Brain is the organ that serves as the body’s control center. The brain constantly receives information from the senses about conditions both inside and outside the body. It rapidly analyzes this information and sends out signals that control the body’s muscles and glands. The brain also stores information from past experiences, making learning and remembering possible. In addition, the brain is the organ of the mind. It is the source of all thoughts, moods, and emotions.
Human beings have the most highly developed brain of any living creature. The human brain enables people to use language, solve difficult problems, and create works of art.
The human brain appears as a grayish-pink, jellylike ball with many ridges and grooves on its surface. An adult brain weighs an average of about 3 pounds (1.4 kilograms). It includes about 100 billion nerve cells, called neurons. The neurons are linked by as many as 50 trillion connections called synapses << sih NAP seez >>. The neurons produce and transmit nerve impulses, electrical and chemical signals that are sent from cell to cell along distinct pathways.
Scientists in various fields work together to study the structure, function, and chemical composition of the brain. This study is called neuroscience or neurobiology. Neuroscience is rapidly increasing our understanding of the brain. But much remains to be learned.
The human brain develops in complexity over time. Most of the brain’s neurons are formed before birth. But many connections between neurons develop after birth. The human brain does not reach its full size until about 6 years of age. Some parts of the brain do not fully mature until after the teenage years.
Proper brain development depends on good nutrition and a stimulating environment early in life, when the brain is growing. Even in adulthood, however, the brain’s structure is not completely fixed. The brain is always forming new connections between neurons, enabling people to form new memories and learn new skills throughout life.
Although the brain never stops making new connections, its ability to form new connections declines with age. The brain also loses neurons over time. Neurons die as people age, and most of these neurons are not replaced. Once the brain has reached adult size, it begins to shrink gradually. This shrinking is part of the normal aging process. But in some diseases, such as Alzheimer’s disease, the brain shrinks faster than normal. In people with Alzheimer’s, the ability to form new memories declines sharply. Eventually, other vital brain functions are lost.
The brain requires vast quantities of oxygen and food, which are supplied by a network of blood vessels. The human brain makes up only about 2 percent of the body’s weight, but it accounts for about 20 percent of the oxygen used by the body at rest. The brain can go without oxygen for only three to five minutes before neurons begin to die, resulting in serious damage.
The brain’s neurons work together with the millions of other neurons that make up the rest of the nervous system. Some of these other neurons form the nerves that connect the brain to various parts of the body. Many nerves connect to the brain through the spinal cord. The spinal cord is a thick cable of neurons that extends from the base of the brain, through the neck, to about two-thirds of the way down the backbone. In addition, 12 pairs of nerves called cranial nerves connect the brain directly with certain parts of the body.
This article will discuss the parts of the brain, differences in individual brains, how the body protects the brain, how scientists study the brain, how the brain works, how neurons work, and the brains of other animals. For more information about the nervous system and the brain’s place in it, see Nervous system.
The parts of the brain
The human brain has three main divisions: (1) the cerebrum << SEHR uh bruhm or suh REE bruhm >>, (2) the cerebellum << sehr uh BEHL uhm >>, and (3) the brain stem. These structures consist of neurons and other specialized cells that support and care for them.
The cerebrum,
also called the forebrain, controls thought and many kinds of learning. It is the largest and most complex part of the brain. The cerebrum makes up about 85 percent of the human brain’s total volume. Some regions of the cerebrum are involved in analyzing complex sensory information. Other parts of the cerebrum control fine movements. Still other areas help regulate breathing, blood pressure, heartbeat, hunger, thirst, urination, and sexual urges.
A large groove divides the cerebrum into halves called the left cerebral hemisphere and the right cerebral hemisphere. A large bundle of nerve fibers connects the hemispheres.
Each hemisphere of the cerebrum is divided into four lobes. The lobes are (1) the frontal lobe, at the front of the brain; (2) the temporal lobe, at the brain’s lower side; (3) the parietal << puh RY uh tuhl >> lobe, in the middle; and (4) the occipital << ahk SIHP uh tuhl >> lobe, at the rear.
The outer portion of the cerebrum is called the cerebral cortex. The cerebral cortex is heavily folded, resulting in a surface with many ridges and grooves. The folds greatly increase the surface area of the cortex, enabling a large number of neurons to be held within the limited space of the skull. The neurons of the cortex are arranged in layers that vary in thickness. Because the neurons appear gray in color, the nerve tissue that makes up the cerebral cortex is often called gray matter.
Just beneath the gray matter, there are a large number of nerve fibers called axons << AK sahnz >>. The axons connect neurons in the different parts of the cortex with one another. They also connect neurons in the cortex with those in other parts of the brain. The axons have a coating of fatty material called myelin << MY uh lihn >>. Myelin insulates the axons and speeds the transmission of nerve impulses along them. Myelin is white, and tightly packed axons covered with myelin form the brain’s white matter. White matter makes up nearly half of the cerebrum.
Just beneath the cerebrum, at the upper end of the brain stem, are two structures called the thalamus and the striatum. The thalamus receives nerve impulses from other parts of the nervous system and sends them to appropriate areas of the cerebral cortex. The striatum receives nerve inputs from the cerebrum and transmits impulses back to it. The striatum also has some important connections to the brain stem.
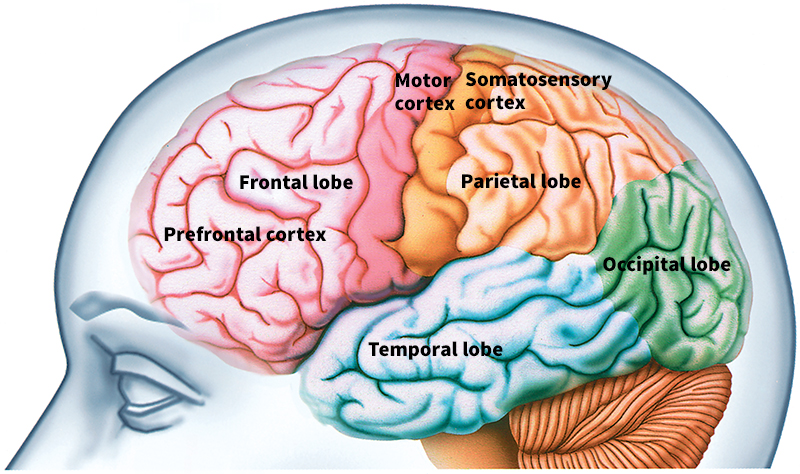
The cerebellum
is the part of the brain most responsible for balance, posture, and the coordination of movement. It makes up about 10 percent of the human brain’s total volume.
The cerebellum lies toward the back of the brain, below the occipital lobe of the cerebrum. Like the cerebrum, the cerebellum is heavily folded. It also has a right and a left hemisphere.
Scientists understand the workings of neurons in the cerebellum more thoroughly than those in any other major region of the brain. The cerebellum sends signals to the muscles to coordinate movements. When movements are not accurate, the cerebellum receives error signals, which it then uses to adjust motor commands.
People who suffer damage to the cerebellum have difficulty maintaining their balance and reaching for objects. Excessive drinking of alcohol can damage the cerebellum and several other regions of the brain.
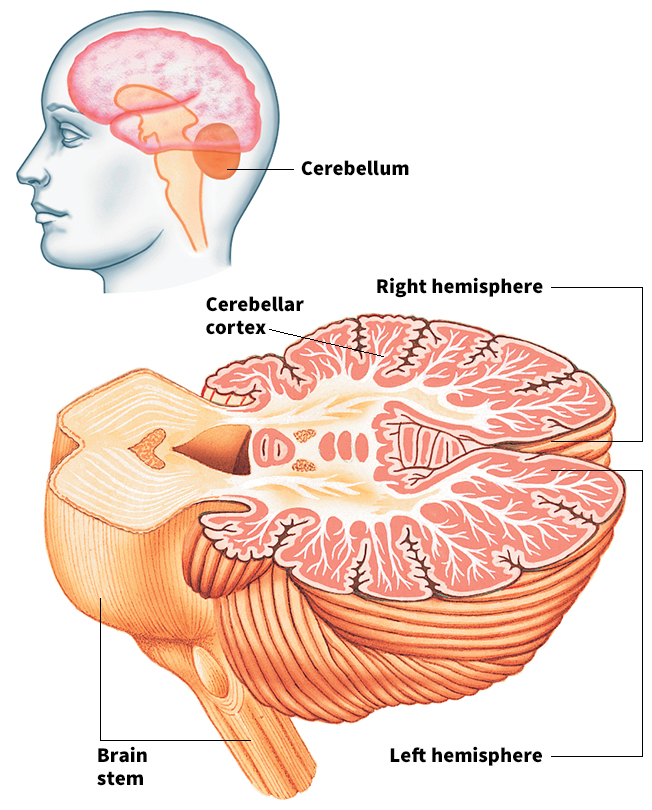
The brain stem
controls heart rhythm and breathing. It also passes signals between the cerebrum and cerebellum and between the rest of the brain and the body. For example, the brain stem receives information from all the senses except for smell. It sends this information on to the cerebrum through the thalamus. The brain stem likewise receives sensory inputs and signals from the cerebrum, which it passes on to the spinal cord and to muscles in the head.
The brain stem is a stalklike structure underneath the cerebellum. Deep within it lies a network of nerve fibers called the reticular formation. The reticular formation helps regulate and maintain arousal (readiness). Sensory messages that pass through the brain stem stimulate the reticular formation. The reticular formation in turn stimulates alertness and activity throughout the cerebral cortex.
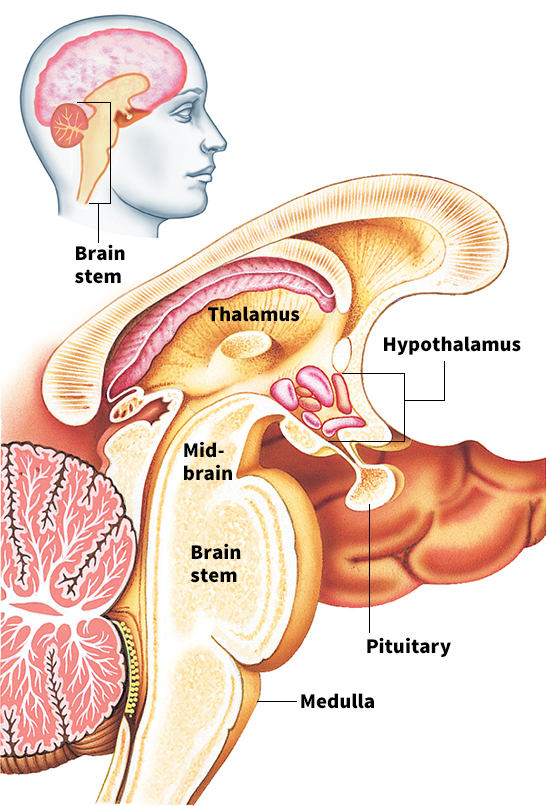
Brain cells.
The human brain has from 10 billion to 100 billion neurons. The neurons connect with one another in complex networks. Neurons are more complex than most other cells of the body. They also vary in size and shape. For more information on neurons, see the section How neurons work later in this article.
Neurons make up much of the brain. But they are outnumbered by another kind of cell, called glial << GLEE uhl or GLY uhl >> cells. There are several types. One type regulates the rate at which blood flows through various regions of the brain, helping to supply neurons with nutrients and energy. Another type of glial cell rids the brain of injured and diseased neurons by engulfing and digesting them. These cells increase in number when the brain is damaged by disease or injury. Still other glial cells produce the myelin sheaths that insulate some axons.
Glial cells continue to multiply into adulthood, replacing those that die over time. Neurons, in contrast, do not typically multiply. Most of a person’s neurons are formed before birth. In only a few regions of the brain do neurons continue to multiply into adulthood. Thousands of neurons die and are removed from the brain each day. However, scientists have found that neurons in one region of the brain associated with learning and memory are continually replaced.
Differences in individual brains
Individual brains can differ significantly in overall size and in organization. Some differences appear to be consistent between the sexes.
In overall size.
Human brains vary most noticeably in overall size. Although the average human brain weighs about 3 pounds (1.4 kilograms), it is not uncommon for a brain to weigh as little as 2.5 pounds (1.1 kilograms) or as much as 3.5 pounds (1.6 kilograms).
Most variation in brain size can be accounted for by variations in body size. People with larger bodies tend to have larger brains. Human females, for example, tend to have smaller bodies compared to males. Brains of adult human females weigh an average of about 0.22 pound (100 grams) less than brains of males. This size difference, however, does not produce a significant difference in intelligence between the sexes.
In organization.
The brain’s internal organization also differs between individuals. For example, in most people, the language circuits of the brain are mainly in the left cerebral hemisphere. In a small number of people, however, the language circuits are mainly in the right hemisphere. Among still other people, the two hemispheres participate almost equally in language.
Particular regions of the brain can vary in size, even among individuals of the same overall brain size. For example, scientists have observed that some areas of the cerebral cortex are larger in musicians than in other people. The cortex of a violinist, for instance, may be enlarged in regions associated with the sensation of touch from the fingers. In such cases, differences in individual experience shape differences in the brain’s organization.
Neuroscientists know that both experience and genes affect brain organization. But it is difficult to distinguish the role of genes from other factors. It is clear, however, that people can perform similarly on many tasks despite differences in brain organization. This fact suggests that brains organized in different ways may perform equally well.
Between the sexes.
In some animals, there are clear differences between the brains of males and females. Among some songbirds, for example, males sing, but females do not. The region of the brain associated with singing is much larger and more developed in the males.
Studies have revealed more subtle differences between the brains of human males and females. For example, studies show that some brain functions are less lateralized in females—that is, they are less strongly associated with a particular cerebral hemisphere. Some patterns of brain activity also differ between males and females, particularly in tasks not related to language. For example, an experiment showed that a part of the right hemisphere becomes more active in men who watch an exciting video. In women watching the same video, the corresponding part of the left hemisphere became more active.
Overall, neuroscientists have observed only a few clear differences in brain structure between human males and females. Researchers are not sure if these differences show that men and women think differently. Some evidence suggests that the sexes may have different mental strengths. Psychological testing consistently shows that men, on average, perform better than women on spatial tasks, such as visualizing objects in three dimensions. Women, on the other hand, do better than men on tests involving writing, reading, and vocabulary. But the average difference in both abilities is small. Many men do better at language tests than the average woman, and many women have better spatial skills than the average man.
How the body protects the brain
The brain ranks among the most vital parts of the body and the most sensitive to damage. For this reason, it needs protection both from injury and from harmful substances in the rest of the body.
From injury.
The hard, thick bones of the skull shield the brain from blows that might injure it. In addition, the brain and spinal cord are protected by membranes called meninges. The outermost membrane is the tough dura mater, which lines the inner surface of the skull. A thinner membrane, called the arachnoid mater, lies just beneath the dura mater. The delicate pia mater directly covers the brain, following the folds of the brain’s surface. The pia mater contains the vessels that carry blood to and from the cerebral cortex. A clear liquid called cerebrospinal fluid surrounds the entire surface of the brain and spinal cord, flowing between the meninges. Cerebrospinal fluid serves as a cushion between the soft tissues of the brain and the hard bones of the skull. It also removes wastes produced by brain cells.
A blow to the head can cause the brain to move violently within these cushioning layers, hitting the inside of the skull. Such a blow may cause an injury called a concussion. A concussion typically results in a temporary loss of consciousness, followed by a brief memory loss. If the injury is severe, more extensive memory loss or permanent brain damage can occur.
Although the meninges protect the brain from blows, they can become infected. Infections of the meninges, called meningitis, often cause serious illness. Symptoms of such illness include headaches, nausea, and sensitivity to light. Physicians can treat the illness with antibiotics or antiviral drugs, but the infections may be fatal.
Some parts of the brain are more vulnerable to damage than others. Damage to the cerebral cortex generally causes fewer serious problems than similar damage to the brain stem. In the cerebral cortex, undamaged neurons can often take over the functions of the damaged cells. Neurons in the brain stem, in contrast, are not so easily replaced.
Because the brain stem controls many of the basic functions necessary for life, most injuries to the brain stem are serious. A stroke or injury that damages the brain stem, for example, can cause a rare neurological disorder called locked-in syndrome. Locked-in syndrome is characterized by the complete paralysis of voluntary muscles, except in some cases for the muscles that control eye movement. More serious brain stem injuries usually prove fatal.
From harmful substances.
Nutrients and other substances in the blood reach body tissues through tiny blood vessels called capillaries. The cells in the wall of a capillary have spaces between them, making it easy for large molecules to pass through. In capillaries that service the brain, however, the cells of the wall are packed more tightly. The packing restricts the passage of certain substances from the blood to the brain cells, a protective effect referred to as the blood-brain barrier. The blood-brain barrier keeps most large molecules from entering the brain. Such large molecules include most of the substances that are poisonous to neurons.
Despite the protection offered by the blood-brain barrier, the brain needs some kinds of large molecules for nutrition. Special molecules must actively pump these nutrients into the brain.
The blood-brain barrier also helps keep out harmful bacteria. As a result, the brain rarely gets infected. Some viruses, however, are small enough to pass through the barrier and infect the brain. When this occurs, the blood-brain barrier can actually hinder the body’s ability to fight the disease. It does this by blocking the passage of cells from the body’s immune system.
Scientific study of the brain
Neuroscientists have learned much about the brain’s structure, function, and organization through laboratory studies of animals, such as rats and monkeys. Importantly, such studies have shown that rodent and monkey brains are similar to human brains in many ways. Therefore, much of the knowledge from animal studies can be applied to human brains. However, the studies also show that human brains are unique in some important ways. For example, the brain regions involved in making complex decisions are much larger in humans than in other animals. Human brains were traditionally studied only after removal from the bodies of dead people. Today, scientists can study the brain using imaging technology, experiments with wires called electrodes, and genetic techniques.
Imaging.
Modern medical technology can produce detailed images of the living human brain. For example, a special kind of X ray called a computed tomography (CT) scan can reveal brain tumors or bleeding in the brain. Two other scanning techniques also enable scientists to study healthy, living brains at work. They are positron emission tomography (PET) and functional magnetic resonance imaging (fMRI). Both techniques produce images similar to X rays. These images show which parts of the brain are active while a person does a particular mental or physical task.
Electrode experiments.
Neuroscientists can also study the brain by placing electrodes on the scalp. These wires pick up electrical signals produced by neurons in the cerebral cortex. The signals can be recorded to produce an electroencephalogram (EEG).
Occasionally, physicians may insert fine electrodes directly into a person’s brain in preparation for brain surgery. The insertion can be done while the patient is awake because the brain tissue cannot feel pain directly. The electrodes can then be used to record the activity of neurons while the patient performs a task. They can also be used to stimulate parts of the brain while the patient reports any feelings experienced. Such experiments help scientists learn about human brain function.
Genetics.
Genes carry instructions for the development of the entire body, including the brain. These instructions are complex, and slight changes in the genes, called mutations, can produce drastic changes in the body. Genetic mutations can lead to serious defects in the structure and functioning of the brain. But scientists have also learned about the brain from studying such mutations. For example, scientists have discovered genetic mutations in mice that produce a condition identical to Alzheimer’s disease. Scientists can use this knowledge to understand how such diseases occur in human beings, enabling the development of new treatments.
How the brain works
Scientific studies have revealed much about the workings of the human brain. These studies provide insight into how the brain: (1) senses the environment, (2) controls movement, (3) regulates bodily processes, (4) remembers and imagines events, (5) regulates emotions, (6) controls attention and consciousness, (7) makes decisions, and (8) produces language.
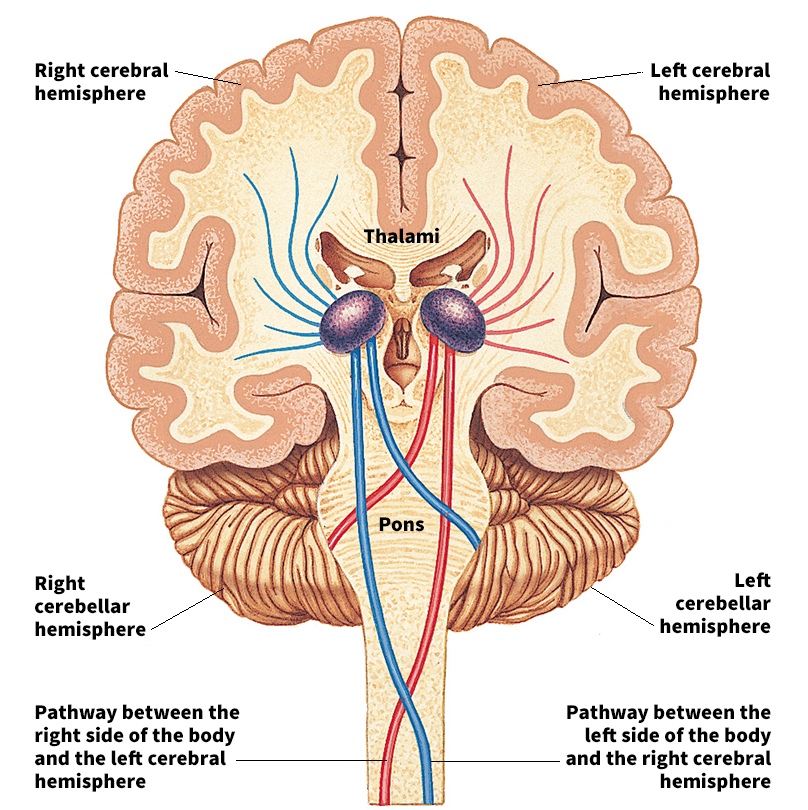
Sensing the environment.
Various parts of the body send sensory messages to the brain in the form of nerve impulses. These messages are received and interpreted primarily in the cerebral cortex. For example, the back of the eye contains cells that detect light. These cells stimulate nerve fibers that join at the back of the eye to form the optic nerve. The optic nerve carries signals from the light-sensing cells to certain regions of the cerebral cortex. The cortex interprets the signals as visual images.
Cells in other parts of the body specialize in detecting pain, smell, sound, taste, temperature, and touch. Some sense blood pressure and blood chemistry. Others detect the stretching and tension of muscles. All of these sensory cells send nerve impulses along nerves to the brain or spinal cord. Through the nerves, the brain receives an enormous amount of information about environment inside and outside the body.
Within the brain, sensory information flows from neron to neuron along multiple pathways. Some of these pathways go to the brain stem. There, sensory information is used to adjust basic functions, such as heart rate, breathing, and posture. Other neuron pathways go to the cerebellum, which helps to fine-tune adjustments made by the brain stem. Many pathways carry sensory information to the cerebrum, where it is used to control more complex behaviors.
Messages related to bodily sensations, such as touch and temperature, are received and interpreted in an area of the cerebrum called the somatosensory cortex. The somatosensory cortex lies near the front of the parietal lobe in each hemisphere. Different areas of the cortex process information from different parts of the body, with information from neighboring body parts usually processed in neighboring areas of the cortex. Certain parts of the body have more sensory cells than other areas. More neurons are required to process information from these sensitive parts. For example, about half of the neurons in the somatosensory cortex process sensory impulses from the hands and face. These sensitive areas have the highest density of touch sensors.
The same sensory information can follow multiple neuron pathways within the cerebrum, each dedicated to a different purpose. Neuroscientists learned this fact by studying patients who suffered brain damage. Upon looking at a photograph of an object, for example, some brain-damaged patients can tell a person where the object is, but they cannot tell the person what the object is. This fact suggests that the two tasks involve different neuron pathways.
Even though various types of sensory information are processed in different parts of the cerebrum, we experience the world as a unified whole. Neuroscientists have wondered how this is possible. Most of them think that connections between neurons in different areas of the cerebrum coordinate patterns of nerve impulses in the brain. The connections enable the cerebrum to combine the impulses into a unified perception of the environment. Support for this view comes from studies of patients who have had their corpus callosum << KOHR puhs kuh LOH suhm >> cut for medical reasons. The corpus callosum is a large bundle of nerve fibers that connects the cerebral hemispheres. Patients who have had theirs cut sometimes act as if they had two separate minds. For example, a patient’s hands—each of which is controlled by a different hemisphere—might struggle against each other.
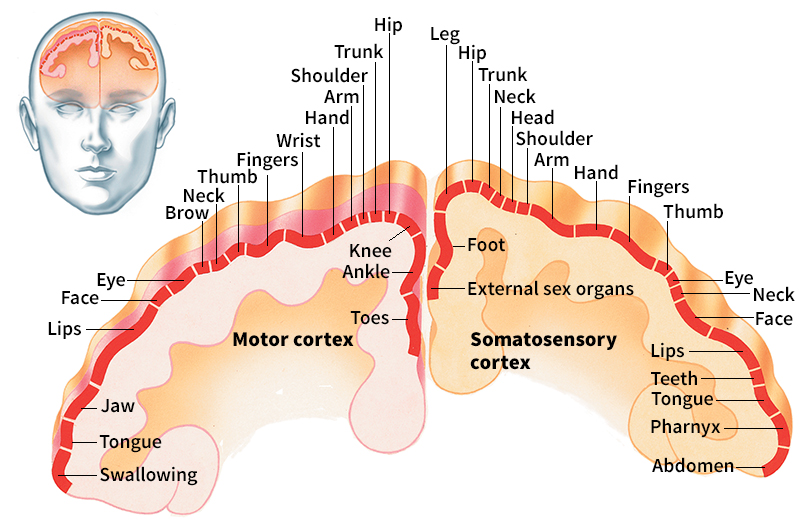
Controlling movement.
Some reflex actions do not involve the brain. If a person touches a hot stove, for example, pain impulses flash to the spinal cord. The spinal cord immediately sends back a message to withdraw the hand. However, the brain also receives information about such pain and plays a major role in controlling our conscious movements.
Certain neurons in the brain stem and spinal cord send command signals to muscles. These neurons are called motor neurons. Motor neurons receive commands from multiple sources in the brain. One source is a region of the cerebral cortex called the motor cortex. The motor cortex lies at the rear of the frontal lobe, just in front of the somatosensory cortex. The motor cortex controls mainly the muscles of the arms, legs, mouth, and face. Damage to the motor cortex can interfere with the ability to handle objects or talk. However, some lost abilities may return as other brain regions take over for damaged areas.
Neurons in the motor cortex work with brain stem neurons to control some involuntary movements, such as quick eye or head movements. They also help control the muscles of the hips and back, which play a role in maintaining posture and balance. The motions controlled by the cerebral cortex are tightly coordinated with those controlled by the brain stem.
When the neuron pathways from the brain to the spinal cord are damaged, most voluntary movements of the body cease. Movements of the eyes and face, however, are generally not affected by spinal cord damage. Their motor neurons originate in the brain stem rather than the spinal cord. Some simple reflexes also remain.
Regulating body processes.
The brain controls not only visible movements but also movements deep inside the body. Such movements include the beating of the heart, the expansion or tightening of blood vessels, and the contraction of muscles in the intestines. In addition, the brain controls the secretion of hormones, tears, sweat, saliva, and digestive juices. All of these functions are vital for survival and good health, but they all happen without much awareness or voluntary control.
Many basic body processes are coordinated by a small structure called the hypothalamus. The hypothalamus is one of the smallest regions within the cerebrum, but it helps regulate many of the most vital body functions. The hypothalamus helps control the autonomic nervous system, which regulates such automatic functions as breathing, blood pressure, heartbeat, hunger, thirst, urination, and sexual urges. The hypothalamus also controls the pituitary gland, the so-called “master gland” of the endocrine (hormone-producing) system.
The hypothalamus helps maintain a stable internal environment by receiving information about the condition of the body and sending out commands. For example, certain neurons detect changes in the level of water in the body’s tissues. They relay this information to the hypothalamus. The hypothalamus helps create the sensation of thirst and causes the person to look for something to drink. Other neurons in the hypothalamus sense when the blood is running low on nutrients. These neurons send out commands that promote eating and the search for food. Still other neurons sense when the stomach is full and signal when it is time to stop eating.
The brain responds to the body’s changing needs during increased activity and in emergencies. At these times, it can send signals to speed up the heartbeat, increase blood pressure, send additional blood to the muscles, and enlarge the pupils of the eyes to take in more light. This fight-or-flight response prepares an individual to either attack or run away. After the need has passed, other brain regions instruct heart rate, breathing, and blood flow to return to normal.
Memory and imagination.
Neuroscientists once thought that different brain regions controlled memory, imagination, and experience. But they now understand that these processes involve overlapping brain regions.
One brain structure important to the formation of memories is the hippocampus, part of the cerebrum. Many neuroscientists believe that memory formation involves the neuron pathways from various parts of the cerebral cortex that connect to the hippocampus. Scientists think the pathways create small networks of neurons called cell assemblies, which store memories.
When a new memory is triggered, a cell assembly in the hippocampus is activated along with corresponding neurons elsewhere in the cerebral cortex. When a memory has been triggered repeatedly, the activated neurons in the cerebral cortex gradually become linked to one another, changing the original cell assembly. Eventually, the memory will become independent of the hippocampus and will be stored primarily in other areas of the cerebral cortex. When part of a cell assembly is stimulated, it can cause the entire assembly to become active. Thus, a sensory signal, such as a familiar smell, can trigger a whole related memory.
People who suffer damage to the hippocampus develop anterograde amnesia, the inability to form new long-term memories. But these people retain at least some old memories. People with Alzheimer’s disease—which destroys the neurons of the hippocampus and the cerebral cortex—tend to lose their old memories. This type of loss is called retrograde amnesia. Interestingly, the oldest memories are usually the last ones to be lost.
Neuroscientists have found that remembering an event activates many of the same regions of the cerebral cortex that were active when the event occurred. In addition, simply imagining an action or event also activates many of the same brain regions that would be active if it actually happened.
Even dreaming can activate most of the same brain regions that are activated by real waking experience. When the dream includes body movements, however, the neuron pathways that send commands to the muscles are blocked. This prevents people from harming themselves by moving while dreaming.
Regulating emotions.
Strong emotions involve both physical responses and our understanding of what caused them. For example, a person’s heartbeat may increase in response to either joy or fear. Memories of recent events help the brain determine which emotion is being experienced.
Fear is a strong emotion that causes most animals to run, attack, or hold completely still. The body’s reaction to fear is largely controlled by a part of the brain called the amygdala << uh MIHG duh luh >>. The amygdala sits deep within the temporal lobe. When a rat smells a cat, for example, the smell activates a pathway from the nose to the amygdala, and from there to the hypothalamus. The hypothalamus triggers the fight-or-flight response. Many fears are learned through bad experiences. Learned fears involve not only the amygdala but also brain regions such as the hippocampus.
The emotion of happiness includes the release of certain chemicals into the blood. These chemicals, called endorphins, are carried to the brain. Endorphins are chemically similar to opioid drugs, such as morphine or heroin. Neuroscientists do not know exactly how endorphins work. But scientists have observed that a small region of the midbrain called the ventral tegmental area, along with a small region of the forebrain called the nucleus accumbens are sensitive to opioids. In laboratory experiments with animals, the direct application of opioids to these areas causes the animals to act happy. Natural happiness also activates the same regions.
In addition to fear and happiness, people may feel anger, surprise, disgust, or more complex emotions. People can also suppress emotions. The ability to control emotional behavior is regulated by neuron pathways that originate in the prefrontal cortex, a part of the frontal lobe. These pathways connect to the amygdala. When the associated part of the prefrontal cortex is damaged, patients become more likely to have emotional outbursts. They may also become depressed because they cannot control their unpleasant feelings.
Attention and consciousness.
The concepts of attention, awareness, and consciousness are difficult to define. This fact makes it difficult to study the brain mechanisms behind these conditions. However, neuroscientists have observed certain changes in brain activity that correlate with falling asleep, dreaming, waking up, or becoming unusually alert. Many of these changes in activity are caused by groups of cells in the brain stem. One region of the brain stem important to attention and consciousness is the locus ceruleus. The neurons in this location are relatively inactive during deep sleep and active while a person is awake. When something unexpected grabs your attention, neurons in the locus ceruleus generate a sudden burst of signals. This burst of activity helps the brain’s sensory pathways to process information more efficiently.
When people focus their attention on a specific location in space, they often move their head and eyes so that they are looking directly at the location. People also sometimes direct their attention to a particular region of space without moving their head or eyes, called covert attention. Neuroscientists have found that covert attention involves some of the same neuron pathways as head and eye movement.
Neuroscientists have learned much about the mechanisms of attention from studies of certain patients. These patients suffer damage to the lower part of the right parietal lobe. Such patients often exhibit hemispatial neglect—that is, they tend to ignore whatever happens to be on their left side. Damage to the left side of the brain is sometimes associated with neglect of the right side of the body. This, however, is less common.
People often direct their attention to particular objects unconsciously. For example, when you take a walk down a familiar path, your brain pays attention to obstacles even though your conscious mind may be preoccupied with different thoughts. Indeed, only a small fraction of what goes on in the brain ever reaches consciousness. Neuroscientists know almost nothing about what causes some brain processes to erupt into consciousness while other processes remain hidden.
One of the most the most remarkable aspects of consciousness is the ability to be aware of oneself. Such self-awareness develops during the first two or three years of life. There is evidence that some apes also have self-awareness. Chimpanzees, for example, can recognize themselves in a mirror. But monkeys cannot. Other intelligent animals, including dolphins and elephants, show some self-awareness. However, the brain mechanisms behind self-awareness remain almost entirely unknown because they are almost impossible to study in animals.
Making decisions.
People make many different kinds of decisions, both conscious and unconscious. One simple form of decision involves choosing between opposite courses of action. When faced with an unfamiliar object, for example, a person might decide to approach it or move away from it. Within the brain, this kind of decision is usually handled by having the pathway involved in one action inhibit (block) the pathway for the other action, or vice versa. This kind of reciprocal inhibition keeps conflicting pathways from becoming active at the same time.
A region of the brain called the striatum << stry AY tuhm >> appears to play a major role in decision making. The striatum is located deep within the cerebrum. It receives impulses from many different regions of the brain. It sends impulses to areas in the brain stem and cerebrum that control movements. The striatum can use information from the rest of the brain to influence which movements should take place. It normally inhibits activity in its target regions, but it can temporarily cancel the inhibition. When the inhibition is removed, neurons in the motor cortex or brain stem send out motor commands. Thus, the striatum effectively decides which movements are allowed and which remain inhibited.
The striatum normally helps select the most appropriate motor commands for action. But research shows what happens when the striatum fails to work properly. For example, in people with Parkinson disease, damage to a nearby part of the brain causes the striatum to inhibit the motor control regions too much. Motor movements are suppressed, and the patient finds it difficult to initiate any new movements. In contrast, people with Huntington’s disease have a weakened striatum that cannot inhibit the motor control regions effectively. These patients tend to perform inappropriate movements uncontrollably.
Neuroscientists believe that some neurons in the striatum are modified during learning. Most scientists agree that damage to the striatum interferes with the ability to learn new habits or skills. This kind of learning may seem to have little to do with making decisions. But it can be seen as the brain learning to decide what actions are appropriate.
More complicated decisions involve the prefrontal cortex. The prefrontal cortex is significantly larger in human beings than in chimpanzees and other intelligent animals. In the 1940’s and 1950’s, thousands of patients had their prefrontal cortex surgically cut as a therapeutic procedure for certain mental illnesses. This procedure, called a prefrontal lobotomy, may have helped a few patients, but it seriously damaged the mind of many other patients. In particular, it destroyed the patients’ ability to make sound decisions and keep to long-term plans.
Language
requires an enormous number of special abilities, all of which are controlled by the brain. Human speech requires the ability to perform intricate movements of the lips, tongue, and vocal cords. It also requires the ability to link words to the concepts or objects that they represent. In addition, people must be able to understand what others are saying.
Human language involves several different regions of the brain. In the late 1800’s, scientists observed that damage to particular parts of the brain caused the same language disabilities in most patients. Damage to the left frontal lobe, in Broca’s area, destroyed the ability to produce fluent speech. Damage to the upper back region of the temporal lobe, in Wernicke’s area, caused difficulty understanding language. These areas are named for the French surgeon Pierre Paul Broca and the German neurologist Carl Wernicke. Broca’s and Wernicke’s areas play crucial roles in human language. But scientists have also found both areas in monkeys. Scientists do not fully understand the function of these areas in monkeys, but the regions seem to be activated by the vocalizations of other monkeys.
Neuroscientists have discovered other areas of the cerebral cortex that also contribute to language. These areas were discovered in experiments in which surgeons electrically stimulated different brain regions. Stimulating certain parts of the cerebral cortex disrupted speech in the patients. Brain imaging studies have shown that speaking or listening activates different areas of the cerebral cortex and some regions outside the cortex.
For most people, the left cerebral hemisphere is more important for controlling language than the right hemisphere. However, all the areas that control language in the left hemisphere also exist in the right hemisphere. Neuroscientists have observed that patients with damage in the language areas of the right hemisphere often sound robotlike and that their speech lacks the rhythm of normal human speech. This fact suggests that although the hemispheres are specialized for different language functions, they work together to produce speech.
How neurons work
All the brain’s functions rely on the transmission of nerve impulses by neurons. A neuron consists of a round cell body with slender extensions called dendrites branching out from it. The dendrites grow narrower the farther they extend from the cell body. Most neurons also have a thin, tubelike axon. The axon may have a myelin coating or no coating at all. The axon extends far beyond the cell body. There, it branches and comes into contact with the dendrites of other neurons, forming synapses. A single neuron may form synapses with thousands of other nerve cells.
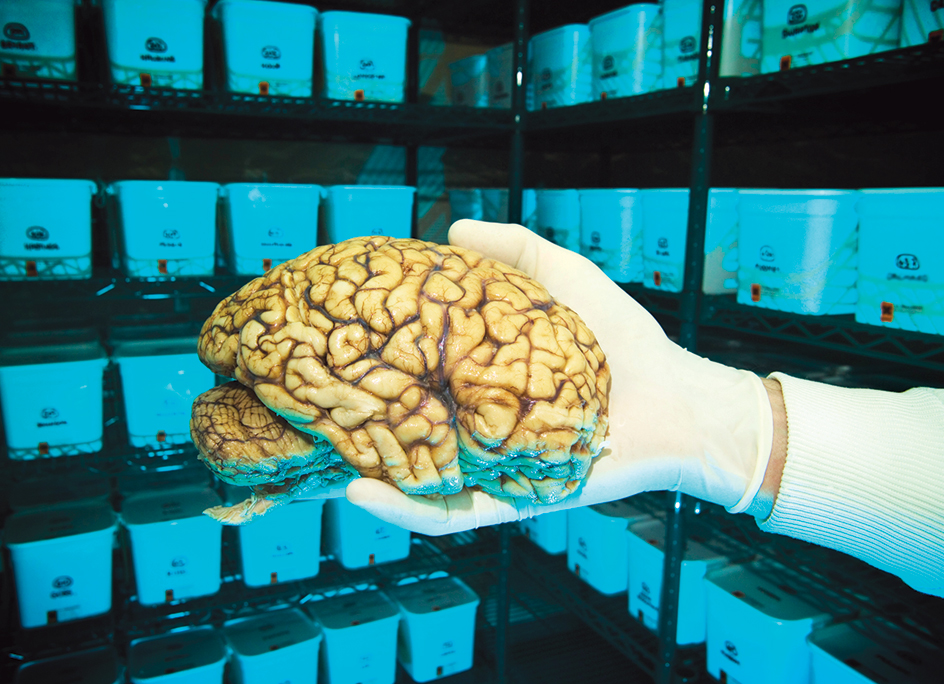
Action potentials.
Neurons differ from other cells in their ability to transmit nerve impulses. A nerve impulse travels along a neuron as an electrical signal called an action potential.
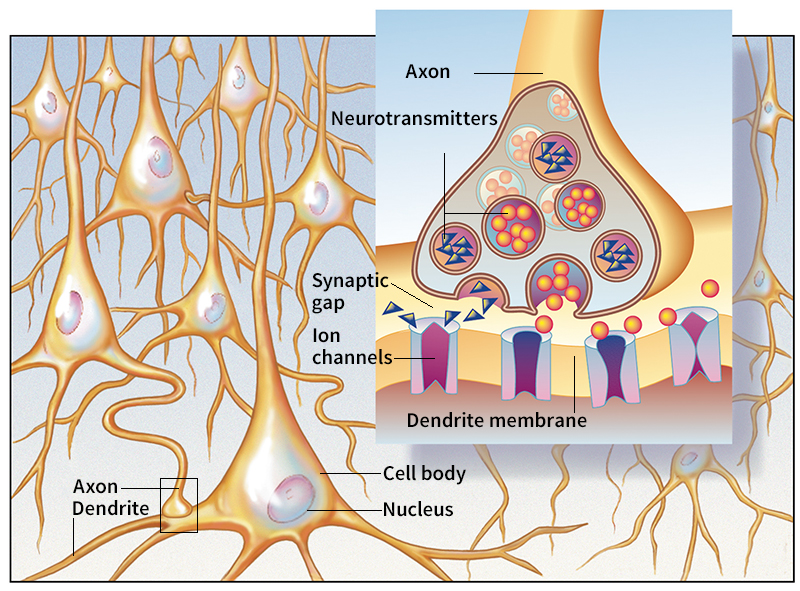
Neurons generate action potentials by controlling the movement of ions (electrically charged particles) across their cell membranes. The ions move in and out of the neuron through pores called ion channels. When the neuron is at rest, the membrane maintains a low concentration of positively charged ions inside the neuron. When stimulated, the ion channels allow more positive ions to enter the neuron, which becomes electrically excited. Once the excitement reaches a certain level, more ion channels open and an action potential is generated. The action potential travels down the axon to a synapse.
Synapses.
The action potential cannot be transmitted electrically across the synaptic gap, the tiny space between the axon of one neuron and the dendrite of another. Instead, chemicals called neurotransmitters carry the impulse between neurons. When the action potential reaches the end of the axon, it triggers the release of neurotransmitter molecules from the cell. The molecules cross the synaptic gap to the dendrite of the next neuron. There, they attach themselves to receptor molecules on the dendrite, causing ion channels to open. If enough channels open, the second neuron will become electrically excited, generating its own action potential. In this manner, nerve impulses are transmitted from neuron to neuron. For more details about this process, see Nervous system (How neurons carry impulses).
Synapses vary in how efficiently they transmit impulses between neurons. Synapses that are more active tend to become stronger and transmit more reliably. Synapses that are inactive become weaker. Neuroscientists have also shown that when two neurons fire electrical impulses at the same time, the synapses that connect those neurons become stronger. This effect is called Hebbian synaptic plasticity. It is named for the Canadian neuroscientist Donald Hebb, who first described it in 1949. Neuroscientists believe Hebbian synaptic plasticity underlies a variety of complex processes in the brain, including the formation of memories.
Neurotransmitters.
The human brain produces many kinds of chemicals that are used as neurotransmitters. The most common ones include acetylcholine, dopamine, norepinephrine, and serotonin. Neurotransmitters are not distributed evenly throughout the brain. Many are found exclusively or primarily in specific areas. For example, the bodies of neurons that contain dopamine are in the brain stem. The axons of these cells reach into other areas, including the frontal lobes of the cerebrum. This arrangement forms dopamine pathways that function in the regulation of emotions and in the control of complex movements.
Disorders of the brain
Injuries, diseases, and inherited disorders can damage the brain. However, the seriousness of brain damage depends chiefly on the area of the brain involved rather than on the cause of the damage. Disorders that destroy brain cells are especially serious because the body cannot replace the lost cells. In some cases, however, undamaged areas of the brain may eventually take over some functions formerly carried out by the damaged areas.
Modern instruments and techniques enable physicians to diagnose brain disorders earlier and more accurately than in the past. For example, an instrument called an electroencephalograph (EEG) measures the patterns of electrical activity produced by the brain. Differences from normal EEG patterns may indicate damage to the brain and help locate the area of the damage.
Another important technique is computed tomography (CT). It involves X-raying the brain in detail from many angles. A computer then analyzes the X-ray data and constructs a cross-sectional image of the brain on a TV screen. CT scans are especially useful for identifying cases of bleeding within the skull, which may cause headaches, nausea, and brain damage. Magnetic resonance imaging (MRI) uses magnetic fields and radio waves to produce three-dimensional images of the brain’s internal structure. The images produced by MRI are more detailed than those obtained from CT scans. They are most often used to check for brain tumors.
Injuries
are a leading cause of brain damage among people under 50 years of age. A blow to the head may cause temporary unconsciousness. People may recover from such blows (also known as concussions) without permanent damage. But a medical doctor should be consulted, especially if the injured person becomes nauseated or remains confused for more than one minute after the blow. Neuroscientists now understand that repeated blows to the head, as occur in some sports, may cause long-term brain damage and depression.
Stroke
is the most common serious disorder of the brain. A stroke occurs when the blood supply to part of the brain is cut off. Nerve cells in the affected areas die, and the victim may lose the ability to carry out functions controlled by those areas. Many stroke victims suffer paralysis on one side of the body. Other symptoms include difficulty in speaking or in understanding language. Most strokes result from damage to the blood vessels caused by hypertension (high blood pressure) or arteriosclerosis (hardening of the arteries). Some victims of massive strokes die, but many other stroke victims survive and recover at least partially.
Tumors
are abnormal growths that can cause severe brain damage. The effects of a tumor depend on its size and location. A tumor may destroy brain cells in the area surrounding it. As the tumor grows, it also creates pressure, which may damage other areas of the brain or interfere with their normal function. Symptoms of a tumor include headache, seizures, unusual sleepiness, a change in personality, or disturbances in sense perception or speech.
Surgery cures some tumors. For cancerous tumors, physicians may combine surgery with drugs or radiation. One type of radiation, called stereotactic radiosurgery, is sometimes used as an alternative to traditional surgery. In stereotactic radiosurgery, doctors use computers and a CT scan or MRI to produce a three-dimensional image of the brain. Beams of radiation are then focused precisely on the target, which may be a tumor or a blood-vessel malformation. The individual beams are either too brief or too weak to harm areas of the brain in the path of the radiation. But their combined effect will destroy the target. These procedures are quick and painless and allow patients to resume moderate activity the same day.
Infectious diseases.
A number of diseases caused by bacteria or viruses can damage the brain. The most common of these infectious diseases are encephalitis and meningitis, either of which may be caused by bacteria or viruses. Encephalitis is an inflammation of the brain. Meningitis is an inflammation of the meninges, the membranes that cover the brain and spinal cord. A virus disease called poliomyelitis attacks the brain and spinal cord. Vaccines to prevent polio were developed in the 1950’s.
Genetic disorders.
Our genes carry instructions for the development of our entire bodies, including the brain. These instructions are extremely complex, and so errors occasionally occur. These errors can lead to serious defects in the structure and functioning of the brain. Some infants have intellectual disability at birth because genetic errors caused the brain to develop improperly during the mother’s pregnancy. In Down syndrome, for example, an extra chromosome is present. Chromosomes are structures in the cell nucleus that contain the genes. The extra chromosome causes intellectual as well as physical defects. Another disorder that causes intellectual disability is fragile X syndrome. This disorder results from an abnormality on the X chromosome, one of the chromosomes that determine a person’s sex.
Some children suffer severe brain damage after birth because of an inherited deficiency of an enzyme that the body needs to use foods properly. For example, a child who has phenylketonuria (PKU) lacks an enzyme needed to convert a certain amino acid (one of the building blocks of protein) into a form the body can use. This amino acid, phenylalanine, accumulates in the blood and damages developing brain tissues. A diet low in phenylalanine can prevent brain damage in people who have PKU.
Some genetic errors damage the brain only later in life. Huntington’s disease, for example, usually strikes during middle age. The disease causes some areas of the cerebrum to wither away. Involuntary jerky movements are the main early symptoms of Huntington’s disease. However, the disease eventually leads to incurable mental disintegration.
Scientists believe that genetic factors play an important role in most cases of Alzheimer’s disease. This disease most commonly strikes after age 60. It is characterized by an increasingly severe loss of memory and other mental abilities. Most people with Alzheimer’s disease eventually cannot care for themselves and become bedridden.
Heredity also plays a role in some types of mental illness. Many children of schizophrenics apparently inherit a tendency to develop schizophrenia. Studies have also revealed an inherited tendency to develop bipolar disorder. These tendencies may involve inherited defects in brain chemistry. Researchers continue to study these tendencies and how they interact with environmental conditions to produce mental illness.
Other brain disorders
include (1) epilepsy, (2) multiple sclerosis (MS), (3) cerebral palsy, and (4) Parkinson disease.
Epilepsy.
Victims of epilepsy suffer attacks called seizures. The seizures occur when many nerve cells in one area of the brain release abnormal bursts of impulses that tend to spread to other brain regions. A seizure may cause temporary uncontrolled muscle movements or unconsciousness. Defects in genes cause some cases of epilepsy, but the cause of most cases is not known. Physicians treat epilepsy with drugs that reduce the number of seizures or prevent them entirely.
Multiple sclerosis
develops when axons in parts of the brain and spinal cord lose their myelin sheaths. As a result, the axons cannot carry nerve impulses properly. Symptoms vary depending on what brain areas are affected, but they may include double vision, loss of balance, and weakness in an arm or leg. A major cause of multiple sclerosis is that the body’s own immune system attacks the myelin sheaths. No cure is yet known. Drugs can relieve some of the symptoms. Some of these drugs help slow the loss of myelin.
Cerebral palsy
is a form of brain damage that develops before, during, or soon after birth. There are several types of cerebral palsy, all of which involve a loss of control over muscle movement. Intellectual disability, seizures, and physical deformities may also be a part of cerebral palsy. The cause or causes of cerebral palsy remain unclear, but premature babies are especially likely to develop this defect.
Parkinson disease
is characterized by slowness of movement, muscle rigidity, and trembling. It may also include problems with thinking, learning, memory, and mood. These conditions result mainly from the destruction of specific nerve pathways that use dopamine as a transmitter. Treatment with the drug L-dopa replaces the missing dopamine and so can relieve the symptoms of Parkinson disease, though it cannot cure the illness. Researchers continue to explore the possibility of replacing Parkinson patients’ lost dopamine-producing cells with transplanted cells from other sources.
The brain in other animals
Many aspects of neuron structure and function are nearly identical for almost all animals. But the number of neurons and their arrangement varies widely. Most invertebrates (animals without backbones) do not have a well-developed brain. All vertebrates (animals with backbones) have a complex brain. The human brain is the most complex of all.
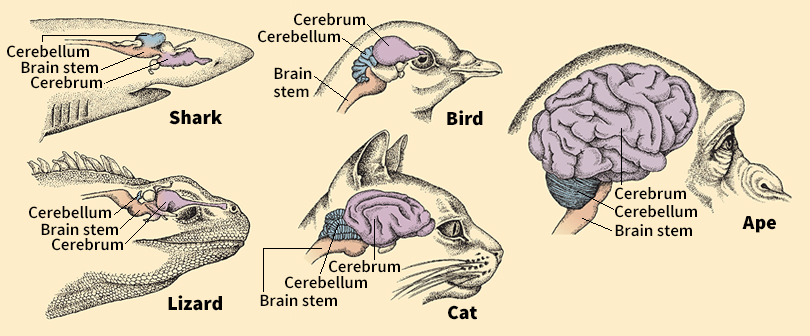
Invertebrates
often lack a brain entirely. For example, jellyfish have a network of neurons distributed over the body, but these creatures lack a distinct brain. Other invertebrates have large clusters of neurons, called ganglia, that coordinate the activities of the body. These clusters can be considered a kind of brain.
Some invertebrates have brains that are surprisingly large in comparison to body size. The brain of a honey bee, for example, includes about 1 million neurons.
The octopus has the largest and most complex brain of any invertebrate. The neurons in the brain of an octopus may number up to about 300 million. The neurons are arranged differently from the neurons in a vertebrate brain, but they perform similar functions. For example, the optic lobe of an octopus’s brain processes information from its eyes. Octopuses are also among the most intelligent invertebrates. In laboratory studies, they quickly learn to solve simple puzzles.
Among vertebrates,
the brain has a cerebrum, cerebellum, and brain stem. The brain stem and the cerebellum are often similar in the different groups of vertebrates, although they may vary greatly in size. The cerebrum, however, varies enormously in size and structure among different groups of vertebrates. In mammals, for example, most of the cerebrum develops into a multilayered cerebral cortex. In reptiles and birds, no such cortex is found. Instead, the cerebrum of reptiles and birds contains large clusters of neurons that are not arranged in distinct layers. In more ancient groups of vertebrates, such as sharks and amphibians, the cerebrum usually makes up only a small part of the brain.
Among vertebrates, brains vary enormously in overall size. The brains of small fish can weigh a tiny fraction of an ounce. The brain of a blue whale, the largest animal, weighs a million times as much, about 15 pounds (7 kilograms). Brain size generally increases with body size, so larger animals tend to have larger brains. Larger-brained animals also tend to be more intelligent and have more complex behaviors. However, a large animal with a large brain is not necessarily more intelligent than a smaller animal with a smaller brain.
Different parts of the brain can vary in proportion among vertebrates. For example, squirrels and rats are both mammals and have brains of roughly equal size. But the main structures of the brain stem responsible for vision are about 10 times larger in squirrels than in rats. Such differences tend to reflect an animal’s behavioral abilities and lifestyle. Squirrels, for example, are active during daylight and rely heavily on eyesight. Rats, on the other hand, are mainly active at night and tend to rely more on smell.
Some vertebrates have brains that are particularly large for their body size. These include several highly intelligent animals, such as whales, dolphins, chimpanzees, and human beings. Scientists have observed that many large-brained animals, including human beings, are highly social. These animals may need large brains to master complex social relationships. However, not all animals with large brains are social. For example, many species of owls have large brains compared to the size of their bodies. But owls do not have the complex social behaviors seen in other birds. Biologists believe that every species has evolved the kind and size of brain that suits its unique needs.