Earth is the planet that is home to human beings and other living things. It is one of several rocky planets that revolve around the sun. The sun is a star—one of hundreds of billions of stars that make up a galaxy called the Milky Way. The Milky Way and billions of other galaxies make up the universe.
The planet Earth is only a tiny part of the universe, measuring about 13,000 kilometers (8,000 miles) in diameter. But Earth is home to all known life. Animals, plants, and other organisms (living things) are found almost everywhere on Earth’s surface. Most of these living things rely on the sun’s warmth and light for life. Earth is close enough to the sun to receive plenty of sunlight. The planet’s atmosphere also helps hold in heat. In addition, living things rely on water. Water covers most of Earth’s surface.
The study of Earth is called geology, and scientists who study Earth are geologists. Geologists study different physical features of Earth to understand how the fea tures formed and how they have changed over time. Much of Earth—such as the deep interior—cannot be studied directly. Geologists must often study samples of rock and use indirect methods to learn about Earth. Geologists also use certain satellites to study and view Earth from space.
This article deals with Earth’s appearance, structure, and history as a planet. For information on Earth as a place where people live, see the article World.
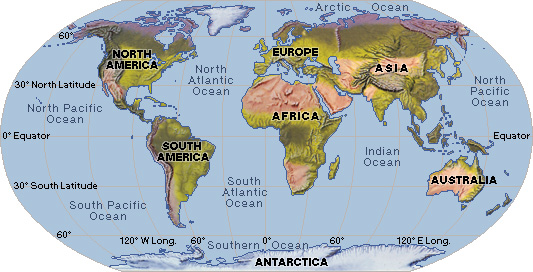
Earth as a planet
Earth ranks fifth in size among the sun’s planets. Jupiter, the largest planet, has a diameter about 11 times that of Earth. Mercury, the smallest planet, has a diameter about two-fifths that of Earth.
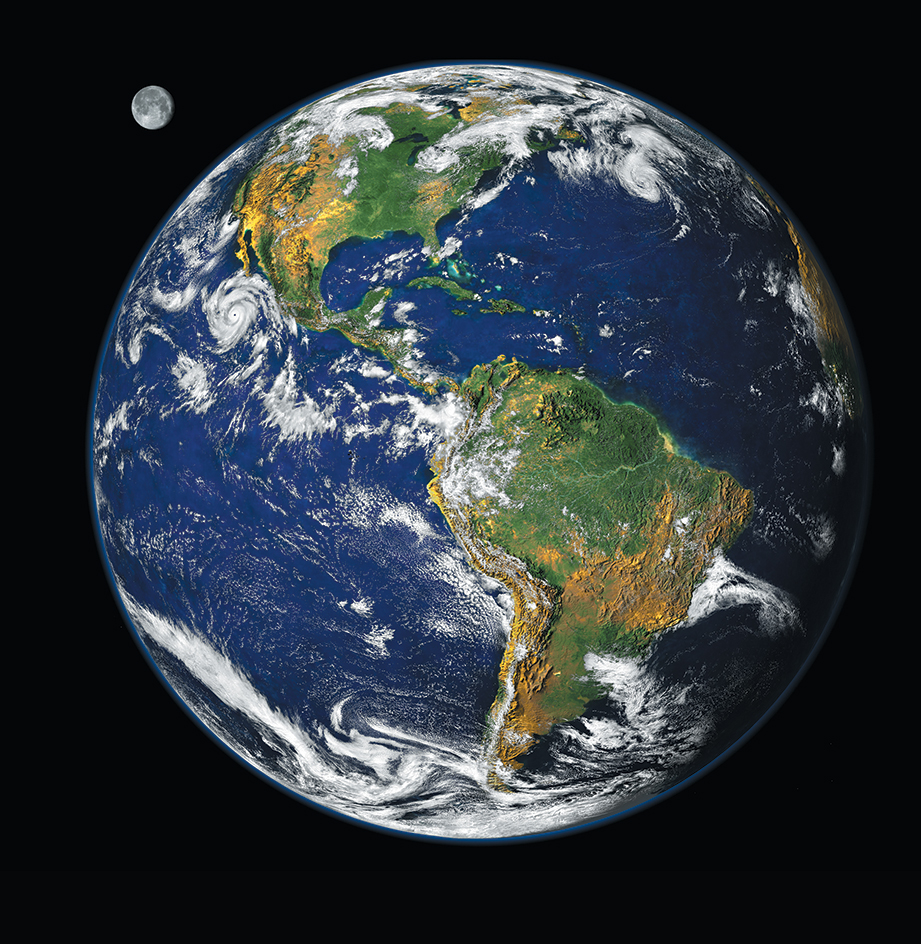
Earth, like all the planets in the solar system, travels around the sun in a path called an orbit. Earth is about 93 million miles (150 million kilometers) from the sun. It takes one year for Earth to complete one orbit around the sun. The innermost planet, Mercury, is only about one-third as far from the sun as is Earth. Mercury circles the sun in only 88 days. Neptune, the outermost planet, is 30 times as far from the sun as is Earth. Neptune takes 165 Earth years to circle the sun.
Size and shape.
People often picture Earth as a ball with the North Pole at the top and the South Pole at the bottom. Earth—like most objects in space bigger than about 200 miles (320 kilometers) in diameter—is round because of its gravity. Gravity pulls matter in toward the center of a massive object. Earth’s gravity also holds people and other bodies to its surface.
Earth is not perfectly round, however. Earth spins, causing the planet to bulge slightly at its middle, near an imaginary line called the equator. The diameter of Earth from North Pole to South Pole is 7,899.83 miles (12,713.54 kilometers). But its diameter through the equator is 7,926.41 miles (12,756.32 kilometers). The difference, 26.58 miles (42.78 kilometers), is only 1/298 the diameter of Earth. It is too tiny to be easily seen in pictures from space, so the planet appears round.
Earth’s bulge also makes its circumference larger around the equator than around the poles. The circumference around the equator is 24,901.55 miles (40,075.16 kilometers). Around the poles, however, it is only 24,859.82 miles (40,008.00 kilometers). Earth’s surface has mountains and valleys. But these features are tiny com pared with the overall size of the planet, causing Earth to appear smooth from space.
Earth and its moon.
Earth has one moon. Earth’s moon has a diameter of about 2,159 miles (3,474 kilometers)—about one-fourth of Earth’s diameter.
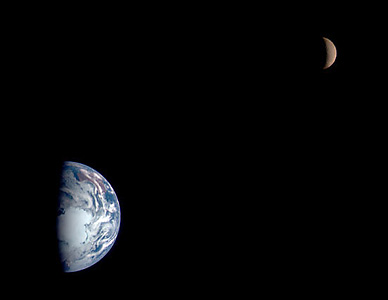
The sun’s gravity acts on Earth and the moon together as if the two were one body with a center about 1,000 miles (1,600 kilometers) below Earth’s surface. This spot is called the Earth-moon barycenter. The barycenter is the point of balance between the heavier Earth and the lighter moon. The path of the barycenter around the sun is a smooth curve. Earth and the moon circle the barycenter as they orbit. As a result, each appears to “wobble” in its path around the sun.
How Earth moves.
Earth moves through space with three basic motions, all at the same time. In one motion, Earth spins like a top. It spins around an imaginary line called an axis that runs from the North Pole to the South Pole. In another motion, Earth travels around the sun. Finally, Earth moves through the Milky Way along with the sun and the rest of the solar system.
Earth takes 24 hours to spin completely around on its axis so that the sun is in the same place in the sky. This period is called a solar day. During a solar day, Earth moves a little in its orbit. As a result, it faces the stars a bit differently each night. Thus, Earth takes only 23 hours 56 minutes 4.09 seconds to spin once so that the stars appear to be in the same place in the sky. This period is called a sidereal day. A sidereal day is shorter than a solar day, so the stars appear to rise about 4 minutes earlier each day.
Earth takes 365 days 6 hours 9 minutes 9.54 seconds to circle the sun. This length of time is called a sidereal year. Because Earth does not spin a whole number of times as it goes around the sun, the calendar gets out of step with the seasons by about 6 hours each year. Nearly every four years, a day is added to bring the calendar back into line with the seasons. Such years are called leap years and have 366 days. The extra day is added to the end of February as February 29.
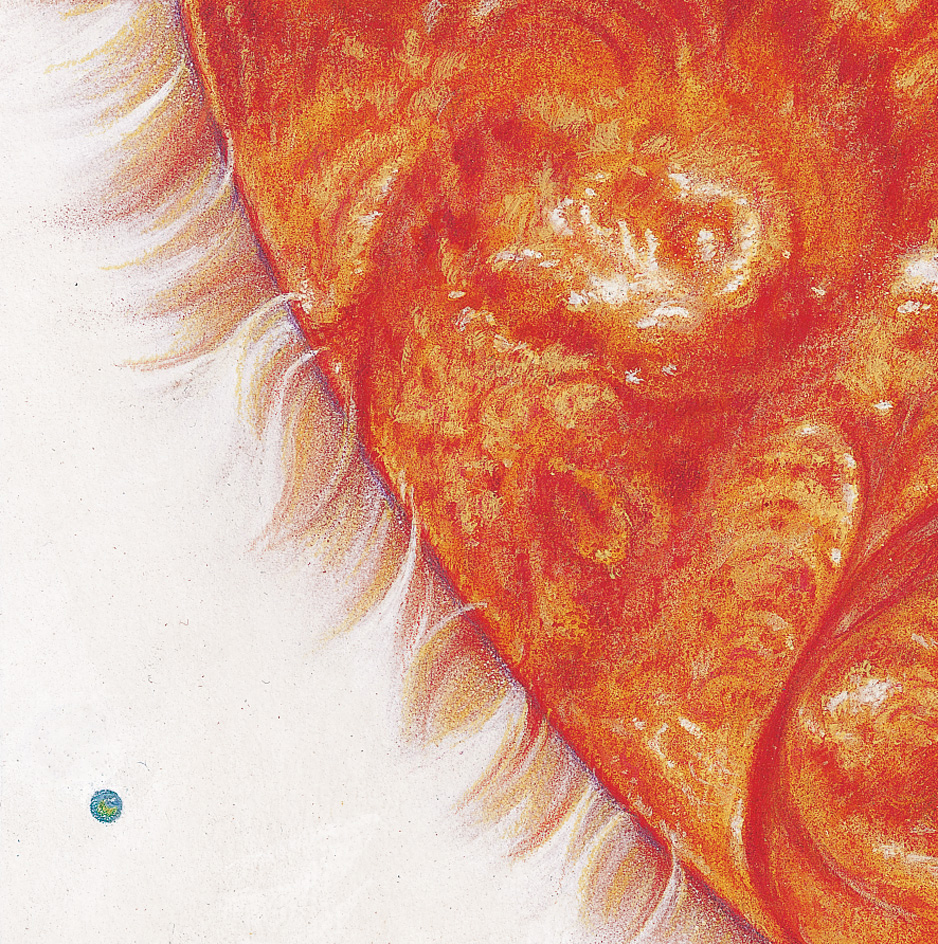
The distance Earth travels in a year is 584 million miles (940 million kilometers). Earth moves along its orbit at 66,700 miles (107,000 kilometers) an hour, or 18.5 miles (30 kilometers) a second. Earth’s orbit lies on an imaginary flat surface around the sun called the orbital plane.
Earth’s axis is not straight up and down. Rather, it is tilted by about 23 1/2 degrees to the orbital plane. This tilt and Earth’s motion around the sun combine to cause the seasons. In January, the northern half of Earth is pointed away from the sun. Sunlight is spread thinly over the northern half of Earth, and the north experiences winter. At the same time, Earth’s southern half is tilted toward the sun. The sunlight falls intensely there, resulting in summer. By July, Earth has moved to the opposite side of its orbit. Now the northern half of Earth is tilted toward the sun. Sunlight falls intensely over the northern half of Earth, and the north experiences summer. At the same time, the sunlight falls less intensely on the southern half, which has winter.
Earth’s orbit is not a perfect circle. Earth is slightly closer to the sun in early January (winter in the Northern Hemisphere) and farther away in July. In January, Earth is 91.4 million miles (147.1 million kilometers) from the sun. In July, it is 94.5 million miles (152.1 million kilometers) from the sun. This variation in distance has a far smaller effect on weather than does the tilt of Earth’s axis. Over thousands of years, the time of year at which Earth is closest to the sun slowly changes.
Just as the moon orbits Earth and planets orbit the sun, the sun and other stars orbit the tightly packed center of the Milky Way. The solar system is about two-fifths of the way from the center of the Milky Way to its edge. The solar system revolves around the galaxy’s center at about 137 miles (220 kilometers) per second. The solar system makes one complete revolution around the center of the galaxy in about 240 million years.
Earth’s spheres
Earth can be divided into several large systems called spheres. The solid Earth consists of a thin outer layer called the crust. A thick rocky layer called the mantle lies beneath the crust. The crust and the upper portion of the mantle make up a layer of rock called the lithosphere. Much of Earth is covered by a layer of water or ice, called the hydrosphere. Earth is surrounded by a thin layer of air, the atmosphere. The portion of the hydrosphere, atmosphere, and lithosphere that serves as home to life is called the biosphere.
Loading the player...Urban heat island effect
The atmosphere.
Air surrounds Earth, becoming progressively thinner farther from the surface. Most people find it difficult to breathe more than 2 miles (3.2 kilometers) above sea level. About 100 miles (160 kilometers) above the surface, the air is so thin that satellites can travel without much resistance. Traces of atmosphere, however, can be found as high as several hundred miles or kilometers above Earth’s surface. The atmosphere has no definite outer edge but fades gradually into space.
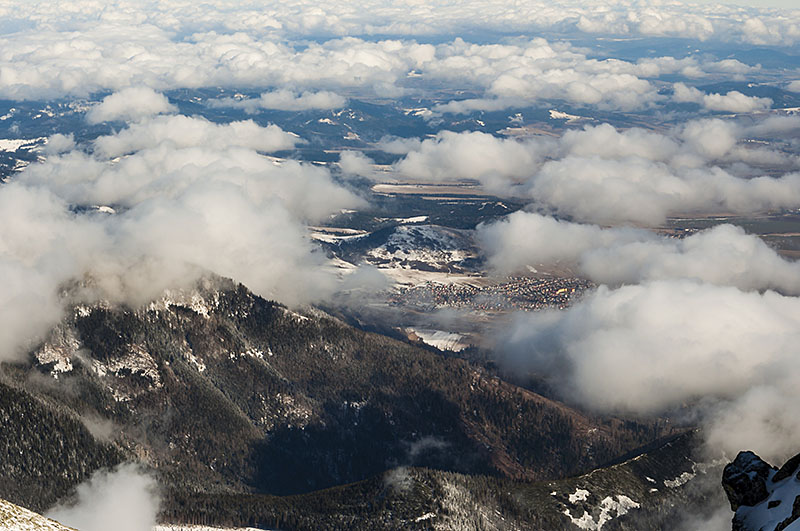
Nitrogen makes up 78 percent of the atmosphere. Oxygen makes up 21 percent. The remaining 1 percent consists of argon and small amounts of other gases. The atmosphere also contains water vapor, carbon dioxide, water droplets, dust particles, and small amounts of many other chemicals released by volcanoes, fires, living things, and human activities.
The lowest layer of the atmosphere is called the troposphere. This layer is in constant motion. The sun heats Earth’s surface and the air above it, causing warm air to rise. As the warm air rises, air pressure decreases and the air expands and cools. The cool air is denser than the surrounding air, so it sinks and the cycle starts again. This constant cycle of the air driven by the sun’s heat produces weather.
Above the troposphere, starting about 6 to 12 miles (10 to 20 kilometers) above Earth’s surface, is a layer of still air called the stratosphere. The stratosphere includes a region where ultraviolet light from the sun strikes oxygen molecules to create a gas called ozone. This ozone layer blocks most of the sun’s harmful ultraviolet rays from reaching Earth’s surface. Some ultraviolet rays get through, however. They can cause sunburn and skin cancer in people. Traces of human-made chemicals have caused some of the natural ozone to break down.
The mesosphere is the region of Earth’s upper atmosphere in which temperature drops with rising altitude. It lies above the stratosphere. The mesosphere begins at an average altitude of about 30 miles (50 kilometers). It ends at the mesopause, at an altitude of about 50 to 60 miles (80 to 100 kilometers). The decline in air temperature in the mesosphere results from a falling concentration of ozone, which gains heat as it absorbs ultraviolet rays. Temperatures average 32 °F (0 °C) at the base of the mesosphere and about –130 °F (–85 °C) at the mesopause. The lowest temperatures in Earth’s atmosphere occur at the mesopause. Above the poles, summer temperatures at the mesopause can drop below –200 °F (–130 °C).
The uppermost region of Earth’s atmosphere is called the thermosphere. It begins at the mesopause and extends into space. The air in the thermosphere is extremely thin. Nitrogen and oxygen make up most of the lower parts. At about 125 miles (200 kilometers), oxygen becomes more abundant than nitrogen. Above about 600 miles (1000 kilometers), the thermosphere contains mainly hydrogen and helium. Air temperatures in the thermosphere rise with altitude because its upper parts absorb more radiation from the sun than do its lower parts. The temperature rises from about –130 °F (–90 °C) at an altitude of 50 miles (80 kilometers) to more than 2000 °F (1000 °C) at about 200 miles (320 kilometers). But the air in the thermosphere is too thin to transfer much of this heat. For this reason, satellites and spacecraft can operate in the thermosphere without becoming hot.
Solar radiation and cosmic rays ionize (electrically charge) atoms in the upper atmosphere. The region of ionized particles, the ionosphere, extends through parts of the upper mesosphere and lower thermosphere.
Water vapor, carbon dioxide, methane, and other gas es in the atmosphere trap heat from the sun, warming Earth. This heat-trapping process is called the greenhouse effect. Without the greenhouse effect, Earth would probably be too cold for living things.
The hydrosphere.
Earth is the only planet in the solar system with abundant liquid water on its surface. Water has chemical and physical properties not matched by any other substance, and it is essential for life on Earth. Water has a great ability to absorb heat. The oceans store much of the heat Earth gets from the sun. Variation in electrical charge within water molecules gives water an ability to attract atoms from other substances. This quality enables water to dissolve many things. Water’s ability to dissolve materials makes it a powerful agent in breaking down rocks. Liquid water also affects the planet’s interior. The presence of water in rock, for example, lowers the rock’s melting temperature. The water thus makes it easier to melt rock beneath Earth’s surface.
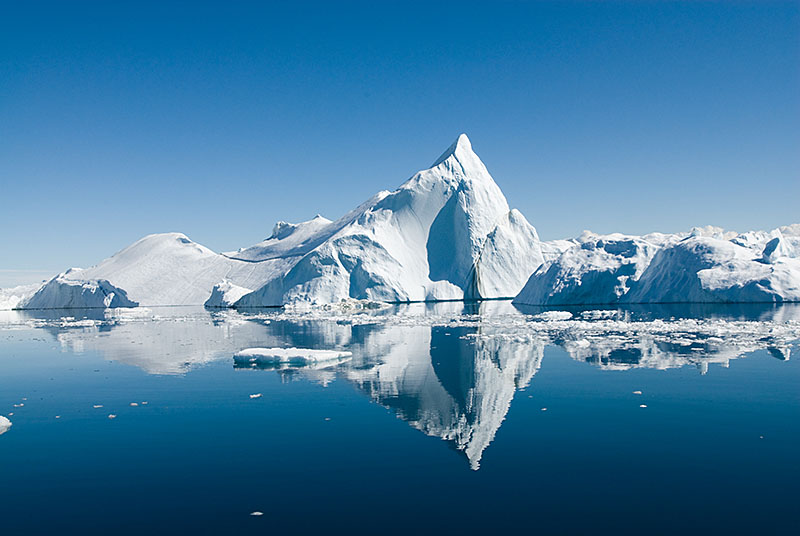
Water covers about 71 percent of Earth’s surface. Most of this water is in the oceans. Ocean water is too salty to drink. Only about 3 percent of Earth’s water is fresh water, water with little salt that is suitable for drinking. Much of Earth’s fresh water is not readily available to people because it is frozen in the polar icecaps or beneath Earth’s surface. Polar regions and high mountains stay cold enough for water to remain permanently frozen. The region on Earth that is covered by snow or ice for at least part of the year is called the cryosphere.
Loading the player...Cryosphere
The lithosphere.
The crust and upper mantle from the surface to about 60 miles (100 kilometers) down make up the lithosphere. The thin crust is made up of rock. The rock consists of various chemicals called minerals. Each mineral is composed of different combinations of elements. Oxygen ranks as the most abundant chemical element in rocks in Earth’s crust. It makes up approximately 47 percent of the mass of rock in the lithosphere. The second most abundant element is silicon, at 28 percent, followed by aluminum (8 percent), iron (5 percent), calcium (4 percent), and sodium, potassium, and magnesium (about 2 percent each). These eight elements make up nearly 99 percent of the mass of rocks on Earth’s surface.
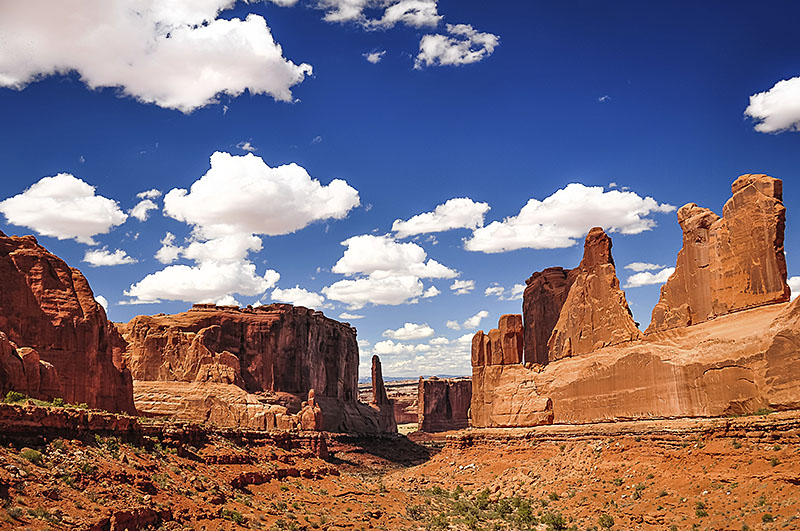
Two elements, silicon and oxygen, make up almost three-fourths of the crust. This combination of elements is so important that geologists have a special term for it: silica. Minerals that contain silica are called silicate minerals. One of the most abundant minerals on Earth’s surface is quartz, made up of pure silica. Another plentiful group of silicates are the feldspars, which consist of silica, aluminum, calcium, sodium, and potassium. Other common silicate minerals on Earth’s surface are pyroxene (PY rahk seen) and amphibole (AM fuh bohl), combinations of silica, iron, and magnesium.
Another important group of minerals is the carbonates, which contain carbon and oxygen along with other elements. The most important carbonate mineral is calcite, made up of calcium, carbon, and oxygen. Limestone, a common rock used for building, is mostly calcite. Another important carbonate mineral is dolomite, composed of carbon, oxygen, calcium, and magnesium.
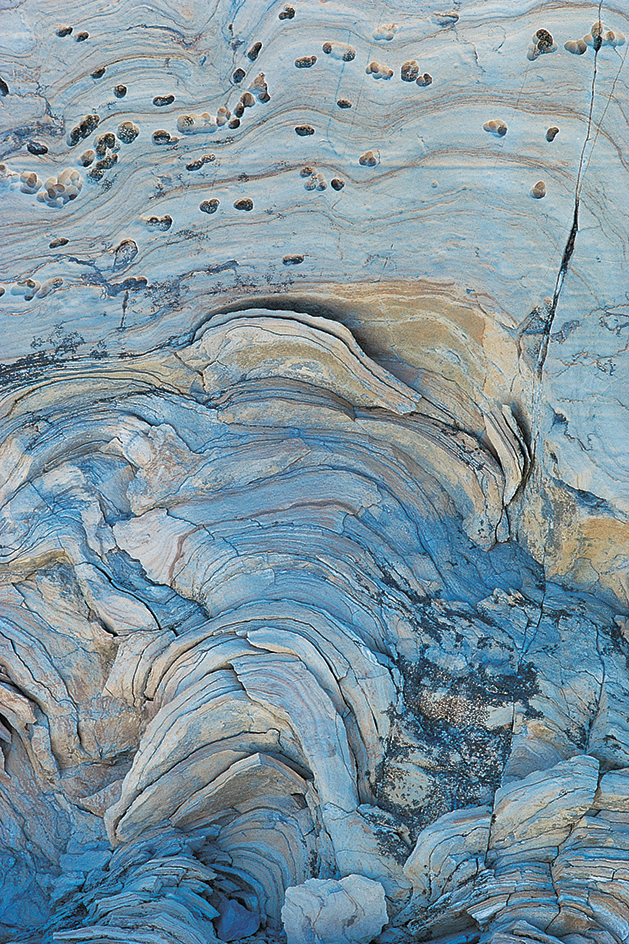
Earth has two kinds of crust. The dry land of the continents consists mostly of granite and other light silicate minerals. The ocean floors, on the other hand, are composed mostly of a dark, dense volcanic rock called basalt. Continental crust averages about 25 miles (40 kilometers) thick. But it is thicker in some areas and thinner in others. Most oceanic crust is only about 5 miles (8 kilometers) thick. Water fills in the low areas over the thin basaltic crust to form the world’s oceans. There is more than enough water on Earth to completely fill the oceanic basins, and some of it spreads onto the continents’ edges. The portion of the continents covered by a band of shallow ocean is called the continental shelf.
The biosphere.
The region containing life extends from the bottom of the deepest ocean to a few miles or kilometers into the atmosphere. There are several million known species (kinds) of living things, and scientists believe that there are a great many more species not yet discovered.
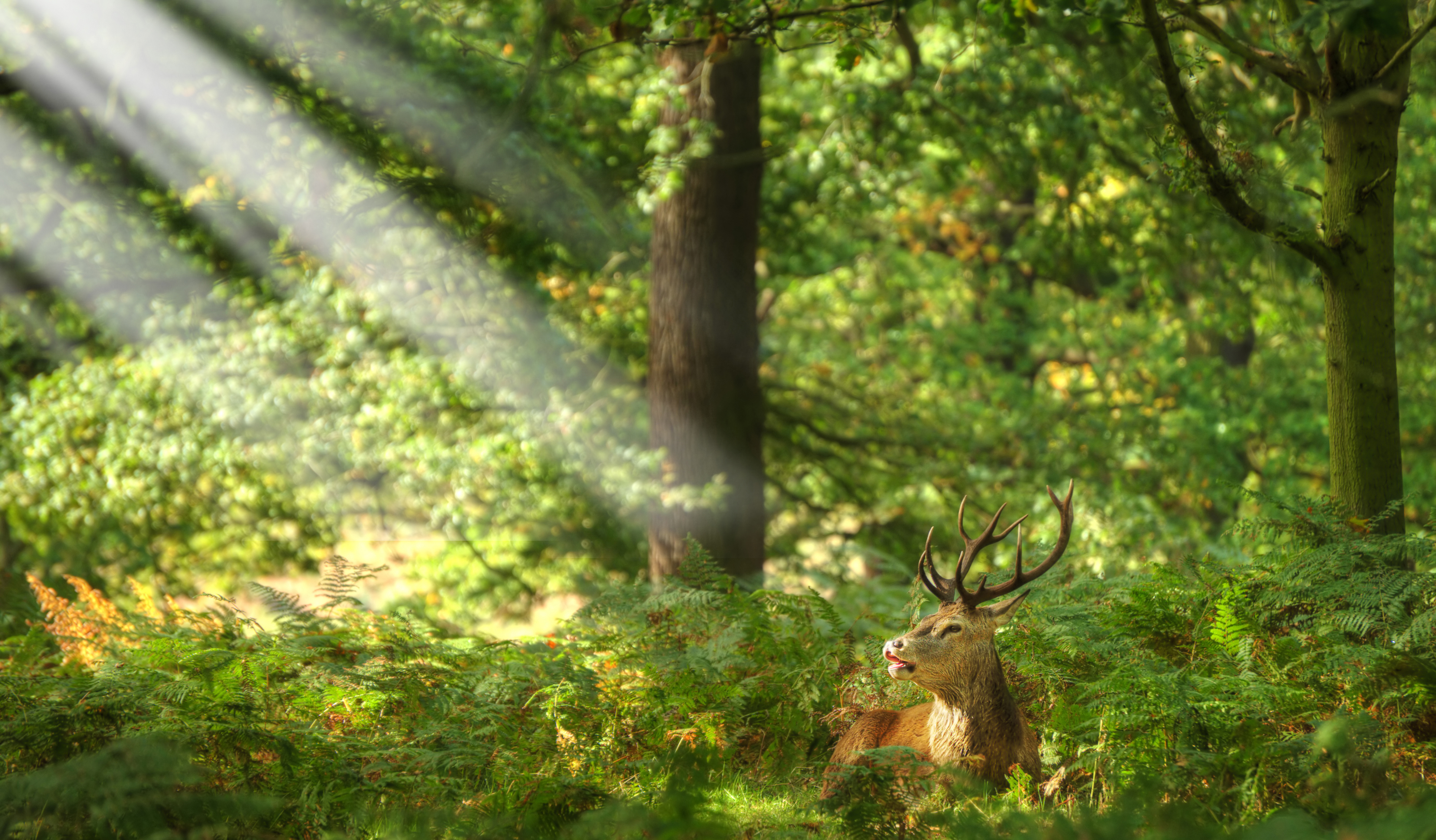
Life affects Earth in many ways. For example, algae and plants are responsible for producing almost all the oxygen gas in the atmosphere. These organisms take in water and carbon dioxide, both of which contain oxygen. They use the energy of sunlight to combine the carbon in carbon dioxide and the hydrogen in water, making chemicals of many kinds. This process, called photosynthesis, gives off oxygen as a waste product. Plants and algae are also eaten by animals to get energy. The animals thus return water and carbon dioxide to the environment through digestion and respiration.
Living things affect the surface of Earth in other ways as well. As plants decompose, they create chemicals that speed the breakdown of rock. Grassland and forest plants also slow the erosion of soil. Erosion is a natural process by which rock and soil are broken loose at one location and moved to another. In addition, human activities have drastically reshaped parts of the planet.
Earth’s rocks
The solid part of Earth consists of rocks. Rocks are sometimes made up of a single mineral, but more often they consist of mixtures of minerals. Geologists classify rocks according to origin. Igneous rocks form when molten rock cools and solidifies. Sedimentary rocks form when grains of rock or dissolved chemicals are deposited in layers by wind, water, or glaciers. Over time, the layers harden into solid rock. Metamorphic rocks develop deep in Earth’s crust when heat and pressure transform other types of rock.
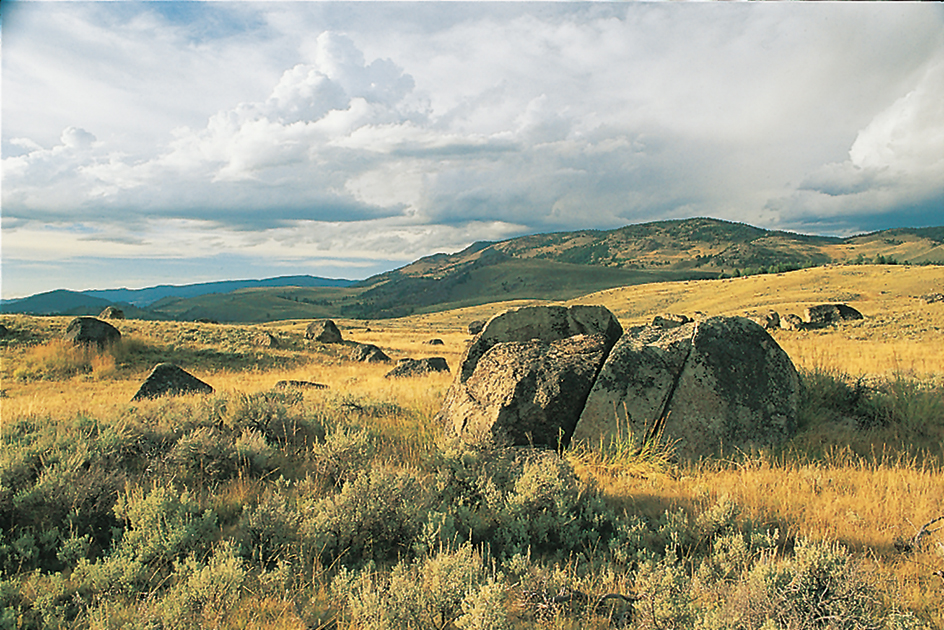
Igneous rocks
form from molten material called magma. Most of Earth’s interior is solid, not molten, but it is extremely hot. At the base of Earth’s crust, the temperature is about 1800 °F (1000 °C). In some portions of the crust, conditions are right for rocks to melt. Rocks can melt more easily near the crust if they contain water, which lowers their melting point.
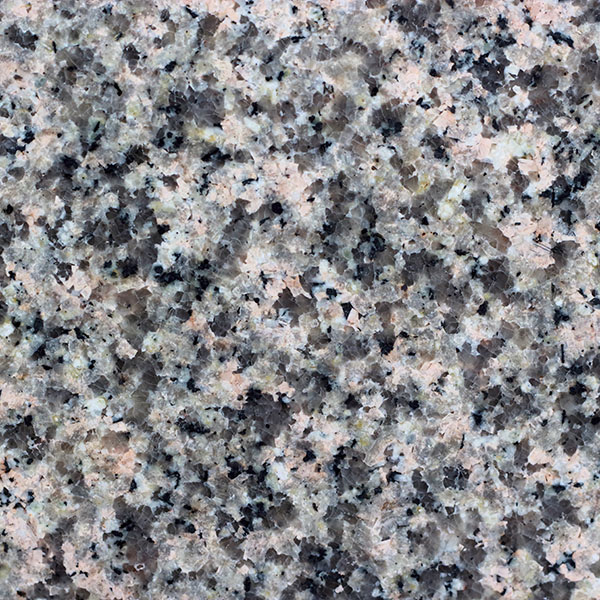
Where conditions are right, small pockets of magma form beneath and within the crust. Some of this magma reaches the surface, where it may erupt from volcanoes as lava. Igneous rocks formed from the cooling lava are called volcanic or extrusive rocks. Vast quantities of magma, however, never reach the surface. They cool slowly within the crust and may only be exposed long afterward by erosion. Such igneous rocks are called plutonic or intrusive rocks. The relatively slow cooling of plutonic rocks enables their minerals to form large crystals. Because they have larger crystals, plutonic rocks tend to have a coarser texture than volcanic rocks.
Igneous rocks that are rich in silica tend to be poor in iron and magnesium, and vice versa. Volcanic rocks that are iron-rich and silica-poor are basalt. Plutonic rocks of the same makeup are called gabbro. Silica-rich volcanic rocks are called rhyolite << RY uh lyt >>, and plutonic rocks of the same composition are granite. Granite lies under most of the continents, while basalt lies under most of the ocean floors.
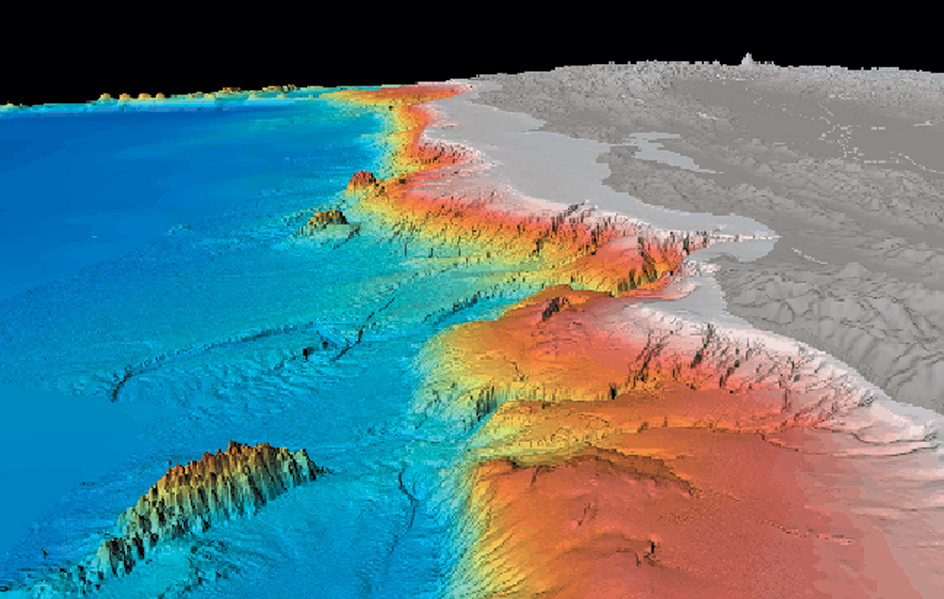
Sedimentary rocks.
Rocks on Earth’s surface are under constant wear by chemicals and by mechanical forces. The processes that break down rocks are called weathering. Water is effective at dissolving minerals, weakening the rock. When water freezes, it expands. The expanding ice helps pry apart mineral grains in rocks. In addition, living things make chemicals that help dissolve rocks, and plant roots can grow into rock, slowly prying it apart.
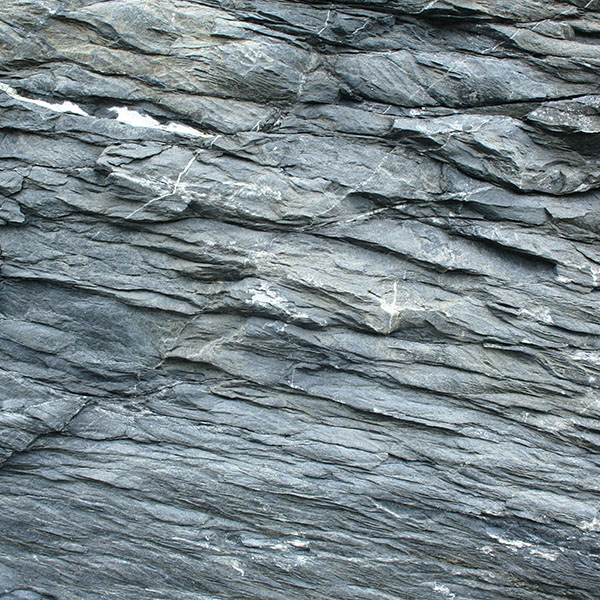
Once rocks break apart, the loose material is often carried away by erosion. Running water erodes rocks. Wind and glaciers also contribute to erosion. Erosion is usually a relatively slow process, but it can happen at different rates in different areas. For example, active mountain ranges such as the Himalaya may erode much faster than the flat interior of Australia. Over millions of years, erosion can uncover rocks originating many miles or kilometers below the surface.
Materials derived from the weathering and erosion of rocks are eventually deposited to form sedimentary rocks. Rocks that are made up of small pieces of other rocks are called clastic sedimentary rocks. Rocks containing larger pebbles are called conglomerates. The particles in these conglomerate rocks are cemented together when minerals dissolved in water crystallize between the grains. The most abundant sedimentary rocks, called mudrocks, consist of tiny particles. Some of these rocks, called shale, split into thin sheets when broken. Sandstone is a sedimentary rock made up of grains of sand cemented together.
Other sedimentary rocks form when dissolved materials undergo chemical reactions and settle out as tiny solid particles. These rocks are called chemical sedimentary rocks. Common chemical sedimentary rocks include some types of limestone and dolomite. Some chemical sedimentary rocks form when water evaporates, leaving dissolved materials behind. Rock salt and gypsum, a calcium and sulfur mineral, form this way.
Some sedimentary rocks, called biogenic rocks, are formed by the action of living things. Coal is the remains of woody plants that have been transformed into rock by heat and pressure over time. Most limestone is formed by microscopic marine organisms that secrete protective shells of calcium carbonate. After the animals die, the shells remain and solidify into limestone.
Metamorphic rocks.
When rocks are buried deeply, they become hot. Earth’s crust grows hotter by about 70 Fahrenheit degrees per mile (25 Celsius degrees per kilometer) of depth. Pressure also increases with depth. At a depth of 1 mile (1.6 kilometers), the pressure is about 6,000 pounds per square inch (40,000 kilopascals). As rocks are heated and subjected to pressure, minerals react in a process called metamorphism. Shale is transformed to slate, limestone, and eventually marble under pressure. Many metamorphic rocks contain recognizable features that reveal their origin. But others change so much that only their chemical makeup provides evidence of the original rock.
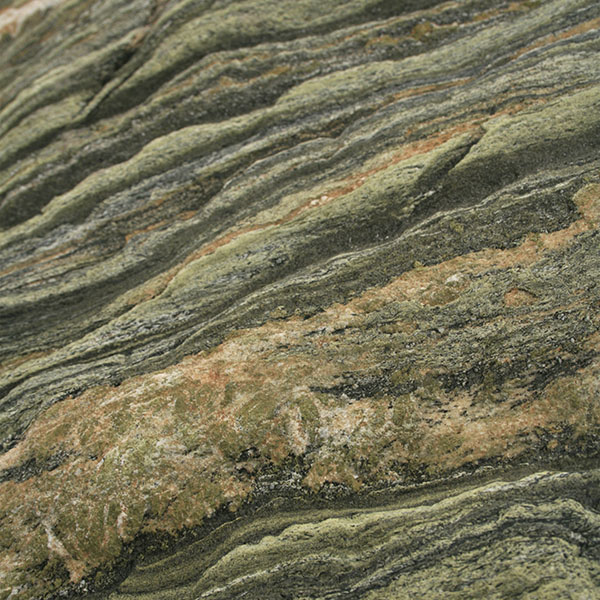
Earth’s crust
The hot rock deep in Earth’s mantle flows upward slowly, while cooler, denser rock near the surface sinks. This rising and sinking motion driven by temperature differences is a form of convection. As the mantle flows, it breaks the crust and the rigid upper mantle into a number of large slabs called tectonic plates. The slow flow of Earth’s mantle drags the plates along, causing the continents to move, mountains to form, and volcanoes and earthquakes to occur. This constant motion of Earth’s outer shell is called plate tectonics.
In some places, usually under the oceans, Earth’s plates are spreading apart. New magma from the mantle rises to fill the cracks between the plates. Places where plates spread apart are called spreading centers. Many volcanoes occur where plates pull apart and magma wells up from within the mantle to fill the gap. The material from the mantle consists of iron and magnesium-rich silicate rock. It hardens to form basalt, creating new oceanic crust.
Subduction.
Earth’s crust cannot spread apart everywhere. Somewhere, an equal amount of crust must be removed. When two plates push together, one of the plates sinks back into the mantle in a process called subduction. The sinking plate eventually melts into magma in Earth’s interior. Much of the magma created in subduction zones does not reach the surface. It cools within the crust, forming plutonic rocks. The heat from the magma also helps create metamorphic rocks.
Continental crust is too thick and not dense enough to sink into Earth’s interior, so only plates of the denser oceanic crust are subducted. The boundary where the two plates meet is marked by a deep trench on the ocean floor. Such trenches are the deepest places in the oceans, reaching up to 36,000 feet (11,000 meters) deep.
The upper plate that remains on the surface may be continental crust or oceanic crust. This plate is also changed by subduction. As the two plates move together, the edge of the upper plate is compressed. The crust becomes thicker and higher, creating a mountain range. When the rocks of the sinking plate reach a depth of about 60 to 90 miles (100 to 150 kilometers), they begin to melt and form magma. Some of the magma reaches the surface to form volcanoes. Regions with many volcanoes, such as Peru, Japan, and the northwestern United States, lie near areas where subduction is happening.
Mountain building.
Occasionally as a plate sinks into Earth’s mantle, it drags along a continent or a smaller land mass. Continental crust is too thick and light to sink. Instead, it collides with the opposing plate. If the opposing plate is also a continent, neither plate will sink. This type of collision often forms a vast mountain chain in the middle of a continent. The Himalaya were formed in this way from the collision of two plates of continental crust.
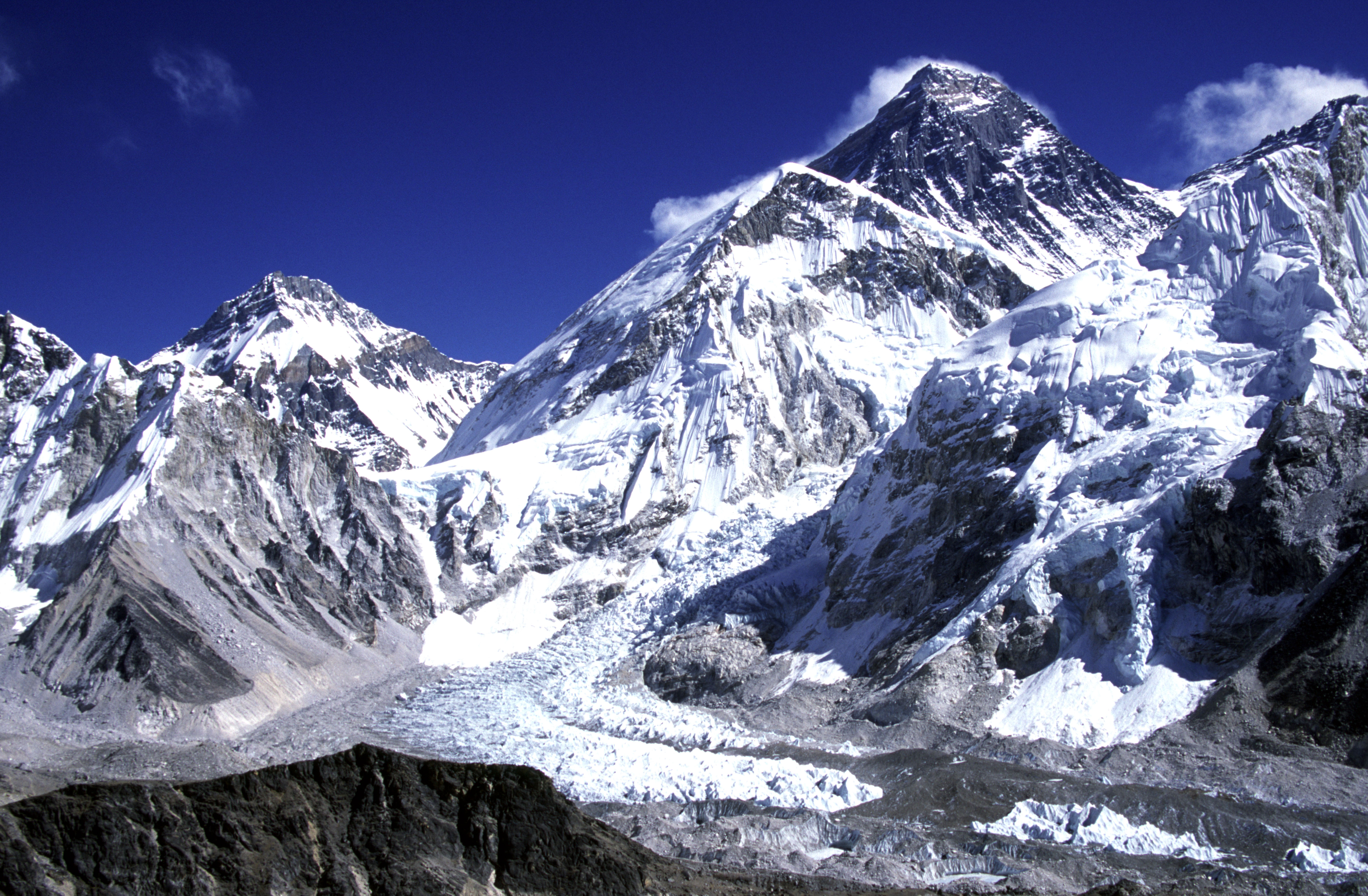
The series of events that happen during formation of a mountain range is called orogeny. Orogeny includes the lifting of mountains, the folding and crumpling of the rocks, volcanic activity, and the formation of plutonic and metamorphic rocks. Long after mountains have vanished due to erosion, geologists can still see evidence of orogeny.
Terrane collisions.
Smaller pieces of continental crust that collide with another plate are often added to the edge of the larger plate. These added pieces of crust are called terranes. Most of the land in the United States west of Salt Lake City has been added to North America by terrane collisions in the last 500 million years.
Earthquakes.
Earthquakes occur when rocks on opposite sides of a break in the crust, called a fault, slide past each other. The boundaries between plates are faults, but there are faults within plates as well. Such faults occur because forces within the plates cause rocks to fracture and slip, even distant from plate boundaries. The boundaries between two plates sliding past each other are called transform faults. The San Andreas Fault in California is a transform fault. There, a portion of crust called the Pacific Plate is carrying a small piece of California northwest past the rest of North America.
The shaping of the continents.
Several times in Earth’s history, collisions between continents have created a single huge supercontinent. Although the crust of the continents is thick, it breaks more easily than oceanic crust. Supercontinents broke quickly into smaller pieces. Material from Earth’s mantle filled the gaps, creating new oceanic crust. As the continents moved apart, new ocean basins formed between them. About one third of Earth’s surface is covered by continental crust, so the pieces cannot move far before colliding. As two continents collide, an old ocean basin is destroyed. The process of continents breaking apart and rejoining is called the Wilson cycle. The name comes from the Canadian geologist John Tuzo Wilson, who first described it.
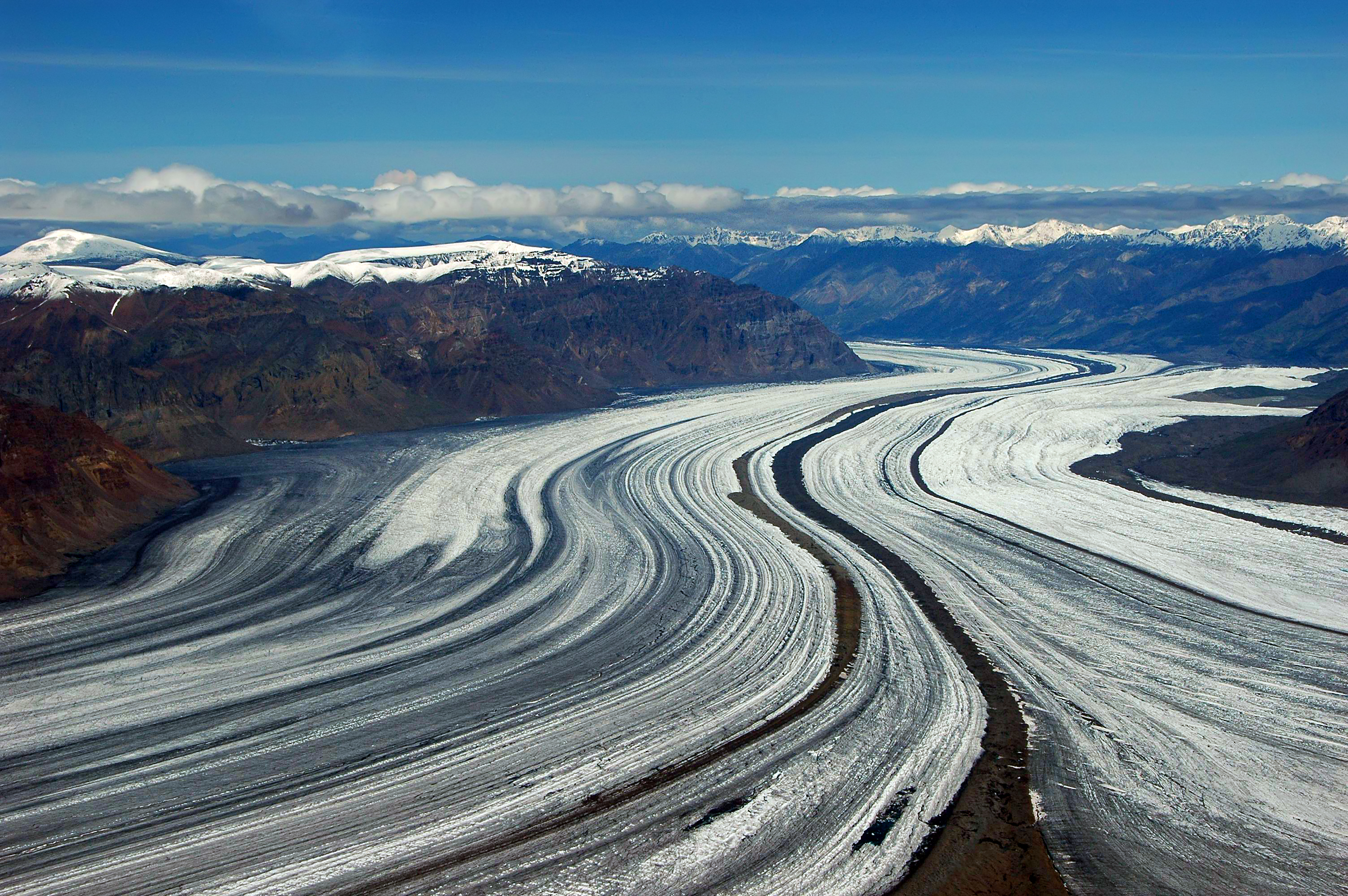
The continents have been in motion for at least the past 3.8 billion years. Geologists only have enough evidence from rocks, however, to understand and reconstruct the continents’ motion over the past 800 million years. Most of the oceanic crust older than that was subducted into the mantle long ago.
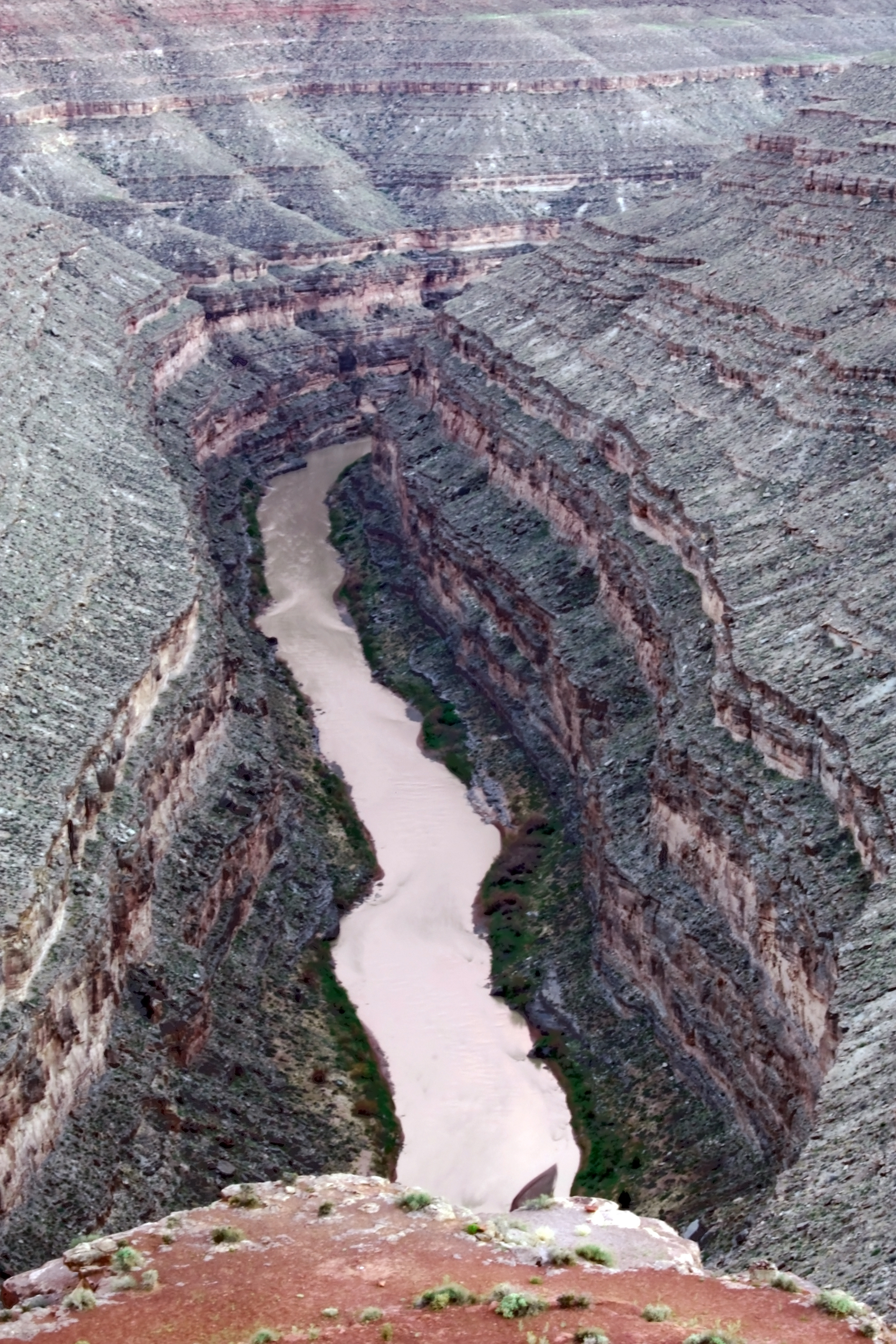
Geologists have determined that the continents were assembled into a large supercontinent called Rodinia about 800 million years ago. What is now North America lay at Rodinia’s center. The flow of material in Earth’s mantle caused Rodinia to break apart into many pieces, which collided again between 500 million and 250 million years ago. Collisions among what are now North America, Europe, and Africa caused the uplift of the Appalachian Mountains. Collisions between parts of what are now Siberia and Europe created the Ural Mountains.
By 250 million years ago, the continents reassembled to form another supercontinent called Pangaea. A single worldwide ocean, called Panthalassa, surrounded Pangaea. About 200 million years ago, Pangaea began to break apart. It split into two large land masses called Gondwanaland and Laurasia. Gondwanaland then broke apart, forming the continents of Africa, Antarctica, Australia, and South America and the Indian subcontinent. Laurasia eventually split apart into Eurasia and North America. As the continental plates split and drifted apart, new oceanic crust formed between them. The movement of the continents to their present positions took place over tens of millions of years.
Earth’s interior
Geologists cannot study Earth’s interior directly. The deepest wells drilled reach less than 9 miles (14 kilometers) below the surface. Geologists know that the whole Earth differs in composition from its thin outer crust. Deep in Earth, pressures are so great that minerals can be compressed into forms not found on the surface.
One way geologists determine the overall composition of Earth is from chemical analysis of meteorites. Certain types of meteorites, called chondrites, are remains from the early solar system that stayed relatively unchanged in space until they fell to Earth. Geologists can use chondrites to estimate the original chemical composition of the entire Earth.
Unlike chondrites, Earth consists of layers that contain different amounts of various chemical elements. Geologists learn about the structure of Earth’s interior by studying vibrations generated by earthquakes, called seismic waves. Geologists record such waves using instruments called seismographs. The speed and motion of vibrations traveling through Earth depend on the composition and density of the material the vibrations travel through. Geologists can thus determine many properties of Earth’s interior by analyzing such vibrations.
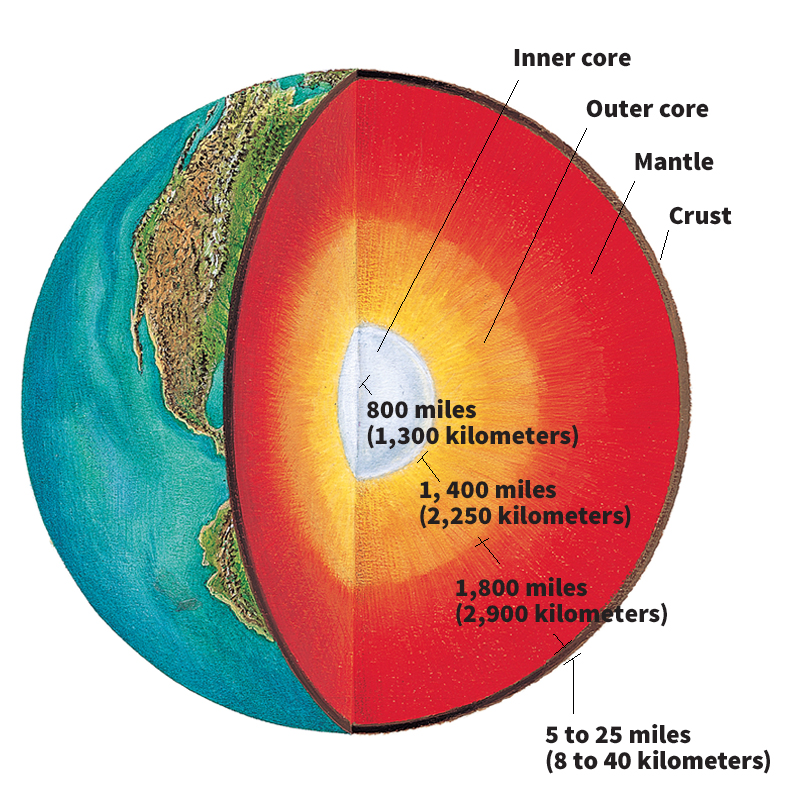
The mantle.
Beneath the crust, extending down about 1,800 miles (2,900 kilometers), is a layer called the mantle. Most of the mantle is not rigid but flows extremely slowly. Earth’s crust floats on the mantle much as a board floats in water. Just as a thick board would rise above the water higher than a thin one, the thick continental crust rises higher than the thin oceanic crust. The slow motion of rock in the mantle moves the continents around and causes earthquakes, volcanoes, and the formation of mountain ranges.
Loading the player...Drifting continents
The core.
At the center of Earth is the core. The core is made mostly of iron and nickel and possibly smaller amounts of lighter elements, including sulfur, oxygen, and silicon. The core measures about 4,300 miles (7,000 kilometers) in diameter, slightly larger than half the diameter of Earth and about the size of Mars. The outermost 1,400 miles (2,300 kilometers) of the core is liquid.
Motions within the liquid core are thought to generate Earth’s magnetic field, the region of magnetic influence surrounding the planet. The field is responsible for several effects. For example, magnetic compasses make use of the field to determine the direction of magnetic north. The magnetic field is also responsible for the northern lights, called the aurora borealis, and the southern lights, aurora australis. Most importantly, the field protects life on Earth from cosmic rays, electrically charged, high-energy particles that travel through space. These particles can damage the cells of living things. But the magnetic field deflects almost all of them.
Geologists believe the innermost part of the core, about 1,500 miles (2,400 kilometers) in diameter, consists of a similar material to that of the outer core, but the inner core is solid. The inner core is about three-fourths as big as Earth’s moon.
Earth gets hotter toward the center. At the bottom of the continental crust, the temperature is about 1800 °F (1000 °C). The temperature increases about 3 Fahrenheit degrees per mile (1 Celsius degree per kilometer) below the crust. Geologists believe the temperature of Earth’s outer core is about 6700 to 12,000 °F (3700 to 6500 °C). The inner core may be as hot as 16,000 °F (9000 °C)—hotter than the surface of the sun. Because it is under great pressures, however, the center of Earth remains solid.
Cycles on and in Earth
Earth can be thought of in terms of a huge system of interacting cycles. In each cycle, matter and energy move around and may change form. Eventually, matter may return to its original condition, and the cycle begins again. Such cycles affect everything on the planet, from the weather to the landscape. There are many cycles on and within Earth. A few of the most important are: atmospheric circulation; ocean currents; the global heat conveyor; the hydrologic cycle, or water cycle; the rock cycle; the nutrient cycle—which includes the nitrogen and phosphorus cycles; and the carbon cycle.
Loading the player...The carbon cycle
Atmospheric circulation.
Near the equator, air warmed by the sun rises. This air flows toward Earth’s poles, cooling and eventually returning to the surface. It then flows back to the equator. This motion, combined with the rotation of Earth, moves heat and moisture around the planet, creating winds and weather patterns.
In some areas, the winds change directions with the seasons. Such seasonal weather patterns are often called monsoons. In summer, for example, air over Asia is heated by the sun. It rises and draws moist air from the Indian Ocean, causing daily rains over most of southern Asia. In winter, the air over Asia cools, sinks, and flows out, pushing the moist air away and creating dry weather. A similar pattern occurs in the Pacific Ocean near Mexico, bringing moist air and afternoon thunderstorms to the southwestern United States in the summer.
Ocean currents
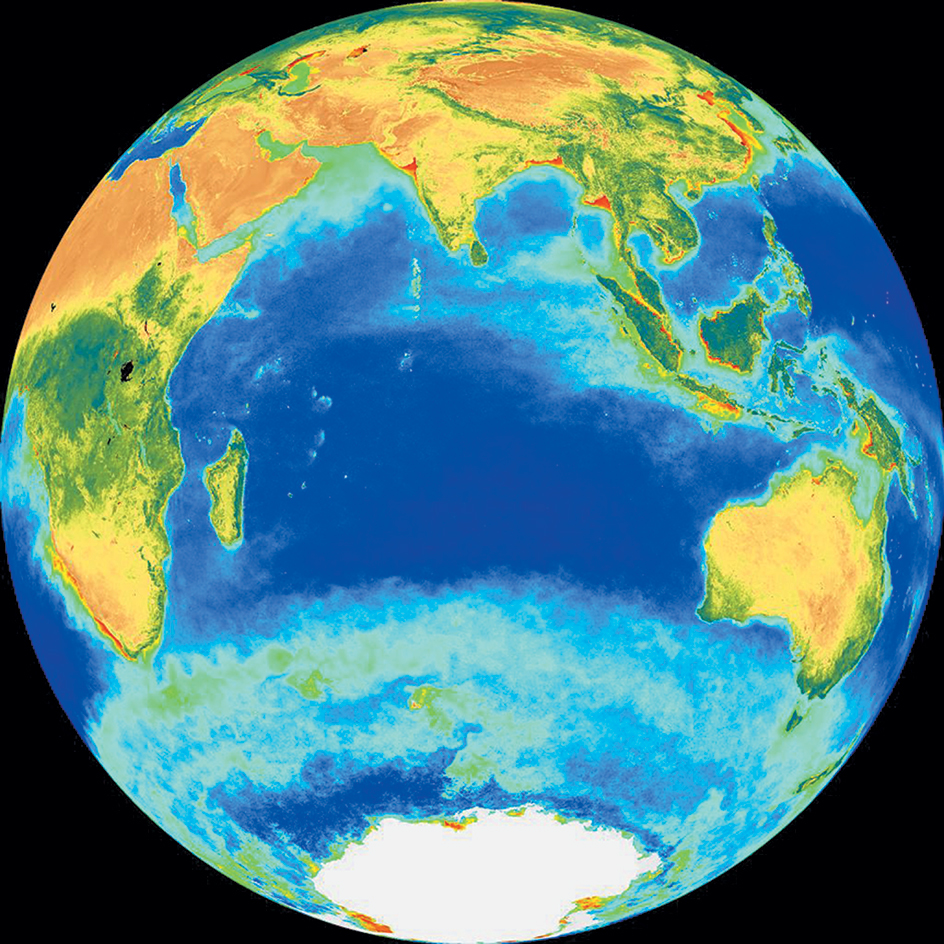
are driven by the winds and follow similar patterns. The flow of the currents, however, is blocked by continents. Ocean currents flow west near the equator, then turn toward the poles when they strike a continent. They turn east, then flow back to the equator on the other side. In all the oceans, the currents form great loops called gyres. The gyres flow clockwise north of the equator and counterclockwise south of it.
The global heat conveyor
is an enormous circulation of ocean water that distributes the oceans’ heat around Earth. Water in the polar regions is very cold, salty, and dense. It sinks and flows along the sea floor toward the equator. Eventually, the water rises along the margins of the continents and merges with the surface water flow. When it reaches the polar regions, it sinks again. This movement of water mixes heat throughout the oceans, warming polar waters. It also brings nutrients from the deep ocean to the surface, where they can be used by marine plants and animals.
The water cycle.
Water from the oceans evaporates and is carried by the atmosphere, eventually falling as rain or snow. Water that falls on the land helps break rocks down chemically, nourishes plants, and wears down the landscape. Eventually, the water returns to the sea to start the cycle over again.
The rock cycle.
Earth has a great diversity of rocks compared to other planets because so many processes act to form and break down rocks on Earth. Geologists sometimes speak of the “rock cycle” to explain how the basic rock types—igneous, metamorphic, and sedimentary—are related. The cycle may begin with a flow of lava from a volcano cooling to form new igneous rocks on Earth’s surface. As the rock is exposed to water, it breaks down and the resulting materials may be carried away to be deposited as sedimentary rocks. These rocks may be come so deeply buried that they change into metamorphic rocks. They may even melt, creating the raw material for the next generation of igneous rocks.
Rocks rarely go through the entire rock cycle. Instead, some steps may be skipped or repeated. For example, igneous rocks can be subjected to heat and pressure and transformed directly into metamorphic rocks. Sedimentary rocks can be broken down by weathering and then reassembled into a new generation of sedimentary rocks. Metamorphic rocks can also be weathered to form the raw material for a new generation of sedimentary rocks. Any basic rock type, igneous, metamorphic, or sedimentary, can be transformed into any other type.
The nitrogen cycle
consists of the chemical changes the element nitrogen goes through around Earth’s surface. All known living things need nitrogen to survive. Earth’s atmosphere contains about 78 percent nitrogen gas (N2). However, most living things cannot use pure nitrogen as a nutrient. It must first be converted into one of several nitrogen-containing chemicals.
Certain bacteria in the soil convert nitrogen gas to ammonia (NH3) in a process called nitrogen fixing. Other bacteria then take the ammonia and convert it to nitrate (NO3 –) or nitrite (NO2 –), depending on the bacteria. This process is called nitrification. Plants are able to grow using the nitrogen in ammonium, nitrates, and nitrites. The usable forms of nitrogen pass up the food chain as animals eat these plants or eat other animals. Other bacteria replenish nitrogen in the atmosphere by converting nitrogen compounds back into nitrogen gas, a process called denitrification. Lightning strikes also split nitrogen gas, making it available to plants.
The phosphorus cycle
consists of chemical changes undergone by the element phosphorus around Earth’s surface. Unlike nitrogen, phosphorus is almost never found in its pure form. Instead, it is locked in a chemical compound with oxygen atoms, called a phosphate. The most common phosphate is orthophosphate (PO4 3-). The largest natural source of phosphate comes from the erosion of rocks. As rocks with phosphate dissolve in water, phosphate becomes available for plants to absorb. The phosphate can then pass on to animals that feed on the plants. The phosphate may also settle on the ocean floor to be converted back into phosphate rocks.
The carbon cycle.
The movement and chemical changes undergone by the element carbon have significant effects on Earth’s climate and living things. Carbon serves as the basic building block of life on Earth. It is also found in fossil fuels such as coal, natural gas, and oil. Some carbon is dissolved in the oceans. Most carbon is locked in rocks called carbonate rocks.
A small percentage of Earth’s atmosphere is carbon dioxide gas (CO2), an important greenhouse gas. Plants use CO2 to grow, taking it from the atmosphere. Plants and animals that die and settle into the soil or ocean bottom effectively remove carbon from the cycle in a process called sequestration. Carbon dioxide is replenished by the erosion of carbonates and by volcanic eruptions.
When mountains rich in silicate minerals wear down through weathering and erosion, calcium and magnesium erode from the rocks. These elements are carried to the sea by water. There, living organisms absorb the chemicals and use them to make protective carbonate shells. The organisms eventually die and sink to the bottom to form limestone deposits. This process, called the carbonate-silicate cycle, removes carbon dioxide from the atmosphere. With less carbon dioxide in the atmosphere to trap heat from the sun, Earth’s climate may cool enough to cause an ice age.
Limestone and dolomite deposits exposed to weathering and erosion, on the other hand, return carbon dioxide to the atmosphere. Subduction can also carry some limestone on the ocean floor down into the mantle. Beneath the crust, the limestone breaks down into magma under heat and pressure. The carbon dioxide in the limestone can then return to the atmosphere via volcanic eruptions.
Since at least the early 1800’s, human activities have altered the carbon cycle. The rapid burning of fossil fuels and of wood for energy has introduced a large amount of CO2 into the atmosphere. The added CO2 traps more heat near Earth’s surface, greatly contributing to the greenhouse effect.
Increasing the concentration of CO2 gas also increases the amount of carbon and of heat absorbed by the oceans. As more CO2 dissolves into the oceans, the waters become more acidic. The increased acidity has been shown to have a negative effect on some ocean life. For example, many shell-producing organisms, such as mollusks and corals, may not be able to grow strong shells in more acidic waters. The additional heat has been shown to alter the habitats and behaviors of certain fish and corals.
History of Earth
Earth’s history is recorded in the rocks of the planet’s crust. Rocks have been forming, wearing away, and re-forming since Earth took shape. The products of weathering and erosion, called sediments, accumulate in layers known as strata. Strata preserve clues about Earth’s past. Geologists study Earth’s past by analyzing the composition of the sediment, the way the strata are deposited, and the kinds of fossils that may occur in the rock.
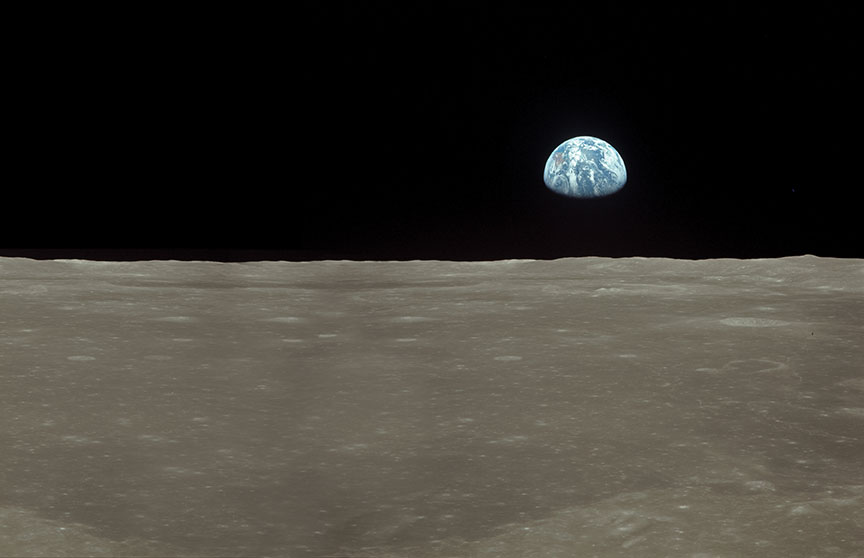
Space exploration has also expanded our understanding of Earth’s origin. The Hubble Space Telescope, for example, has observed stars that appear to be in the process of forming planets. Since the mid-1990’s, scientists have identified distant stars with their own planets. These discoveries have helped scientists develop theories about the formation of Earth.
Age.
Scientists think that Earth probably formed at about the same time as the rest of the solar system. They have determined that some chondrite meteorites, relatively unaltered remains from the formation of the solar system, are up to 4.6 billion years old. Scientists believe that Earth and the other planets are probably that old. They can determine the ages of rocks by measuring the amounts of natural radioactive materials in them. Radioactive elements decay (change into other elements) at a known rate. For example, uranium gives off radiation and decays into lead. Scientists know the average time it takes for an atom of uranium to change into an atom of lead. Thus, they can find the age of a rock by comparing the amount of uranium to the amount of lead in it.
Geologists divide Earth’s known history into four long stretches of time called eons. Starting with the earliest, the eons are the Hadean, Archean, Proterozoic, and Phanerozoic. The first three eons, which together lasted about 4 billion years, are called the Precambrian times. The Phanerozoic Eon, when life became abundant, is divided into three eras. They are, from the earliest to the latest, the Paleozoic, Mesozoic, and Cenozoic eras. Eras are divided into periods, and periods are divided into epochs. These divisions are named for places where rocks of each period were studied. Periods are mostly separated by important changes in the types of fossils found in the rocks. As a result, the lengths of the various eras, periods, and epochs are not equal.
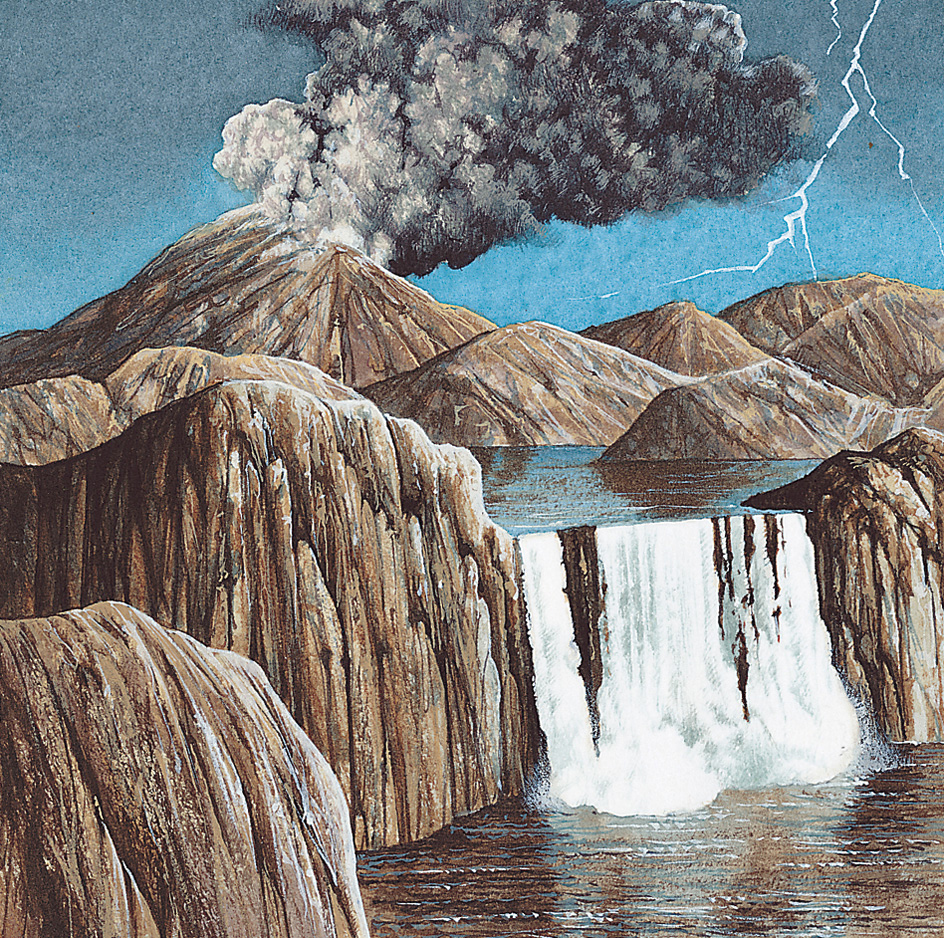
A chart showing an outline of Earth’s history is called a geological time scale. On such a chart, Earth’s earliest history is often at the bottom, and its recent history at the top. This organization mimics the basic arrangement of rock strata, with new strata forming atop old ones.
Formation.
Most scientists think that the solar system began as a thin cloud of gas and dust in space. The sun itself may have formed from a portion of the cloud that was thicker than the rest. The cloud’s own gravity caused it to start contracting, and dust and gas were drawn in toward the center. Much of the cloud collapsed to the center to form a star, the sun, but a great ring of material remained orbiting around the star. Particles in the ring collided to make larger objects, which in turn collided to build up the planets of the solar system in a process called accretion. Scientists believe that many small planetlike objects formed and then collided to make larger planets.
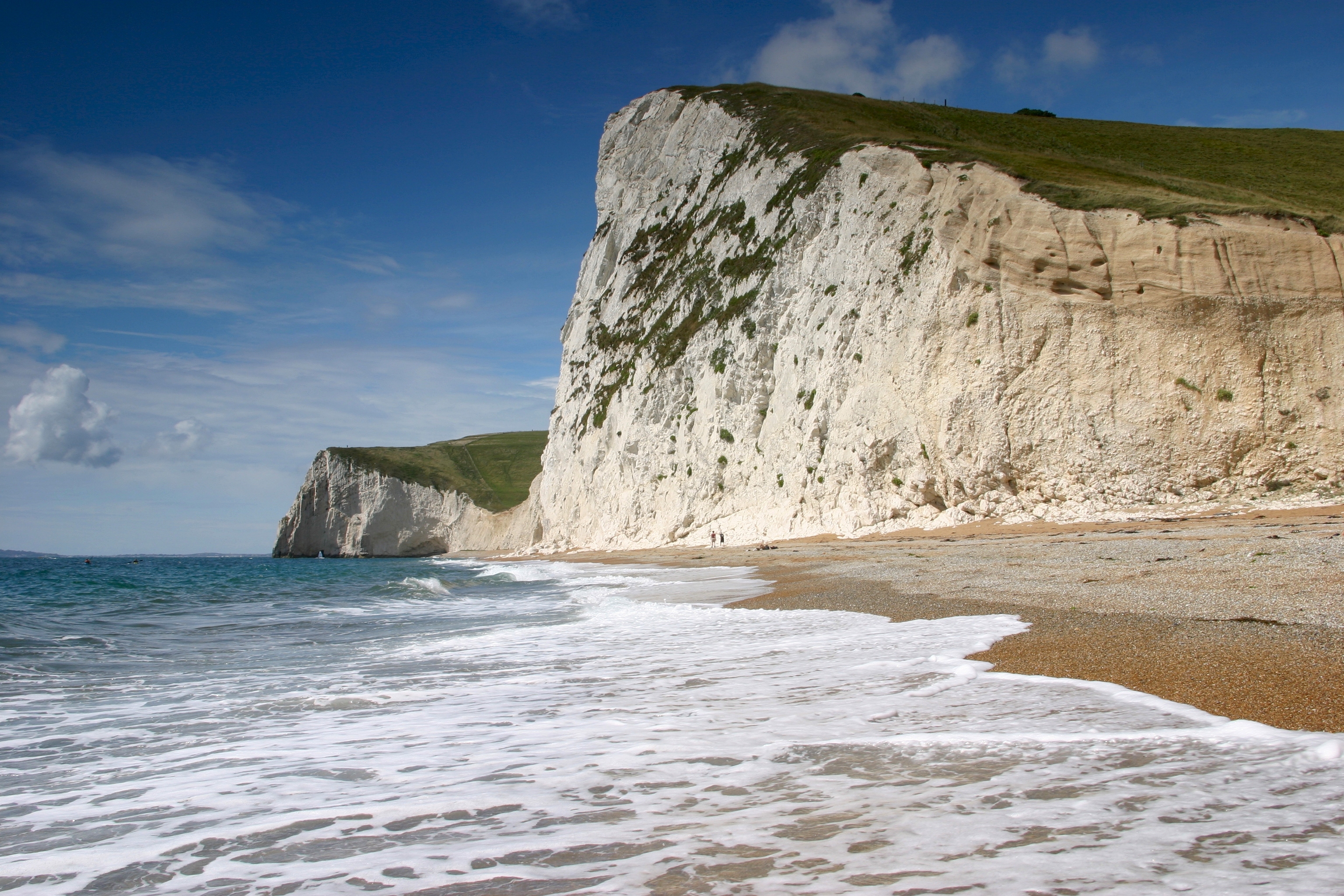
Early development.
Scientists theorize that Earth began as a waterless mass of rock surrounded by a cloud of gas. Radioactive materials in the rock and increasing pressure in Earth’s interior produced enough heat to melt the interior. The heavy materials, such as iron, sank. The light silicate rocks rose to Earth’s surface and formed the earliest crust. The heat of the interior caused other chemicals inside Earth to rise to the surface. Some of these chemicals formed water, and others became the gases of the atmosphere.
Scientists have identified a crystal of the mineral zircon that is about 4.4 billion years old. Through chemical analysis, they have determined that liquid water probably existed on Earth’s surface when the crystal was formed. They concluded that Earth’s crust and oceans may have formed within about 200 million years after the planet took shape.
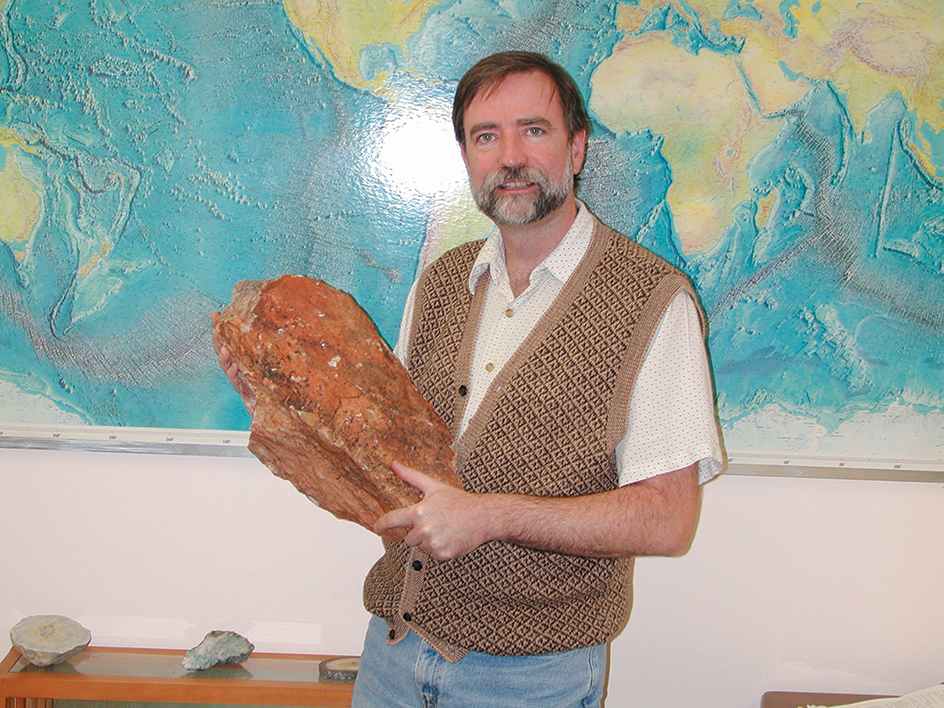
Astronomers believe that the sun was about 30 percent fainter when Earth first formed than it is today. But mineral studies show that Earth was warm enough for liquid water to exist. The early atmosphere must have trapped more heat from the sun than today. Over millions of years, water slowly collected in low places of the crust and formed oceans.
The newly formed planets swept up most of the remaining debris from the early solar system. During this time, the planets and debris underwent frequent, violent collisions. The impacts created the cratered surfaces of Mars, Venus, and Mercury, in addition to that of the moon. Earth was also struck, but the craters produced have mostly been erased by erosion and plate tectonics. Geologists have identified evidence that tectonic activity has occurred for at least 3.8 billion years.
Some scientists believe that Earth’s early atmosphere contained hydrogen, helium, methane, and ammonia, much like the present atmosphere of Jupiter. Others believe it may have contained a large amount of carbon dioxide, as does the atmosphere of Venus. Scientists agree that Earth’s earliest atmosphere had little oxygen.
About 2 billion years ago, a change in Earth’s atmosphere occurred. Geologists know this because certain kinds of iron ores that develop in oxygen-poor environments stopped forming at that time. Instead, large deposits of red sandstone formed. The red color results from iron reacting with oxygen to form iron oxide, or rust. The sandstone deposits serve as evidence that Earth’s atmosphere contained some oxygen. The air would not have been breathable, but the atmosphere may have had about 1 percent oxygen.
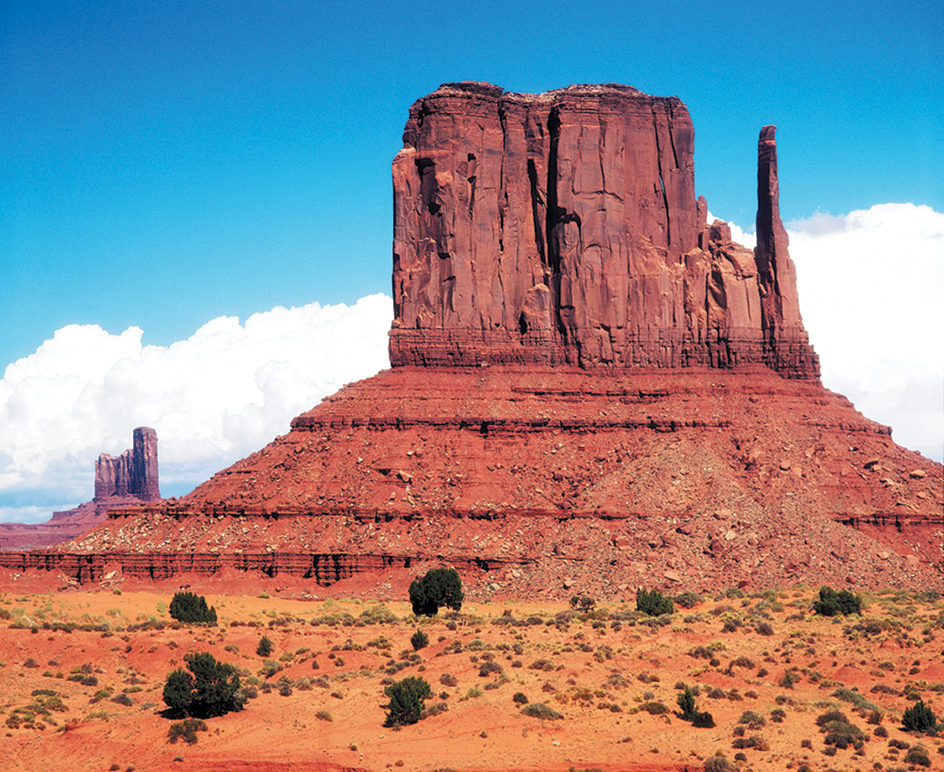
The abundant oxygen in Earth’s atmosphere today came mainly from plants and microorganisms such as algae. These organisms use carbon dioxide and give off oxygen through the process of photosynthesis. As such oxygen-producing organisms developed and became more plentiful, the amount of oxygen in the atmosphere increased.
The ice ages.
Throughout Earth’s history, the global climate has changed many times. Between about 750 million and 600 million years ago, during Precambrian times, Earth underwent several extreme climate changes called ice ages or glacial epochs, separated by warm periods. The climate may have grown so cold that some scientists believe Earth nearly or completely froze several times. The idea that the entire Earth froze is sometimes called the “snowball Earth” hypothesis. Other scientists think that the changes from a cold to a warm climate occurred too quickly for Earth to completely freeze.
Most of the time, Earth has been largely ice free. Brief ice ages occurred about 450 million years ago and 300 million years ago. In the last 50 million years, Earth’s climate has cooled. Glaciers began forming in Antarctica about 35 million years ago, but the climate there was warm enough for trees to grow until about 5 million years ago. By about 2.6 million years ago, at the beginning of the Pleistocene Epoch, large amounts of ice began accumulating on other continents as well.
Numerous separate ice advances, periods when ice sheets covered vast areas, occurred during the Pleistocene Ice Age. The advances alternated with periods when the climate was warmer and the ice melted. Geologists analyzing sediment deposits from the North Atlantic Ocean determined that there were at least 60 advances and retreats of ice sheets in the past 2 million years. Several of these ice advances were big enough to extend over much of Europe and to cover most of Canada and reach deep into the United States.
The most recent major advance of ice began about 70,000 years ago and reached its farthest extent about 20,000 years ago. The vast glaciers and sheets of ice scoured out the basins of the Great Lakes and blocked rivers. So much water was trapped in the form of ice that sea level around Earth dropped as much as 390 feet (120 meters), exposing parts of what is now ocean floor.
The most recent ice advance ended about 11,500 years ago. Most scientists believe that Earth is currently in an interglacial period and that another ice advance will follow. However, climate change caused by human activity may alter this outcome.
Scientists do not fully understand why Earth has ice ages. Most of them believe that ice ages may partly result from small, periodic changes in Earth’s orbit and the tilt of its axis caused by gravitational pull of other planets. These changes gradually alter the amount of energy received from the sun. Such changes are probably only a part of the reason for an ice age. Many scientists also believe that variations in the amount of carbon dioxide in the atmosphere are responsible for long-term changes in the climate.
Life on Earth.
Many rocks contain fossils that reveal the history of life on Earth. A fossil may be an animal’s body, a tooth, or a piece of bone. It may simply be an impression of a plant or an animal made in soft sediment before it hardened into rock. Fossils help scientists learn which kinds of organisms lived at different times in Earth’s history. Scientists who study prehistoric life are called paleontologists.
Loading the player...Fossil formation
Many scientists believe that life appeared on Earth almost as soon as conditions allowed. There is evidence of chemicals created by living things in rocks from the Archean age, 3.8 billion years ago. Fossil remains of microscopic living things about 3.5 billion years old have also been found at sites in Australia.
For most of Earth’s history, life consisted mainly of microscopic, single-celled creatures. The earliest fossils of larger creatures with many cells are found in Precambrian rocks that are over 600 million years old. Many of these creatures differed from any living things today.
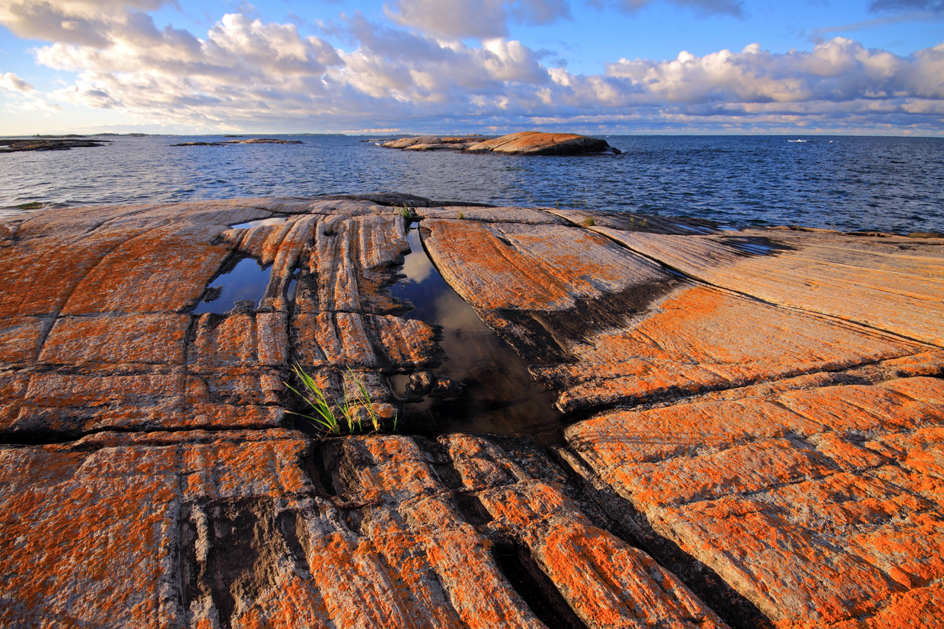
The Paleozoic Era.
Fossils become abundant in Cambrian Period rocks that are about 540 million to 490 million years old. This apparent sudden expansion in the number of life forms in the fossil record is sometimes called the “Cambrian explosion.” It marks the start of the Paleozoic Era. The Cambrian explosion actually occurred over tens of millions of years, but it appears sudden in the fossil record. Scientists are unsure whether this shows a relatively rapid increase in the diversity of life forms or whether the diversity evolved more gradually, with earlier life forms leaving fewer fossils.
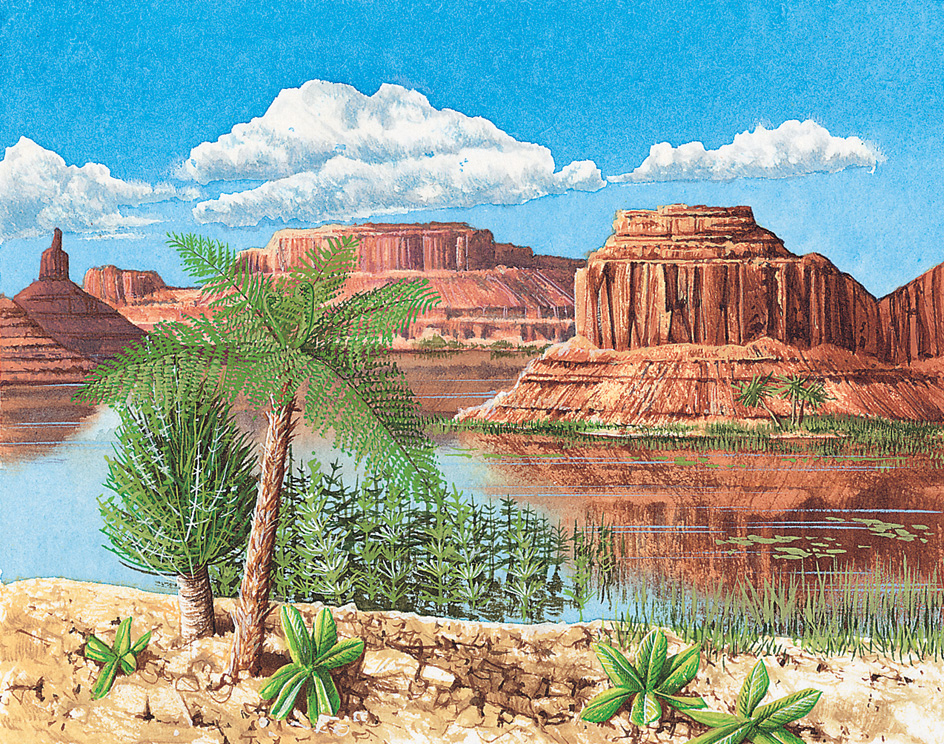
Most fossil organisms found in Paleozoic rocks are invertebrates (animals without a backbone). They include corals; mollusks, a group of soft-bodied animals that includes clams and snails; and prehistoric sea animals called trilobites. Fish first appear in Cambrian Period rocks about 500 million years old. Ordovician Period rocks about 470 million years old contain the earliest known fossil evidence of land plants. The first tetrapods (four-limbed animals) appear as fossils in Devonian Period rocks about 370 million years old. Some early tetrapods likely spent much of their lives on land. Like modern amphibians, they needed to lay eggs in water.
Fossil remains show that by 300 million years ago, large forests and swamps covered the land. The carbon-rich remains of some of these forests are preserved as coal deposits in the United States, Canada, the United Kingdom, and other parts of the world. The Carboniferous Period is named for these huge deposits of coal.
The earliest fossil remains of amniotes are found in rocks of the Carboniferous Period. Amniotes lay eggs with shells that can develop away from the water. These eggs gave amniotes an advantage over animals that had to return to water to lay their eggs. Toward the end of the Paleozoic Era, in rocks from the Permian Period, some fossil amniotes begin to show some characteristics of mammals.
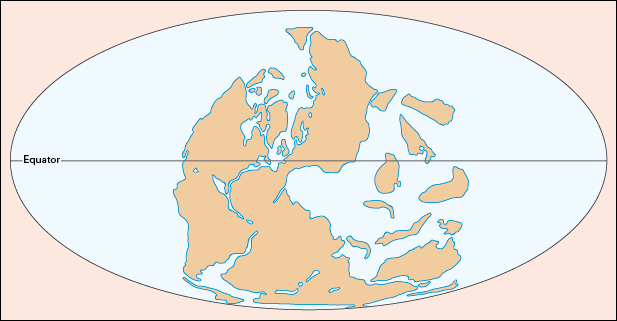
Several times in Earth’s history, there have been great extinctions, times when many of Earth’s living things die out. The greatest of these events, called the Permian extinction, happened about 250 million years ago. As much as 95 percent of the species on Earth became extinct in a relatively short time. The cause of this event is a mystery. But many scientists suspect that huge volcanic eruptions in what is now Siberia may have disturbed the climate, causing many organisms to die off.
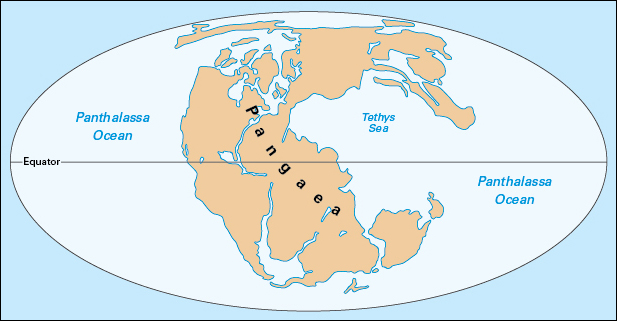
The Mesozoic Era.
Following the Permian extinction, the fossil record shows that reptiles became the dominant animals on land. The most spectacular of these reptiles were the dinosaurs. The Mesozoic is often called the Age of the Reptiles, but mammals and birds also appear in the fossil record during this time—mammals about 200 million years ago and birds about 150 million years ago. Most scientists believe that birds descended from dinosaurs. Fossil plants of the Mesozoic Era represent two main groups, gymnosperms and angiosperms. Gymnosperms have naked seeds, and most are cone-bearing. They include conifers, cycads, and ginkgoes. These gymnosperms evolved in the late Paleozoic Era and were dominant into the Cretaceous Period. Angiosperms have covered seeds and are flowering plants. They appeared and became the dominant plant group in the Cretaceous Period and continue to be so today.
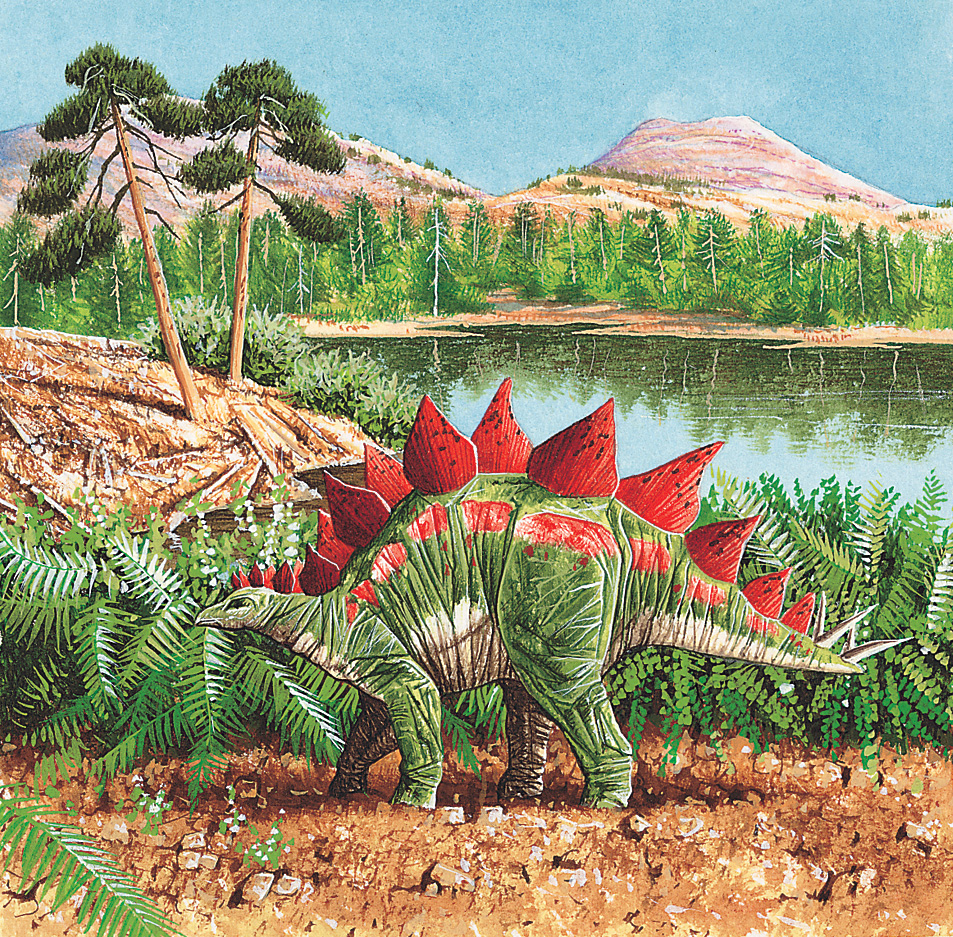
The dinosaurs died out in another great extinction about 65 million years ago. Most scientists believe that the extinction was caused by the impact of an asteroid with Earth. The impact would have thrown so much dust into the atmosphere that the surface would have been dark and cold for months or years, killing off plants and the animals that fed on them. Debris from the collision has been found all over the world.
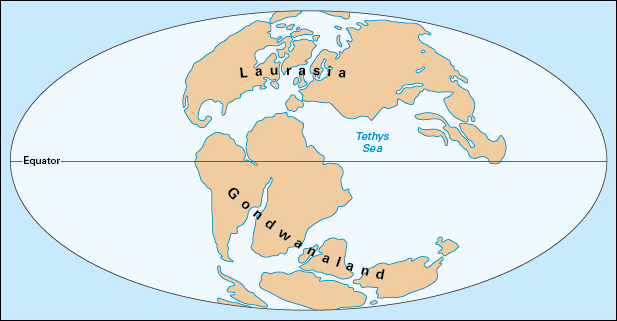
The Cenozoic Era.
The wide variety of plants and animals that we know today came into existence during the Cenozoic Era. Mammals survived the events that killed off the dinosaurs and expanded to become the dominant land vertebrates of today.
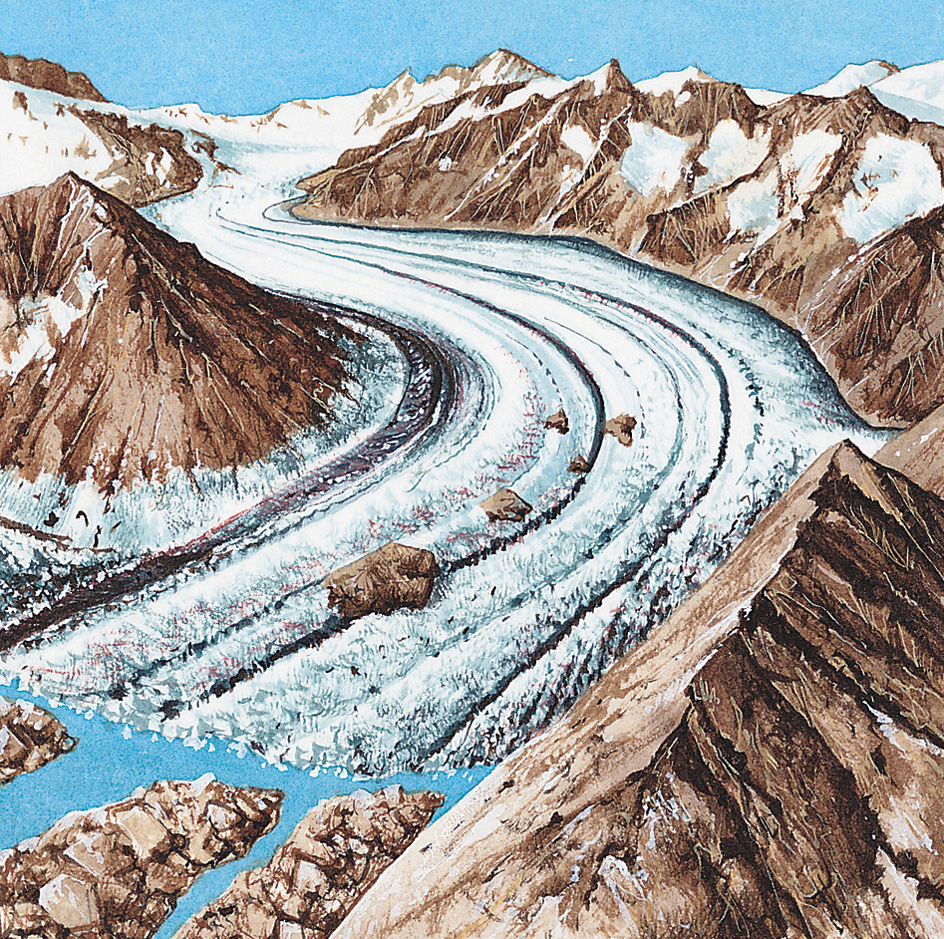
During the Eocene Epoch, ancestors of the horse, rhinoceros, and camel roamed North America. By the Oligocene Epoch, dogs and cats had appeared, along with three-toed horses about as large as sheep. The mammals developed in greater variety as prairies spread over the land during the Miocene Epoch.
By the Pliocene Epoch, many kinds of mammals had grown to gigantic size. Elephantlike mammoths and mastodons and giant ground sloths roamed the prairies and forests. These animals died out at the end of the Pleistocene Epoch.
Fossils of the first human beings—primates classified under the genus Homo—appeared on Earth approximately 2 million years ago. The first physically modern human beings appeared later, perhaps less than 200,000 years ago. Humanity’s years on Earth make up only a tiny fraction of the planet’s history.
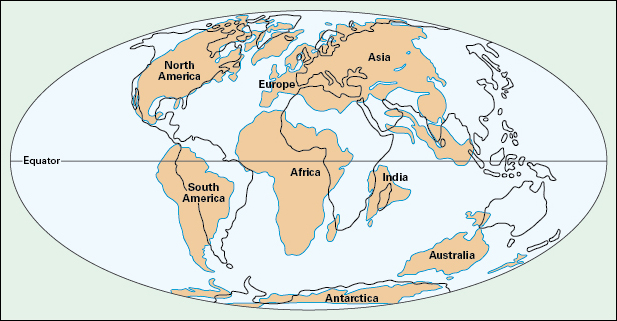
The current epoch is the Holocene. It began about 11,700 years ago, around the end of the last ice age. This date also coincides with the start of human agriculture.
Some scientists have proposed a new epoch called the Anthropocene. It is defined as the time in which humans have become the greatest force of change on Earth’s surface. Some of them believe the Anthropocene began in the early 1800’s, with the start of the Industrial Revolution. Still other scientists think it began as early as the beginning of the Holocene Epoch.
Human activity is now the most active surface process on Earth, moving more soil and rock than all other geologic processes combined. Driven by human activity, extinction rates in the last century have approached those of the great extinctions in the past. Humans have also changed the atmosphere, pushing carbon dioxide levels beyond those experienced naturally for millions of years. The resulting climate change is warming the polar regions dramatically, raising sea level, and likely fueling more extreme weather events.