Climate is the weather of a place averaged over a length of time. Climate includes the variability, extremes, and frequency of the various aspects of weather, such as temperature, precipitation, and wind speed. Earth’s climate varies from place to place, resulting in a variety of environments. Thus, on various parts of Earth, there are deserts, prairies, and tropical rain forests. Earth’s surface also features tundras (frozen, treeless plains) and forests of conifer (cone-bearing) trees and bushes. There are even coverings of glacial ice.
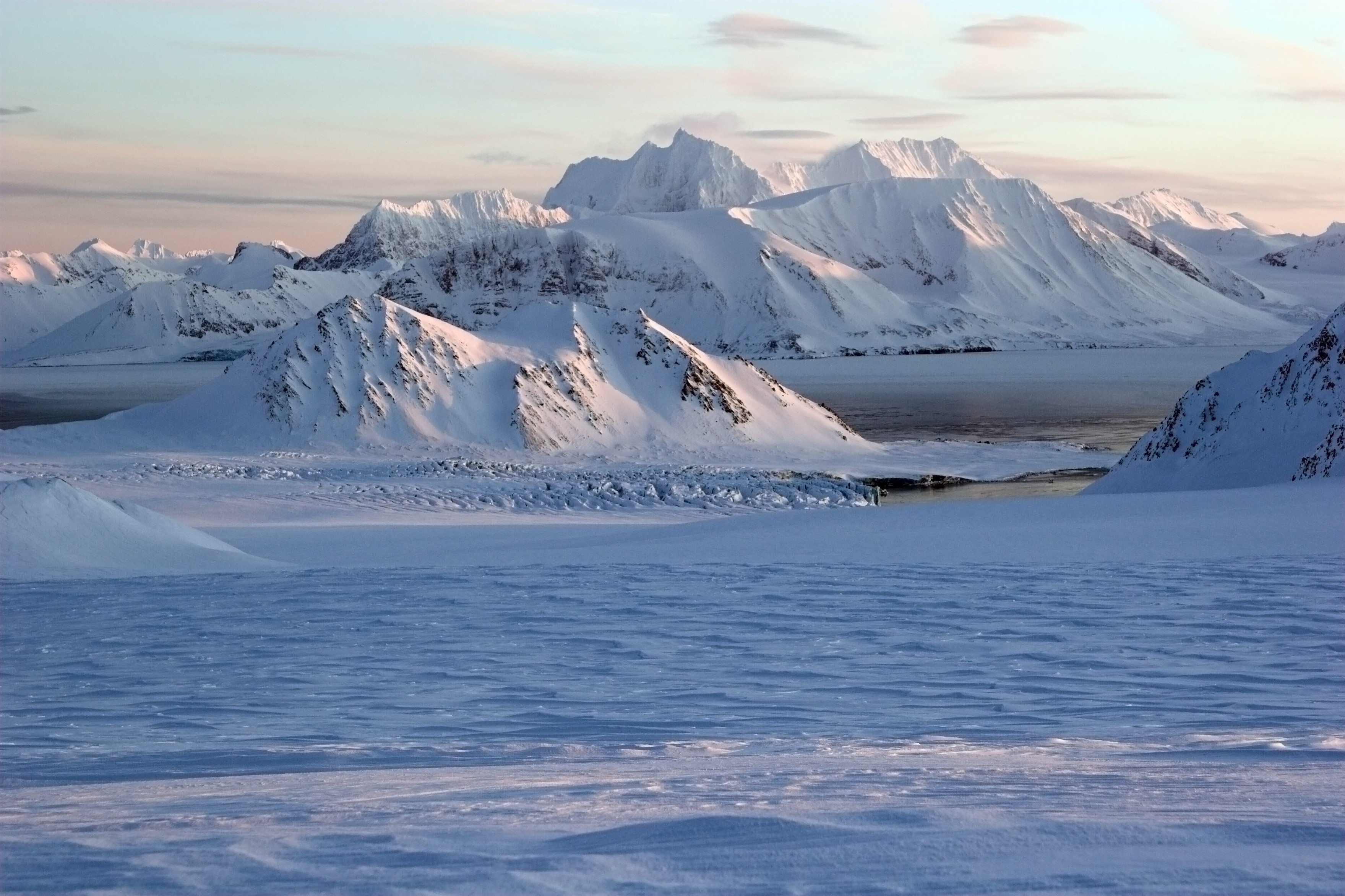
Climate changes with time. For example, a thousand years ago, the northern latitudes were milder than they are today. The warmer climate enabled Vikings from Iceland to settle on the southern coast of Greenland. But the colder climate that developed over the following centuries may have helped to lead Vikings to desert those settlements.
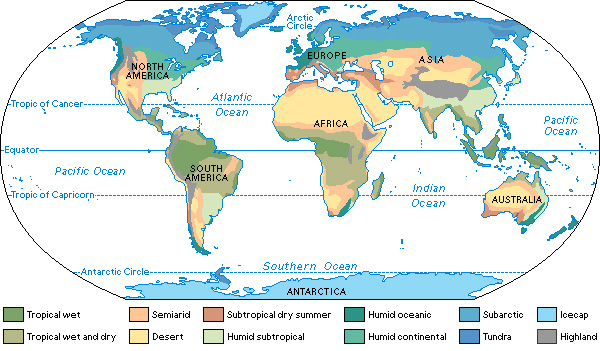
One major environmental concern is that human activity is changing the global climate. The burning of fossil fuels—coal, oil, and natural gas—is increasing. Such fuels power motor vehicles, heat buildings, generate electric power, and perform various industrial tasks. Fossil fuels contain carbon. Burning them releases carbon dioxide (CO2) gas into the atmosphere. The concentration of CO2 in the atmosphere has been increasing greatly since the beginning of the Industrial Revolution, when the widespread burning of fossil fuels began. Atmospheric CO2 slows the escape of heat released by Earth into space. As a result, an increase in atmospheric CO2 can cause global warming—a rise in the temperature of the air near Earth’s surface.
Global warming may already be changing rainfall patterns, leading to shifts in plant and animal populations. It could also melt enough polar ice to raise the sea level significantly, submerging small islands and low-lying coastal areas. It could increase the frequency and severity of tropical storms and other severe weather events.
Why climates vary
Climates vary from place to place because of five main factors—latitude (distance from the equator), elevation (height above sea level), topography (surface features), distance from oceans and large lakes, and the circulation of the atmosphere.
The role of latitude.
The sun continually sends electromagnetic radiation into space. Some of the radiation is visible light. The sun also gives off infrared (heat) rays and ultraviolet rays. About 30 percent of the radiation that reaches Earth’s atmosphere is reflected back into space, mostly by clouds. The remaining 70 percent is absorbed by the atmosphere and by Earth’s surface, heating them.
The intensity of the solar radiation reaching the atmosphere decreases with increasing latitude. The intensity depends on how high in the sky the sun climbs. The closer a place is to the equator, the higher the climb.
At latitudes between the Tropic of Cancer (23 1/2° north) and the Tropic of Capricorn (23 1/2° south), the sun is directly overhead at noon twice a year. At these times, the sun’s rays shine directly down toward the surface. The radiation that reaches the atmosphere in these regions is therefore at its most intense.
In all other cases, the rays arrive at an angle to the surface and are therefore less intense. The closer a place is to the poles, the smaller the angle and therefore the less intense the radiation. Due to decreases in the intensity of radiation, average temperatures decline from the equator to the poles. Seasonal changes in solar radiation and the number of hours of sunlight also vary with latitude.
In tropical latitudes—those near the equator—there is little difference in the amount of solar heating between summer and winter. There is also little difference in number of hours of sunlight throughout the year. Average monthly temperatures therefore do not change much during the year.
In middle latitudes, from the Tropic of Cancer to the Arctic Circle (66 1/2° north) and from the Tropic of Capricorn to the Antarctic Circle (66 1/2° south), there are considerably more hours of daylight in summer than in winter. As a result, solar heating is considerably greater in summer than in winter. In these latitudes, summers are therefore warmer than winters.
At the Arctic Circle and Antarctic Circle, there is at least one summer day per year in which the sun never sets below the horizon and at least one winter day per year in which the sun never rises. In latitudes north of the Arctic Circle and south of the Antarctic Circle, there are even more consecutive hours of daylight in summer and darkness in winter. At the North Pole and South Pole, the sun never sets for the six months centered around summer and never rises for the six months centered around winter. Therefore, the contrast in solar heating between summer and winter is extreme in high latitudes. Winters are bitterly cold, while summers are milder and can reach temperatures above freezing near the Arctic and Antarctic Circles.
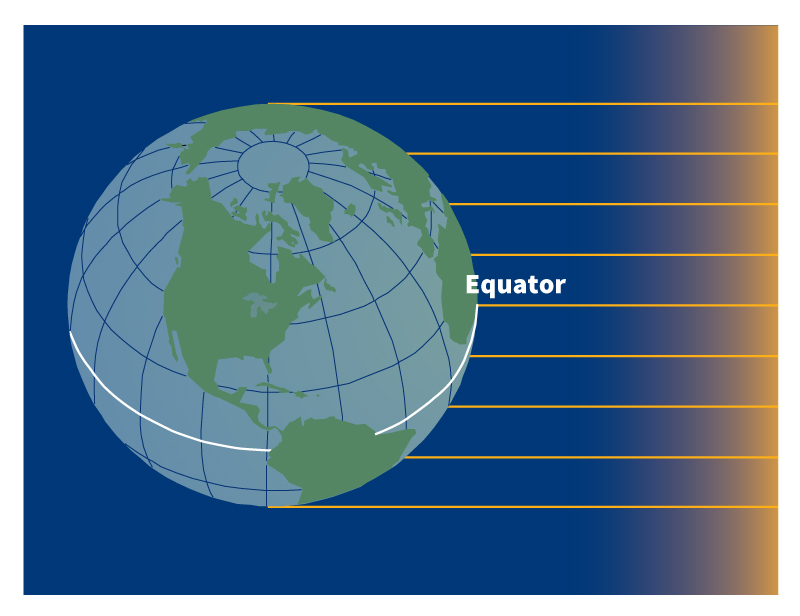
The role of elevation.
The higher a place is, the colder it is. Air temperature drops by an average of about 3.5 Fahrenheit degrees per 1,000 feet of elevation (6.5 Celsius degrees per 1,000 meters). The air temperature determines how much precipitation falls as snow, rather than rain. Even in the tropics, it is not unusual for mountaintops to be snow-covered.
The role of topography.
The surface features of Earth influence the development of clouds and precipitation. As humid air sweeps up the slopes of a mountain range, the air cools, and so clouds form. Eventually, rain or snow falls from the clouds. Some of the rainiest places on Earth are on windward slopes, those facing the wind.
As winds blow down the opposite slopes, known as the leeward slopes, the air warms. Clouds thin out or vanish. Leeward slopes of mountain ranges are therefore dry. The dry area, called a rain shadow, may stretch hundreds of kilometers downwind of a mountain range.
Oceans and large lakes
make the air temperature less extreme in places downwind of them. An ocean or lake surface warms up and cools down more slowly than a land surface. Thus, between summer and winter, the temperature of the water varies less than the temperature of the land. The temperature of the water influences the temperature of the air above it. Therefore, air temperatures over the ocean or a large lake also vary less than air temperatures over land. As a result, places that are immediately downwind of the water have milder winters and cooler summers than places at the same latitude but well inland.
San Francisco and St. Louis, for example, are at about the same latitude and therefore receive about the same amount of solar radiation during the year. But San Francisco is immediately downwind of the Pacific Ocean, and St. Louis is well inland. Consequently, San Francisco has milder winters and cooler summers.
Atmospheric circulation
influences climate by producing winds that distribute heat and moisture. Six belts of wind encircle Earth. These belts consist of two belts of trade winds, two belts of westerlies (winds from the west), and two belts of polar easterlies (winds from the east). The trade winds blow between 30° north latitude and the equator and between the equator and 30° south latitude. The westerlies blow between 30° and 60° north of the equator and between 30° and 60° south of the equator. The polar winds blow north of 60° north latitude and south of 60° south latitude.
Trade winds north of the equator blow from the northeast. South of the equator, they blow from the southeast. The trade winds of the two hemispheres meet near the equator, causing air to rise. As the rising air cools, clouds and rain develop. The resulting band of cloudy and rainy weather near the equator is called the intertropical convergence zone (ITCZ), or doldrums.
Westerlies blow from the southwest in the Northern Hemisphere and from the northwest in the Southern Hemisphere. Westerlies generally steer storms eastward across middle latitudes.
Westerlies and trade winds blow away from the 30° latitude belt. This causes air to sink from aloft near broad regions centered on 30° latitude, replacing the surface air that moved away from the area. Descending air warms and is dry. The tropical deserts, such as the Sahara of Africa and the Sonoran Desert of Mexico, occur under these regions of descending air.
Polar easterlies blow from the northeast in the Arctic and from the southeast in the Antarctic. The boundary between the cold polar easterly winds and the milder westerly winds is known as the polar front. A front is a narrow zone of transition. Most fronts separate a mass of cold air and a mass of warm air. Where the air masses meet, storms can develop. The storms move along the polar front, bringing cloudy weather, rain, or snow.
As the seasons change, the global wind belts shift northward and southward. In the spring, they move toward the poles as the coldest air retreats poleward with the end of winter. In the fall, they shift toward the equator as polar air begins to occupy more of Earth’s surface. These shifts help explain why some areas have distinct rainy seasons and dry seasons. Parts of Central America, North Africa, India, and Southeast Asia have wet summers and dry winters. Southern California and the Mediterranean coast have dry summers and wet winters because the descending air near the 30° latitude belt makes its closest approach in summer.
Kinds of climates
Earth’s surface is a patchwork of climate zones. Climatologists (scientists who study the climate) have organized similar types of climates into groups. This article uses a modified version of a classification system introduced in 1918 by Wladimir Köppen, a German climatologist. Köppen based his system on a region’s vegetation, average monthly and annual temperature, and average monthly and annual precipitation.
The modified version specifies 12 climate groups: (1) tropical wet, (2) tropical wet and dry, (3) semiarid, (4) desert, (5) subtropical dry summer, (6) humid subtropical, (7) humid oceanic, (8) humid continental, (9) subarctic, (10) tundra, (11) icecap, and (12) highland.
Tropical wet climates
are hot and muggy the year around and lie in or around the ITCZ. They support dense tropical rain forests. Rainfall is heavy. Rain occurs in frequent showers and thunderstorms throughout the year. Average annual rainfall varies from about 70 to 100 inches (175 to 250 centimeters). In a few places, where the humid air moves upslope, annual precipitation can even exceed 400 inches (1,000 centimeters)
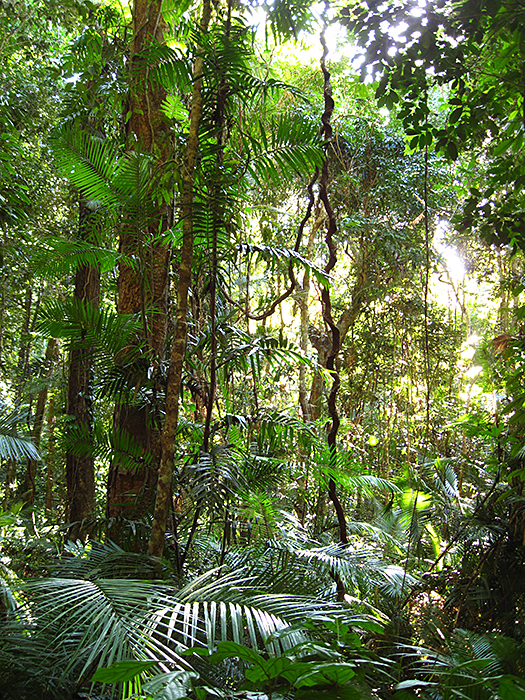
Temperatures are high in tropical wet climates and change little during the year. The coolest month has an average temperature no lower than 64 °F (18 °C). The temperature difference between day and night is greater than the temperature difference between summer and winter. Frost and freezing temperatures do not occur. Plants grow the year around.
Tropical wet and dry climates
occur in areas closer to the descending air belt, next to regions that have tropical wet climates. Temperatures in tropical wet and dry climates are similar to those in tropical wet climates. They remain high throughout the year.
The main difference between the two climates lies in their rainfall. In tropical wet and dry climates, winters are dry, and summers are wet. Generally, the length of the rainy season and the average rainfall decrease closer to 30° latitude. Not enough rain falls in tropical wet and dry climates to support rain forests. Instead, they support savannas—grasslands with scattered trees.
Semiarid and desert climates
occur in regions with little precipitation. Desert climates are drier than semiarid climates. Semiarid climates, also called steppe climates, usually border desert climates. In both climate groups, the temperature change between day and night is considerable. One reason for the wide swings in temperature is that the skies are usually clear and the air is dry. Thus, there are few clouds to reflect the sun’s radiation during the day, enabling the air near the surface to heat rapidly. At night, the lack of clouds and water vapor leaves little to absorb the radiation given off by the ground. Most of this radiation consists of infrared rays. Thus, as a result of clear skies, rapid cooling occurs at night.
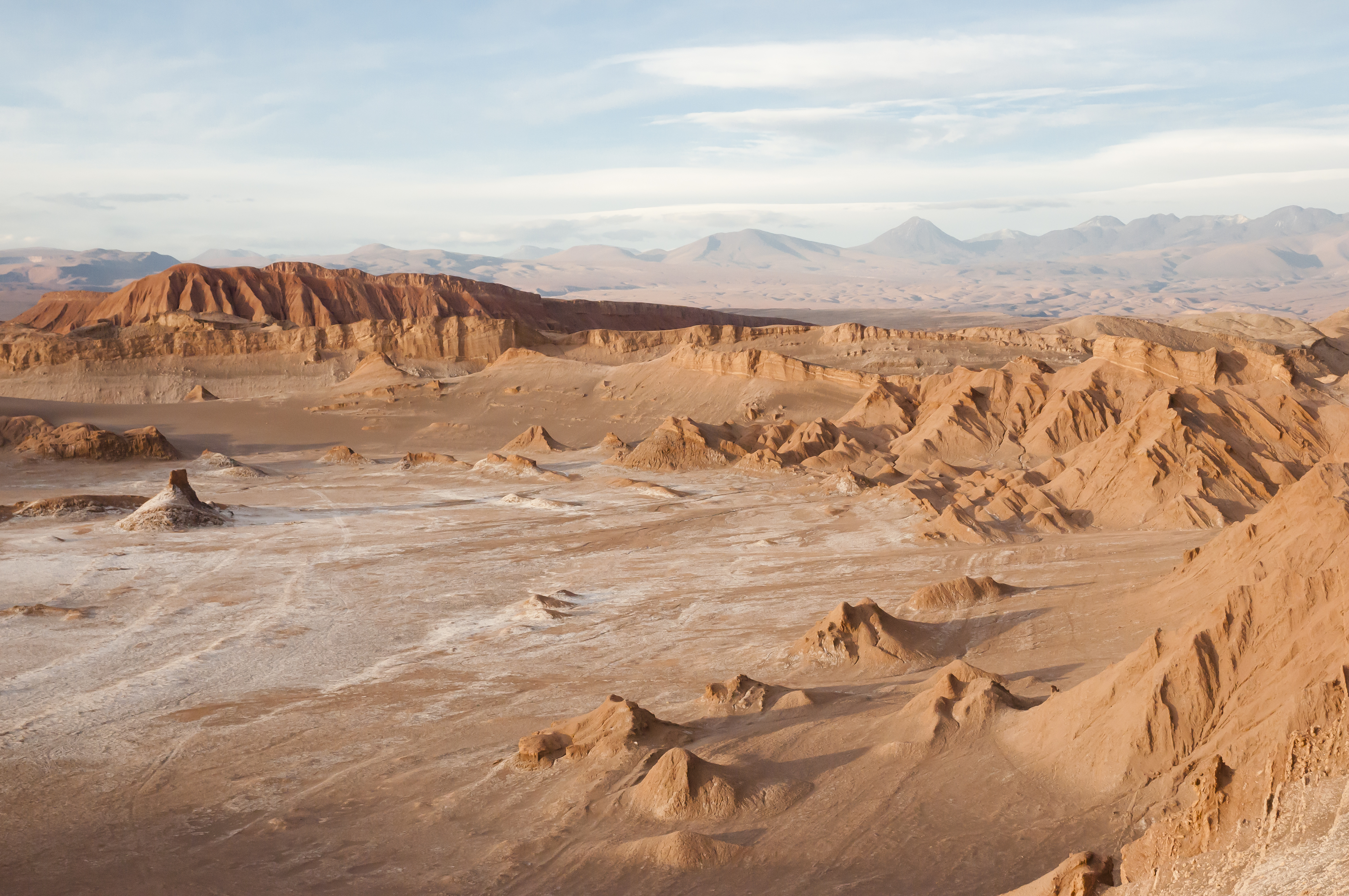
Semiarid and desert climates occur over a greater land area than any other climate grouping. They occur in both tropical and middle latitudes. They cover broad east-west bands near 30° north and south latitude.
Middle latitude semiarid and desert climates are in the rain shadows of mountain ranges, usually far from oceans. Winds that descend the leeward slopes of these ranges are warm and dry. Middle latitude semiarid areas and deserts differ from their tropical counterparts mainly in their seasonal temperature changes. Winters are much colder in middle latitude semiarid areas and deserts.
Subtropical dry summer climates
feature warm to hot, dry summers and mild, rainy winters. These climates are sometimes called Mediterranean climates. They occur on the west side of continents roughly between 30° and 45° latitude. The closer to the coast the area is, the more moderate the temperatures. There is also less contrast between summer and winter temperatures in coastal areas.
Humid subtropical climates
are characterized by warm to hot summers and cool winters. Rainfall is distributed fairly evenly throughout the year. Winter rainfall—and occasionally snowfall—is associated with large storm systems that the westerlies steer from west to east. Most summer rainfall occurs during thunderstorms and an occasional tropical storm or hurricane. Humid subtropical climates lie on the southeast side of continents, roughly between 25° and 40° latitude.
Humid oceanic climates
are found on the western sides of continents where prevailing winds blow from sea to land. The moderating influence of the ocean reduces the seasonal temperature contrast. Winters are cool to mild, and summers are warm. Moderate precipitation occurs throughout the year. Low clouds, fog, drizzle, and some snowfall are common. Thunderstorms, extreme cold, heat waves, and droughts are rare.
Humid continental climates
feature mild to warm summers and cold winters. The temperature difference between the warmest and coldest months of the year increases inland. The difference is as great as 45 to 63 Fahrenheit degrees (25 to 35 Celsius degrees). Precipitation is distributed fairly evenly throughout the year. But many locations well inland have more precipitation in the summer.
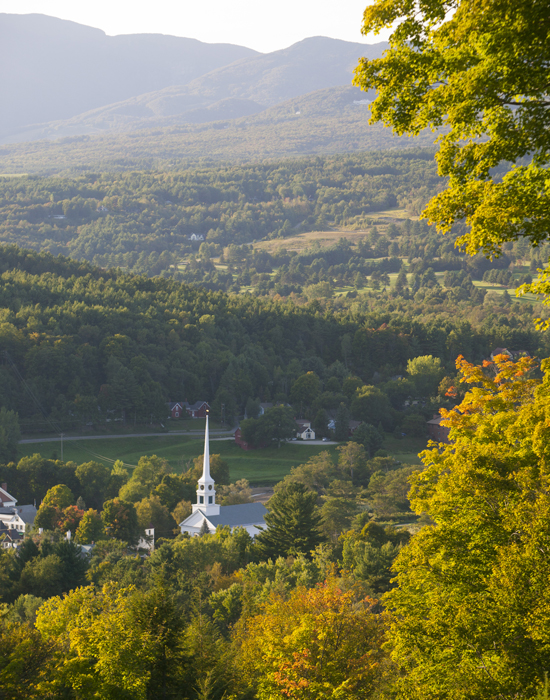
Snow is a major element in humid continental climates. Winter temperatures are so consistently low that snowfall can be substantial and snow cover persistent. Snow cover has a chilling effect on climate. Snow strongly reflects solar radiation back into space, lowering daytime temperatures.
Subarctic climates
have short, cool summers and long, bitterly cold winters. Freezes can occur even in midsummer. Most precipitation falls in the summer. Snow comes early in the fall and remains on the ground into early summer.
Tundra climates
are dry. They have a brief, chilly summer and a bitterly cold winter. Continuous permafrost (permanently frozen ground) lies under much of the treeless tundra regions.
Icecap climates
are the coldest on Earth. They occur over the vast ice sheets that cover Greenland and Antarctica. Summer temperatures rarely rise above the freezing point. Temperatures are extremely low during the long, dark winter. Precipitation is meager and is almost always in the form of snow.
Highland climates
occur in mountainous regions. A highland climate zone is composed of several areas where climates are like those found in flat terrain. Because air temperature decreases with increasing elevation in the mountains, each climate area is restricted to a certain range of elevation.
A mountain climber may encounter the same sequence of climates in several thousand feet or meters of elevation as he or she would encounter traveling northward several thousand miles or kilometers. For example, the climate at the base of a mountain might be humid subtropical, and the climate at the summit might be tundra.
Causes of climate change
Climate changes over time. About 18,000 years ago, a sheet of glacial ice up to 10,000 feet (3,000 meters) thick covered much of what is now Greenland, Canada, the northern United States, and other parts of the world. A warming trend gradually melted almost all the glaciers, except in Antarctica, Greenland, and the tops of high mountains. In Canada and the United States, the last large fields of ice had disappeared by about 11,500 years ago. The warming trend ended after a mild period from 7,000 to 5,000 years ago, when the global average temperature was higher than it is today.
Within the past 1,200 years, the period between about A.D. 950 and 1250 was mild. The years from about 1400 to 1850 were so cool that people nicknamed this period the Little Ice Age. Since then, global average temperatures generally have risen.
Many natural processes influence changes in a region’s climate. Some of these processes, such as volcanic eruptions, are short-lived and cause short-term changes. Other processes, such as mountain building, occur over long periods and cause long-term changes in climate. Human activity also has an effect on climate.
Volcanic eruptions
can cause short-term cooling over large portions of the planet. Cooling is especially strong if the eruptions throw large amounts of sulfur gases high into the atmosphere. The sulfur gases combine with moisture to produce droplets of sulfuric acid and tiny sulfate particles. The sulfur droplets and particles created by major volcanic eruptions absorb some solar radiation and reflect some back to space. As a result, less solar radiation reaches Earth’s surface, and so air temperatures fall.
Because the droplets and particles are so small, they can remain suspended in the atmosphere for months or years. Meanwhile, winds carry them around the globe. Scientists believe that volcanic eruptions can cause a maximum global cooling of about 2 Fahrenheit degrees (1 Celsius degree).
In 1991, the eruption of Mount Pinatubo in the Philippines threw large amounts of sulfur gases high into the atmosphere. This eruption likely caused a drop of 1.1 Fahrenheit degree (0.6 Celsius degree) in the global average temperature during the following few years.
Changes in ocean circulation
can also alter the climate. For example, changes in ocean currents that occur during El Niño can affect the climate for a year or two.
El Niño is a large-scale interaction between the tropical atmosphere and tropical oceans that happens over a period of months about every two to seven years. Changes in the air pressure over the tropical Pacific Ocean cause the trade winds there to weaken or even reverse direction. This change enables the warm waters of the ocean surface to drift from the western tropical Pacific to the eastern tropical Pacific. This flow makes sea-surface temperatures lower than usual over the western tropical Pacific and higher than usual over the eastern tropical Pacific.
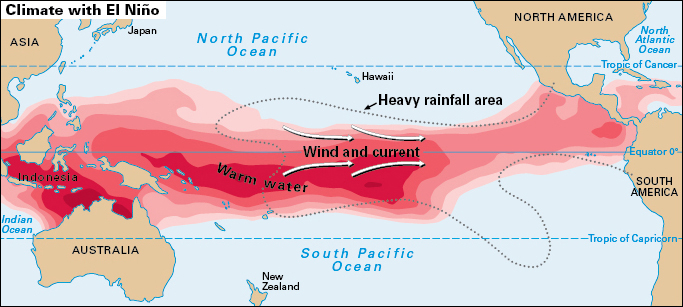
The sea-surface temperature changes, in turn, alter the circulation of the atmosphere in tropical and middle latitudes. These alterations cause weather extremes in various parts of the world. Heavy rains drench the normally arid coast of western South America. Drought is more likely in Hawaii and eastern Australia. Winters tend to be wetter than normal along the Gulf of Mexico coast.
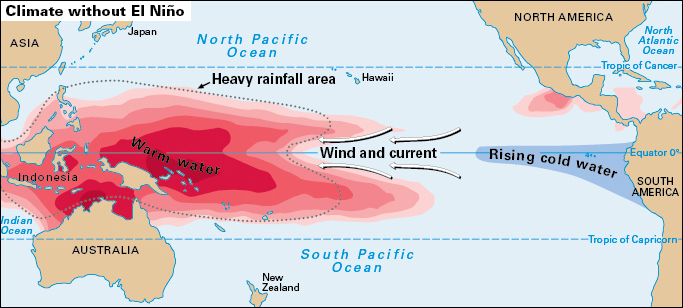
Activity on the sun’s surface
may affect Earth’s climate for short periods. Sunspots are dark, relatively cool blotches that appear on the surface of the sun. Faculae are relatively bright, hot areas on the solar surface. The number of sunspots and faculae increases and decreases over a cycle of about 11 years. The average amount of energy given off by the sun is slightly higher during a sunspot maximum. At such times, the number of sunspots and faculae is high. The average amount is slightly lower during a sunspot minimum—when the number is low.
Climatologists are not sure about the relationship between changes on the sun’s surface and variations in Earth’s climate. During the period from 1645 to 1715, the number of sunspots was unusually low. This episode corresponds to a portion of the Little Ice Age. Climatologists have not proven, however, that the reductions in the number of sunspots caused the cooling.
Changes in carbon dioxide concentration
in the atmosphere can cause short-term and long-term variations in the climate. Atmospheric CO2 slows the flow of heat from Earth to space. This gas absorbs heat that radiates from Earth’s surface and radiates heat back to the surface.
Human activity is currently increasing the concentration of atmospheric CO2. This concentration has also varied naturally throughout the history of Earth. With past variations in atmospheric CO2, the global climate has warmed or cooled. For example, about 100 million years ago, volcanic activity on the floor of the Pacific Ocean may have released enough CO2 to cause global warming of perhaps 18 Fahrenheit degrees (10 Celsius degrees).
Changes in Earth’s orbit
about the sun may cause climate changes over tens of thousands to hundreds of thousands of years. Milutin Milankovitch, a Serbian mathematician, proposed in the early 1900’s that three planetary variations affect Earth’s climate. These variations change how sunlight is distributed seasonally and geographically over the planet. The variations are a precession (wobble) of Earth’s axis, a cycle in the tilt of the axis, and a cycle in the path of Earth’s orbit around the sun.
The precession of the axis varies over a period of 23,000 years. This cycle alters the times of the year when the Northern Hemisphere is tilted toward and away from the sun. Today, the Northern Hemisphere is tilted toward the sun in June, causing summer in the Northern Hemisphere (and winter in the Southern Hemisphere). In 11,500 years, however, the Northern Hemisphere will be tilted away from the sun in June, and it will be winter in the Northern Hemisphere (and summer in the Southern Hemisphere).
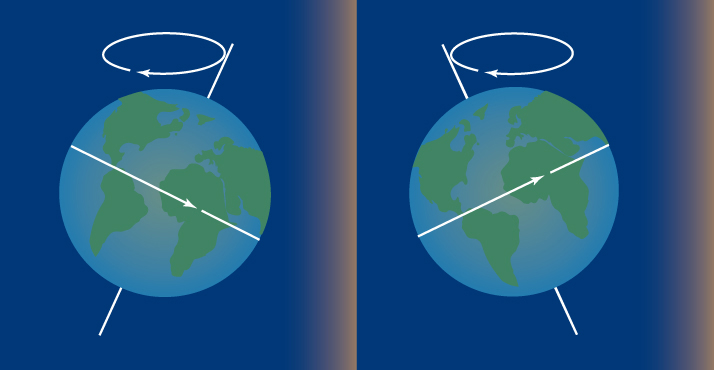
The tilt of the axis changes from 22.1° to 24.5° over a period of 41,000 years. This cycle affects the contrast between winter and summer temperatures. When the axis is tilted further toward the equator, the direct rays of the sun penetrate farther poleward. This factor tends to make summers warmer and winters colder outside the tropics.
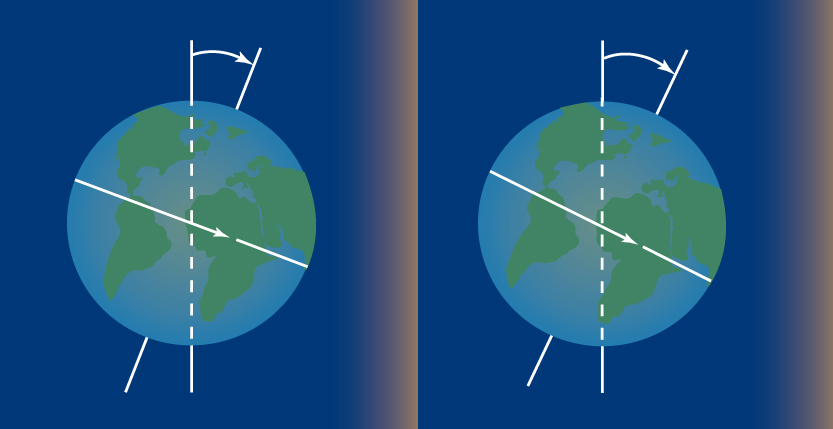
The path of the orbit varies over a period of about 100,000 years. The orbit is always elliptical—that is, shaped like a flattened circle. But during the 100,000-year cycle, the amount of flatness changes from a maximum to a minimum, then back to the maximum. This variation changes the distance between Earth and sun over the course of a year, affecting the amount of solar radiation Earth receives.

These three cycles probably have altered global temperatures at regular intervals throughout Earth’s history. For example, climatologists believe that the cycles may have governed the major fluctuations in the planet’s glacial ice cover during the Pleistocene Epoch. The Pleistocene was the period from about 2.6 million years ago to 11,500 years ago. The three cycles probably produce temperature changes that cause the ice cover to expand and contract at regular intervals.
Continental drift
is an extremely slow process that influences the climate over tens of millions of years. The planet’s solid outer shell, which is typically 60 miles (100 kilometers) thick, is divided into about 30 vast plates. The plates move slowly over the surface of the globe. The continents are embedded in these plates and slowly drift with them. The process is known as continental drift. About 200 million years ago, there was just one huge continent, called Pangaea. Pangaea slowly split into fragments that drifted apart. Eventually, the fragments reached their present locations as the continents we know today.
Continental drift helps explain the presence of coral reef fossils in Wisconsin and of tropical plant fossils in areas north of the Arctic Circle. When the coral and plants were alive, Wisconsin and the Arctic regions were at much lower latitudes than they are today.
Mountain building,
another extremely slow process, likely helped set the stage for the ice ages during the Pleistocene Epoch. About 40 million to 50 million years ago, the Himalaya and the adjacent Tibetan plateau began to rise in southern Asia. At about the same time, mountain building began in the western part of North America. Half the total uplift of the Himalaya and the Colorado Plateau may have occurred within the past 10 million years. The rise of these massive landforms likely altered the wind belts that encircle the planet. As a result, Earth’s climate became more diverse, wetter, and colder.
Human activity
also affects the climate. The building of cities and the clearing of forests can cause climatic changes. So can the burning of oil, coal, and natural gas. Climatologists disagree, however, about the extent of the impact of human activity on climate, particularly on the recent global warming trend.
The construction of cities creates areas that are warmer and usually drier than the surrounding countryside. Cities are warmer for several reasons. The use of storm drainage systems means that less solar radiation is used to evaporate water and more is used to heat the city surfaces and air. The brick, asphalt, and concrete surfaces readily radiate the heat they absorb and so raise urban air temperatures even more. In addition, cities themselves generate heat from a number of sources, including motor vehicles and heating and air conditioning systems.
Cities are drier than the surrounding countryside because their storm sewer systems quickly carry off rainwater and snowmelt. Exceptions occur in desert cities where water is pumped from elsewhere, creating artificial green spaces (areas of plant growth).
Large urban areas also affect the climate in the areas downwind of them. Smokestacks and automobile tailpipes in cities emit water vapor and tiny particles that stimulate the formation of clouds. Heat from a city also spurs the growth of clouds. Thus, the climate downwind from many large urban areas is cloudier and wetter than the climate upwind from those same areas.
The burning of fossil fuels has contributed to recent increases in the amount of CO2 in the atmosphere. Since the mid-1800’s, the concentration of atmospheric CO2 has risen by about 50 percent. Most of this increase resulted from an increased use of fossil fuels for transportation, space heating, and the generation of electric energy. The clearing of forests also contributes to the buildup of atmospheric CO2 by reducing the rate at which the gas is removed from the air. Trees and other green plants remove CO2 from the air as they grow.
Global temperature records indicate that a warming trend began in the late 1800’s, corresponding with a period of rapid increase in fossil fuel use. It was interrupted by an episode of cooling from about 1940 to the late 1970’s. The cooling effect of the Mount Pinatubo eruption also interrupted the warming trend in the early 1990’s. Most scientists argue that the buildup of atmospheric CO2 due to human activities has caused the warming trend. A few scientists argue that natural fluctuations have a more important role in recent warming.
Determining past climates
Scientists study the climate record to learn about climate and its changes. The most reliable portion of the record is based on standardized measurements by weather instruments.
The reliable instrument-based record dates back only about 135 years. It is too short to reveal all possible variations in climate. Climatologists have lengthened the record by various means. For example, they study historical documents. They examine tree growth rings, fossil plants and animals, and deposits of pollen. They also look at cores drilled out of glacial ice and seafloor sediments and mineral deposits called speleothems that form in caves.
Historical documents
that contain information about climate include logs maintained by ships’ captains and lighthouse keepers, diaries kept by farmers, and records of harvests. Another source is a record of the duration of ice cover in a harbor.
Growth rings.
A tree adds a growth ring each year. The thickness of those rings depends to some extent on seasonal weather conditions. By analyzing growth rings from living and dead trees, scientists can distinguish years of relatively favorable weather from years of stressful weather. In the southwestern United States, tree growth rings provide information about the climate record dating back almost 8,000 years.
Fossils
are the remains or imprints of organisms that lived in the past. Based on an understanding of the environmental conditions required by these organisms, scientists can reconstruct climatic conditions of the places where the fossils are found. Knowing the age of fossils, scientists can identify major shifts in climate.
Pollen
consists of tiny grains that play an essential role in the reproduction of flowering and cone-bearing plants. Pollen is released by the plants and carried by the wind. Some pollen falls into lakes and settles to the bottom along with tiny bits of clay and other particles to form sediment.
Scientists can drill into a lake bottom and extract a sediment core—a sample of many layers of sediment. The core contains a record of changes in pollen, which reflect changes in a region’s vegetation. Because climate largely determines the type of vegetation in a region, climatologists can use the pollen record to reconstruct an area’s climate record.
For example, climatologists have used pollen records to determine that the average July temperature in western Europe was 3.5 Fahrenheit degrees (2 Celsius degrees) warmer 6,000 years ago. In parts of the midwestern United States, climatologists have reconstructed changes in climate from the pollen record as far back as 12,000 years ago.
Glaciers
are composed of layers of ice created by the compression of winter snows. Each layer corresponds to one winter’s snowfall. Through the chemical analysis of ice layers, scientists can estimate winter temperatures. Tiny air bubbles trapped in the ice also provide clues about the chemical composition of the atmosphere when the snow fell. Scientists have obtained lengthy ice cores from ice sheets in Antarctica and Greenland. Cores extracted in Greenland in the early 1990’s covered a period of almost 200,000 years.
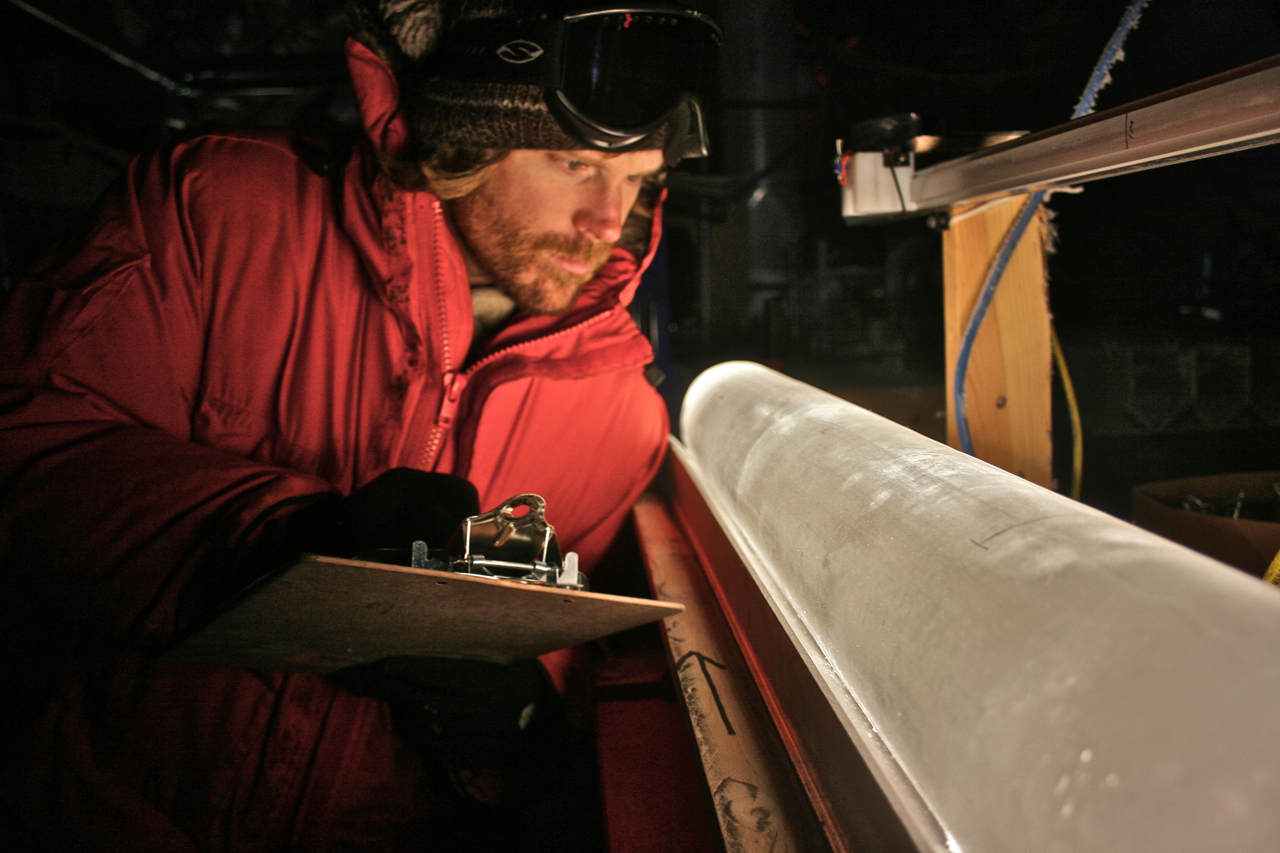
Sea-floor sediments.
The tiny shell and skeletal remains of organisms settle out of ocean water and accumulate with other sediment on the sea floor. A core extracted from sea-floor sediment provides a record of marine life through time. Scientists use chemical analysis of shells and skeletons removed from the sediment core to reconstruct seawater temperatures. Analysis of deep-sea sediments revealed much of what is known about the climatic variations of the Pleistocene Epoch.
Speleothems
form when water seeps into a cave and deposits dissolved minerals on its floor, walls, or ceiling. Scientists have dated material in speleothems by measuring the relative amounts of certain chemical elements. Speleothems can be dated as far back as 600,000 years. By studying speleothems in distant parts of the world, scientists have found a strong link between rainfall patterns in the two hemispheres over the last 90,000 years.
Climate models
Climatologists rely on powerful and complex models to predict Earth’s climate. A climatologist programs a computer with a numerical model of the climate. The model consists of a set of mathematical equations that describe how various factors influence climate. By altering one or more factors, the climatologist can use the model to predict changes in climate. One common application of climate models is to predict the impact of rising concentrations of atmospheric CO2.