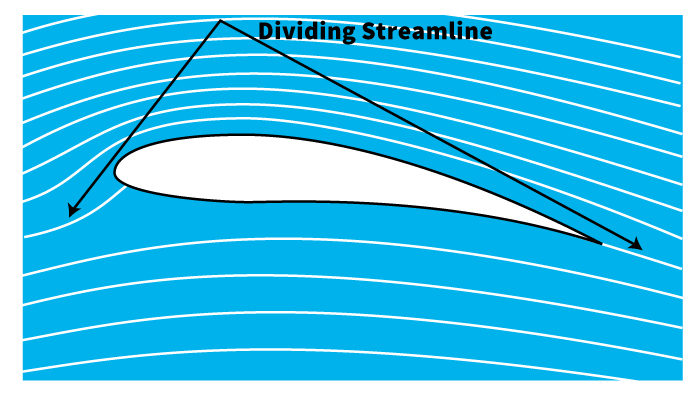
In addition, the airflows along the top and bottom of the airfoil merge smoothly as they leave the trailing edge. This condition is known as the Kutta condition—named for its discoverer, Martin W. Kutta, a German mathematician.
Another explanation for lift is related to an airfoil’s ability to deflect (turn) air downward. An airfoil deflects air by guiding the air along its cambered surface and by meeting the air at an angle. Deflection produces lift according to a law of motion explained in 1687 by the English scientist and mathematician Isaac Newton. This law states that, for every action, there is an equal and opposite reaction. Thus, as an airfoil deflects air downward, the reaction to the deflection produces an upward force by the air on the airfoil.
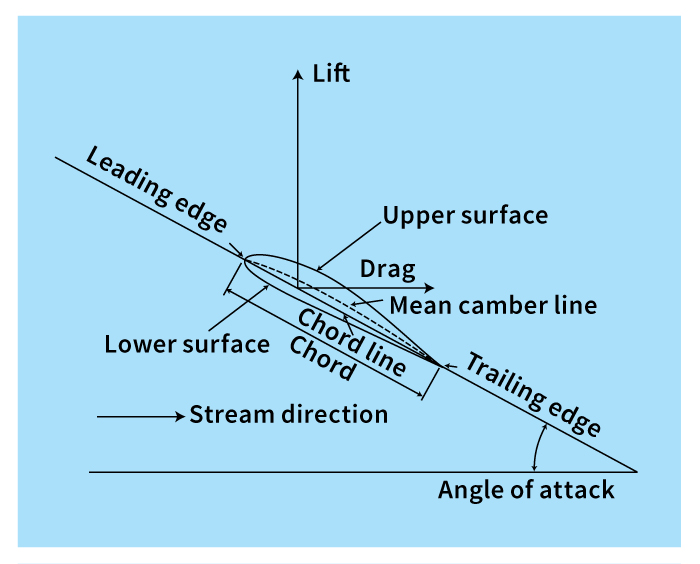
The amount of lift created by the airflow along a wing depends mainly on the wing’s angle of attack, speed, and camber. The area of the wing and the density of the air also affect the amount of lift.
Angle of attack
is the angle that a wing makes with the air flowing past it. A pilot can change this angle by changing the plane’s position in space. Increasing the angle of attack increases the lift—but only up to a point. If the angle of attack becomes too large, the airflow will separate from the upper surface of the wing. As a result, lift will decrease sharply, producing a condition called stall. An airplane at the point of stalling may crash unless the angle of attack is quickly reduced. Airplanes fly at angles of about 3 to 15 degrees. An airplane will stall if the angle becomes larger than 15 or 20 degrees.
Speed.
A wing’s speed through the air helps determine how much lift will occur. The faster the airplane is flying, the greater will be the lift.
Wing area.
An increase in wing area creates extra lift by increasing the total forces due to air pressure and shear stress. If the air pressure and the shear stress are held constant, the amount of force depends only on the area: The greater the area, the greater the force.
Air density.
Air that is relatively dense creates more lift than does relatively thin air. This happens because, according to Bernoulli’s principle, pressures increases with density.
Creating extra lift.
During take-offs and landings, pilots want to fly as slowly as possible. Special parts called high-lift devices enable a plane to fly at minimum speeds. These devices are extensions that fit smoothly against the wing while the airplane is cruising. The pilot can lower them when they are needed. The extension at the leading edge of the wing is called a slat. The extension at the trailing edge is a flap. When lowered, these extensions increase the wing camber and area, furnishing extra lift.
Drag
is a force that resists the forward motion of a solid object in a fluid. The object’s shape affects the amount of drag. Objects shaped to produce little drag are called streamlined or aerodynamically clean.
Two types of drag—friction drag and form drag–act on all moving objects. A third type, induced drag, affects only objects with lift. Still another kind of drag, wave drag, results when an object moves faster than the speed of sound. Wave drag also occurs when a ship generates waves on the surface of the water. This section discusses friction drag, form drag, and induced drag. For a discussion of wave drag, see the section Shock waves later in this article.
Friction drag
is a component of the drag due to shear stress. Friction has its strongest effect in the boundary layer, a thin layer of fluid next to the surface.
The amount of friction drag depends upon whether the fluid flow is laminar or turbulent. In laminar flow, the fluid molecules move in orderly paths essentially along the direction of the surface. Turbulent flow occurs at higher speeds. In turbulent flow, the fluid’s speed and direction of flow vary randomly from an average value. This variation increases the shear stress. As a result, friction drag is much higher when flow is turbulent than it is when flow is laminar.
Airflow is usually laminar near a wing’s leading edge, and it becomes turbulent farther along the surface. Airplane designers try to delay the change from laminar flow to turbulent flow. One way to do this is to make the surface as smooth as possible.
Form drag
is a component of the drag due to pressure. The amount of form drag on an object depends on the object’s form, or shape. If the object is not streamlined, the drag force is mostly form drag. If the object is streamlined, the drag force is mostly friction drag.
In form drag, the flowing fluid separates from the object. The pressure next to the rear surface of the object therefore decreases. This decrease makes the pressure on the front surface larger than that on the rear surface. The net result is a force that pushes against the front of the object. This force is the form drag. Designers can reduce form drag by streamlining the object.
Induced drag
is a result of a phenomenon that is also responsible for lift. Airflow will lift an airplane wing if the air pressure on the wing’s lower surface is greater than that on the upper surface. But this pressure difference also makes air flow at the tip of the wing. The air at the tip moves from the lower surface to the upper surface. This flow creates vortices, swirling streams of air that flow away from the tip and continue behind the wing.
The creation of wing-tip vortices uses energy that could otherwise be spent to provide lift and propel the aircraft. In addition, the vortices threaten the safety of airplanes flying close behind. Aircraft designers lessen induced drag by giving airplanes long, narrow wings.
Lift and drag in a sailboat.
The principles of aerodynamics also apply to objects that move through air but do not fly. One can use these principles to explain, for example, how a sailboat sails into the wind.
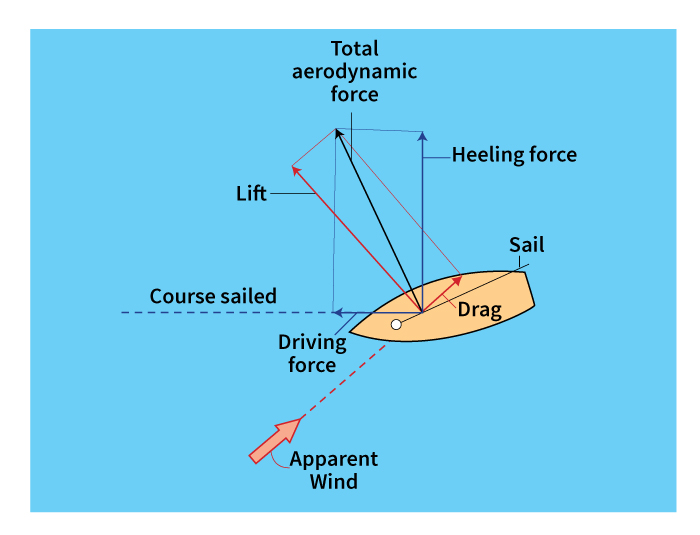
When the wind puffs the sail out, the sail resembles a cambered airplane wing. The apparent wind—the wind measured by an observer on the boat—thus generates a total aerodynamic force that corresponds to the force on a wing. This force can be resolved into (represented by) two components relative to the apparent wind: (1) lift, which tends to push the sail in a direction perpendicular to the apparent wind; and (2) drag, which resists the movement of the boat directly against the wind.
The total aerodynamic force can also be resolved into another pair of components: (1) a driving force in the sailing direction, and (2) a heeling force, which is perpendicular to the driving force. The driving force propels the boat. As the boat moves, the water exerts an aerodynamic force on its hull and keel. The keel is the main timber that extends the entire length of the bottom of the boat.
The boat will sail at constant speed when the aerodynamic force generated on the sail is both equal to and opposite the corresponding aerodynamic force that the water exerts on the hull and keel. This condition also has the effect of canceling the heeling force.
Supersonic aerodynamics
Effects of supersonic aerodynamics occur when the airplane flies at speeds greater than the speed of sound. Supersonic means faster than the speed of sound. Two of the major effects of supersonic aerodynamics are shock waves and sonic booms. Both are created by pressure disturbances that a moving airplane produces in the air.
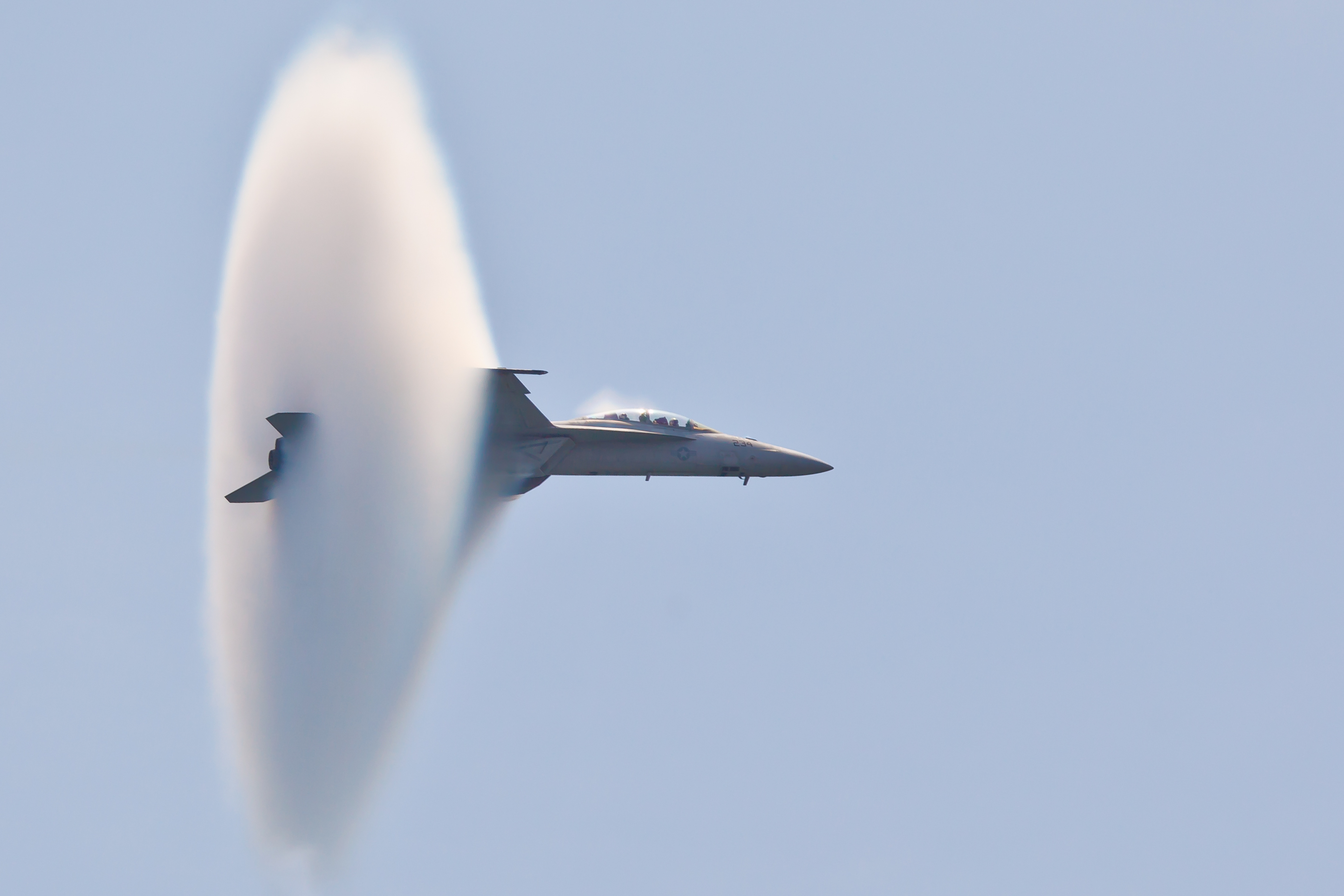
These disturbances result from the flow of air around the plane. The disturbances travel away from the plane just as ripples in a pond spread from the spot where a stone falls into the water. Pressure disturbances travel at the speed of sound—about 760 miles per hour (mph), or about 1,225 kilometers per hour (kph), at sea level.
Sound itself is a pressure disturbance, and so some of the disturbances produced by the airplane can be heard. If the plane is flying at less than the speed of sound, the sound of the plane travels ahead of the plane. Thus, people on the ground can hear the plane coming toward them. However, the sound of a plane flying faster than the speed of sound cannot be heard on the ground until the aircraft has passed.
Engineers and pilots use Mach numbers to describe the speed of planes flying near or above the speed of sound. A Mach number is found by dividing the speed of an airplane by the speed of sound at the plane’s altitude. For example, the Mach number of a plane flying at 1,520 mph at sea level would be 2. Modern airliners cruise at an altitude of about 35,000 feet (9,000 meters) and a speed of about Mach 0.80 to Mach 0.85.
Flight that is slightly faster or slower than Mach 1 is known as transonic flight. Flight that is significantly slower than Mach 1 is subsonic. Flight that is significantly faster than Mach 1 is supersonic, and flight at or faster than about Mach 5 is hypersonic. Mach numbers are named for Austrian physicist and psychologist Ernst Mach.
Shock waves
are pressure disturbances produced by the flight of an airplane at supersonic speed. Because disturbances cannot move ahead of the plane, they build up into shock waves. The waves then attach themselves to the front and rear of the plane. An airplane flying at slightly less than the speed of sound can also produce shock waves. These waves occur because the airflow next to some surfaces of the plane is actually supersonic.
Shock waves create wave drag, thereby increasing the total amount of drag on the plane. A plane designed for transonic and supersonic flight therefore has features that help reduce wave drag. For example, its nose is sharply pointed, and its wings have sharp, thin edges that can knife through the air. The wings may also be angled back from the body of the plane.
Sonic boom.
After a supersonic airplane flies overhead, people on the ground may hear a sharp “bang.” This sound, called a sonic boom, is caused by shock waves from the plane. A plane flying at supersonic speed sends out at least two shock waves—one from its front and the other from its rear. But the two waves may reach the ground so close together that people hear only one boom. A sonic boom may be strong enough to break windows or damage buildings.
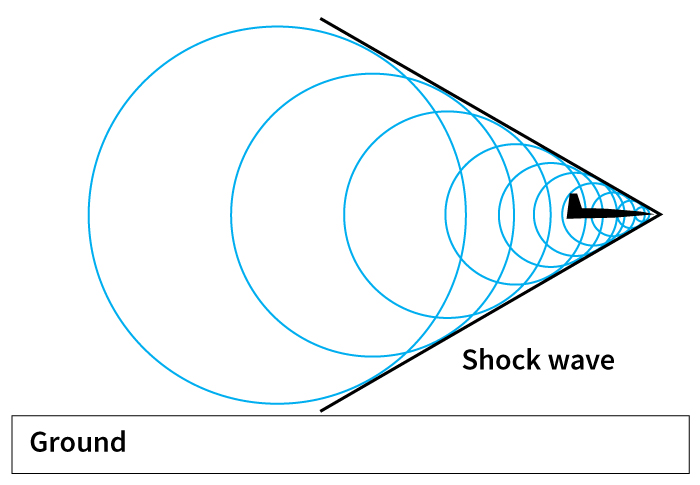
Applying aerodynamic principles
To design an airplane, engineers need to determine how the airflow will interact with the surfaces of the plane. They need to know in detail how strong the air pressure and shear stress will be at various places along the body, the wings, and other parts of the airplane. To gather the information they need, the engineers must study more than the layers of air next to the surfaces. They must investigate the entire flow field, the large region of space in which the airflow and the plane will interact. To study flow fields, aircraft design engineers almost always use a combination of two techniques—wind-tunnel testing and computational fluid dynamics.
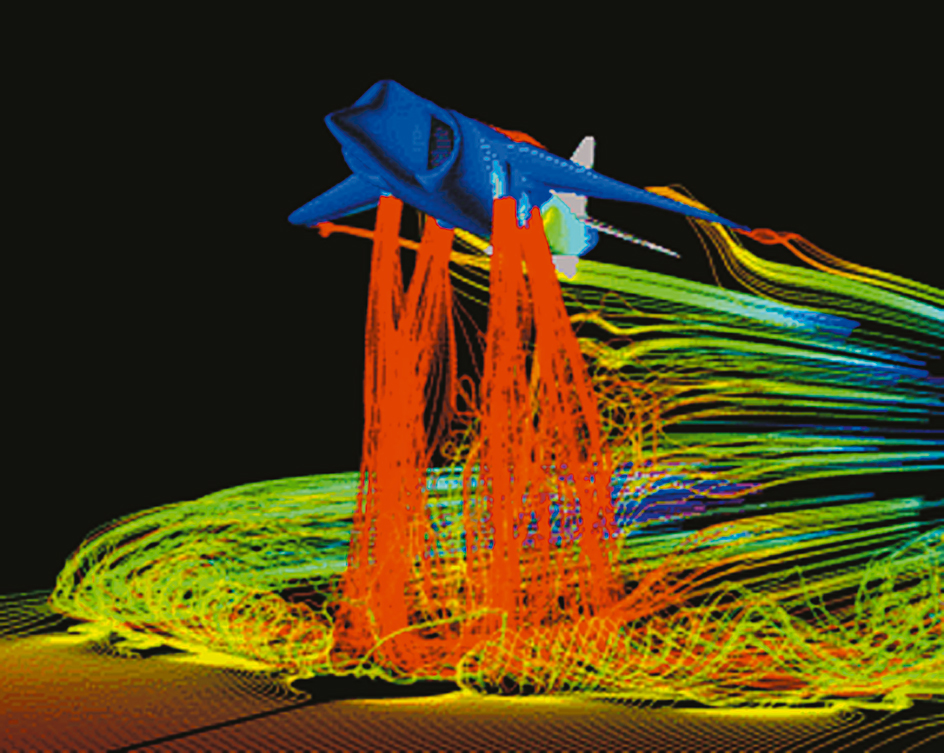
Wind-tunnel testing.
A wind tunnel is a ground-based facility in which a stream of air is blown at an object at uniform speed. Some wind tunnels are so huge that they can be used to test full-sized experimental airplanes. But most wind tunnels are relatively small facilities used to test scaled-down models.
Engineers can obtain much valuable information from wind-tunnel testing. However, the models are expensive to build. Furthermore, as the design evolves, the engineers often must test a large number of different airplane shapes.
Computational fluid dynamics
employs supercomputers, the fastest and largest computers. In this technique, a supercomputer solves equations describing physical laws that govern what happens at a large number of points in a flow field. The equations contain a huge number of factors that are related to one another in complex ways. Some factors have to do with flying conditions, including aircraft speed, wind speed, angle of attack, and air density.
The engineers first enter the equations into the supercomputer. Next, they enter the numerical values that apply to the plane they are designing and to the flying conditions. The computer then calculates the corresponding pressures, shear stresses, and other results. These calculations simulate (represent) how the airplane would interact with the air. In many cases, the computer creates a motion picture that shows a few moments of flight. The engineers evaluate the results and change the design as necessary.