Electricity is one of the most basic features of our universe. When most people hear the word electricity, they think of lighting, television, computers, air conditioners, and other electrically powered devices. Electricity makes possible these and many other useful things. But electricity is much more fundamental than that. The matter around us, for example, is made up of electrically charged particles. In fact, attraction among such particles holds together the atoms and molecules that make up matter. In this way, electricity is part of the structure of almost everything that exists.
Electricity includes a variety of effects related to charged particles. Perhaps the most familiar of these effects is electric current. An electric current is a flow of electric charges. It is electric current that flows through wires into our homes and powers our electric devices. Another familiar effect is static electricity. Static electricity occurs when an object or material accumulates an overall electric charge. Under the right conditions, the charge can be released in the form of a visible spark.
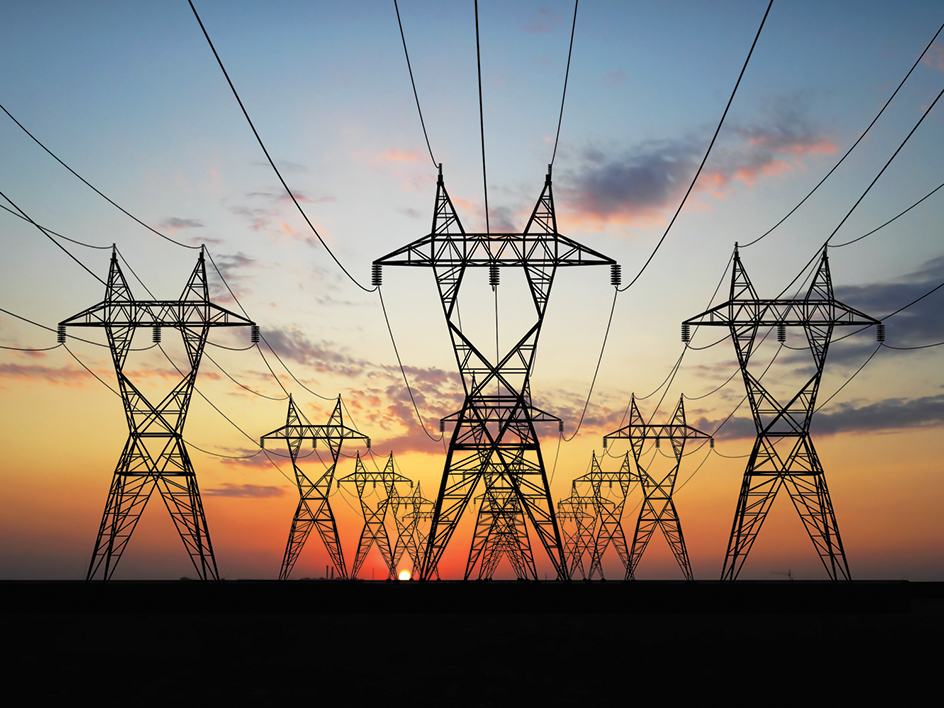
Electric charges come in two types: positive and negative. A positive charge will attract (pull on) a negative charge, and vice versa. This fact is often stated as Opposite charges attract. Two negative charges will repel (push away) each other, as will two positive charges.
Materials vary in their ability to conduct (carry) electric current. Current moves easily through materials called conductors. Metals are good conductors. Most wires used to carry electric current are made of metal, usually copper. Some liquids can also conduct electric current. For this reason, it can be dangerous to operate electrical equipment in wet conditions. Materials known as insulators resist the movement of current. Glass, rubber, plastic, dry wood, and dry air are good insulators. Insulators are important for electrical safety. Most electrical cords are made from a conductor covered with an insulator, such as rubber or plastic. The insulator helps to prevent heat and electric current from leaving the cord, reducing the risk of fire and electric shock.
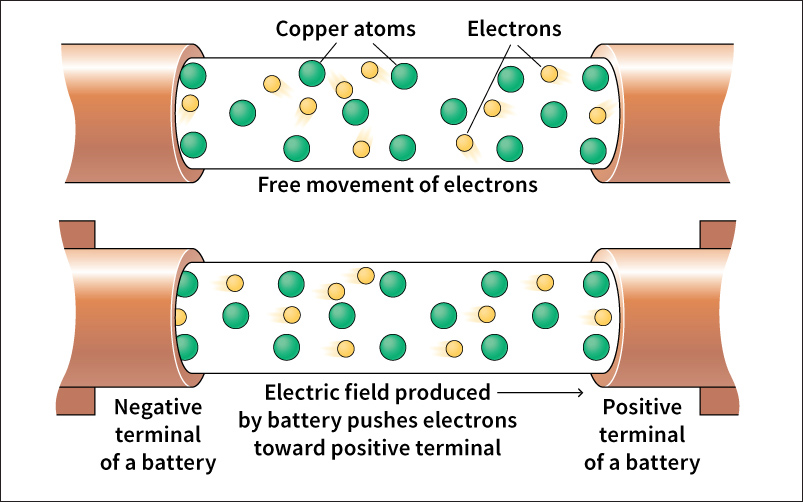
Electricity is closely related to magnetism. Every electric influence exerts a magnetic influence, and vice versa. In fact, electricity and magnetism together make up a single force called electromagnetism.
Electricity is also associated with many processes in living things. In the human body, for example, electrical and chemical impulses travel along nerves, carrying information to and from the brain. Such signals tell the brain what the eyes see, what the ears hear, and what the fingers feel. Electrochemical impulses from the brain tell muscles to move and tell the heart when to beat.
During the 1800’s, people learned to harness the energy of electric current to do work. This new form of power had so many practical applications that it greatly changed the way people lived. Inventors and scientists learned how to generate electric current. They found ways to use that current to produce light, heat, and motion. They developed electrically powered devices that enabled people to communicate across great distances and to process information quickly. The demand for electric power grew steadily during the 1900’s. In the 2000’s, most people could not imagine life without electric power.
Uses of electric power
Many aspects of our daily lives depend on electric power. People in developed nations use many electrically powered devices every day. One of the most important such devices is the computer, which uses electricity to process information. Computers are a fundamental part of the way many people live, learn, and work.
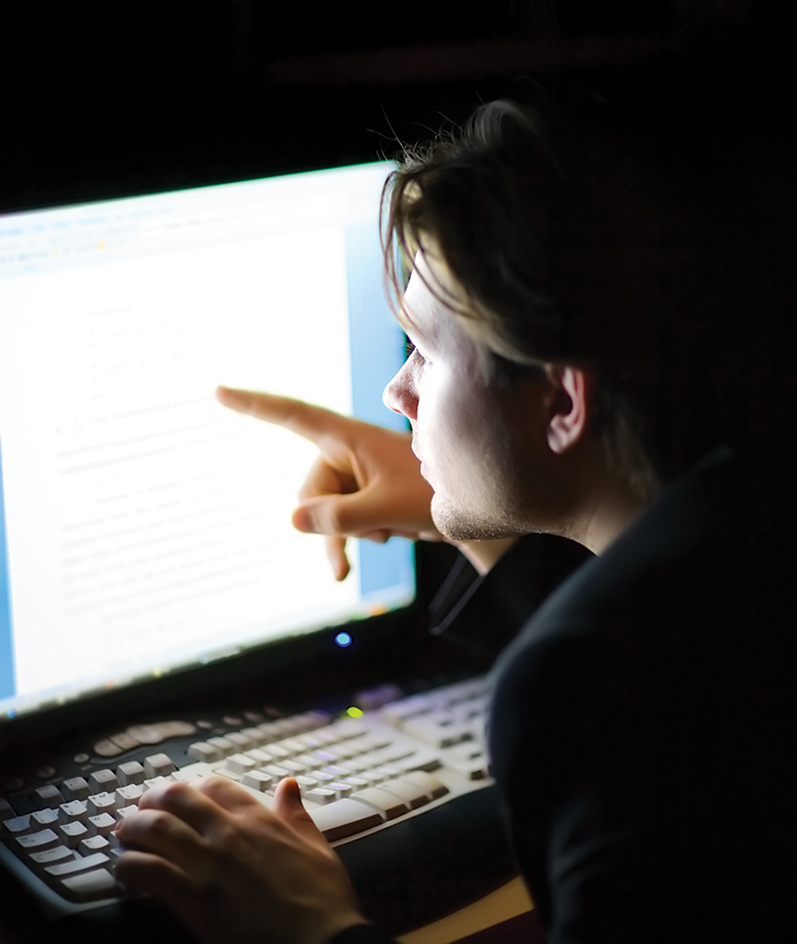
In homes.
Electric appliances save hours of labor once done by hand. Such appliances include dishwashers, vacuum cleaners, and washing machines. Electric ranges, microwave ovens, and food processors help us prepare meals quickly and easily. Refrigerators and freezers preserve food. Air conditioners and electric fans cool homes. Electric heaters provide warmth and hot water. Televisions, radios, computers, video game systems, and music players furnish entertainment. Electric lights brighten dark spaces.
In industry.
Modern industry would be impossible without electric power. Factories produce many items using electrically operated conveyor belts, robots, and other equipment. Manufacturers use electric instruments to ensure correct product sizes and quality. Drills, saws, and many other small tools run on electric power. Electric motors run elevators, cranes, and most other large machinery. Computers are vital to industry.
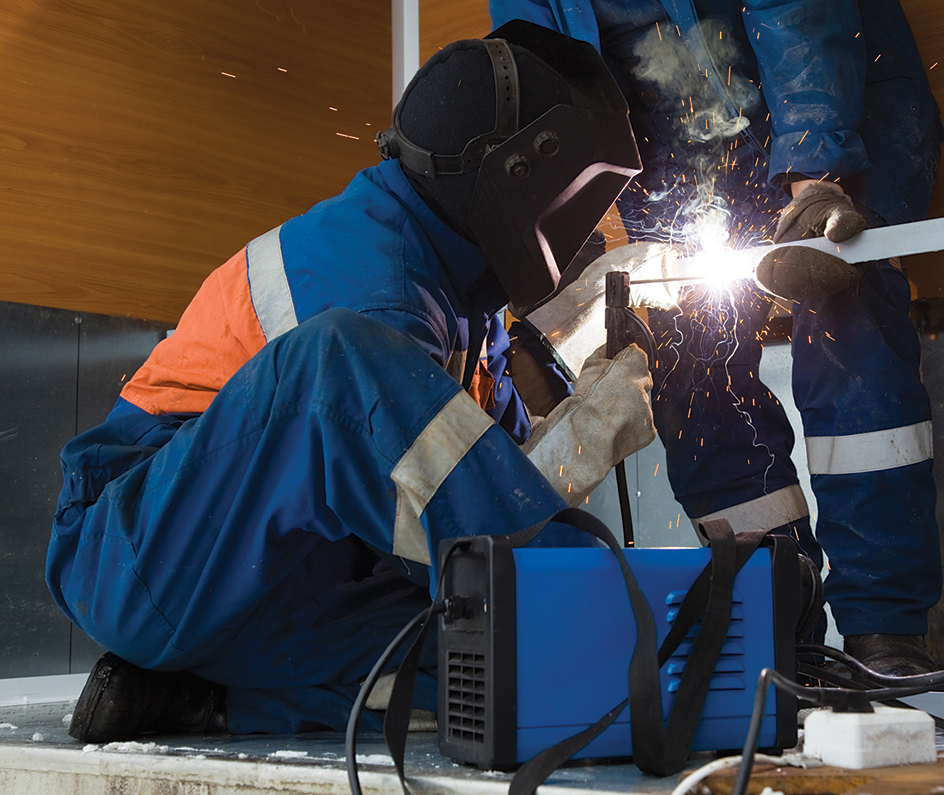
In communication.
Electric energy powers almost every device people use to communicate. Telephones, TV’s, radios, and computer modems all run on electric power. Communications satellites use electric energy from devices called solar cells to relay information around the world. Solar cells convert sunlight into electric energy. TV and radio signals are electromagnetic in nature. So are telephone and computer network signals.
In transportation.
Electric energy powers subways, trolleys, and trains that carry millions of people. Most automobiles use electric sparks to ignite the gasoline that powers the engine. Increasing numbers of cars rely on electricity for part or all of their main power. Many controls in airplanes and ships are electrically powered.
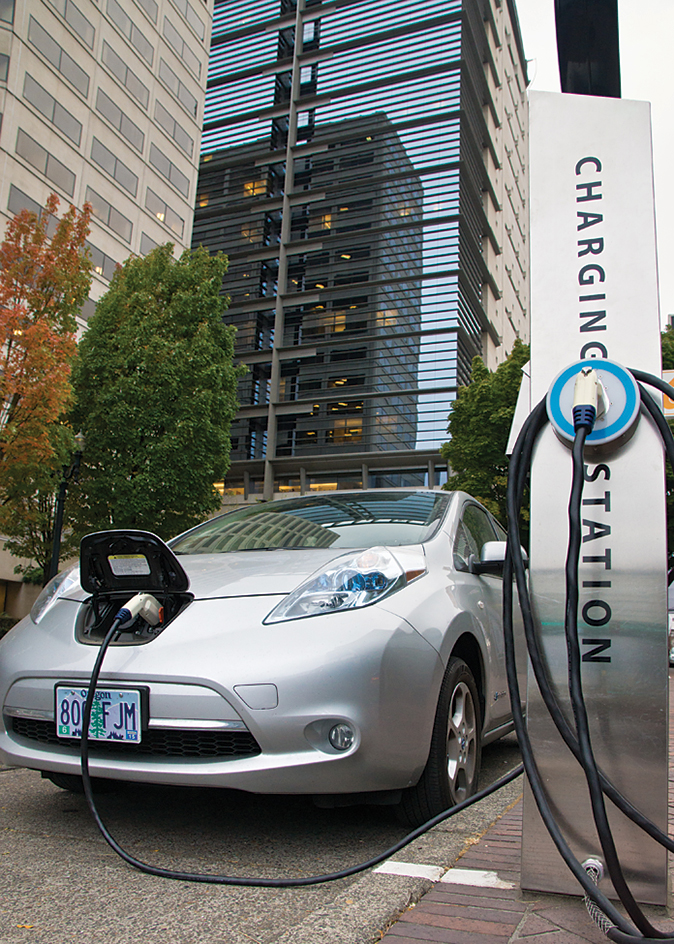
In medicine and science.
Health care workers use numerous electric instruments to examine patients and perform medical tests. For example, doctors look inside the body using X-ray machines and magnetic resonance imaging (MRI) machines, both of which use properties of electricity to operate. Machines called electrocardiographs (ECG’s) record tiny electrical signals from the heart. ECG’s help doctors to diagnose heart disease or damage. Electrically powered lasers are used for a variety of medical procedures. Their uses range from tattoo removal to vision repair to cancer treatment.
Scientists from every field use electric devices to conduct research. Microbiologists, for example, use powerful instruments called scanning electron microscopes to magnify and examine cells. Physicists use electrically operated particle accelerators to probe the interiors of atoms. Huge telescopes with electric motors and sensors help astronomers study planets, stars, and galaxies.
Electric circuits
The electric current in our homes is a flow of charged particles called electrons. There is energy associated with this flow. As current passes through an electric device, this electric energy may be converted to useful forms. For example, an electric range converts electric energy into heat. A light bulb converts it into light.
To make use of electric energy, an electric device must be connected to an energy source, such as an electrical outlet or battery. A complete path must be provided for current to flow from the energy source to the device and back again. Such a path is called an electric circuit.
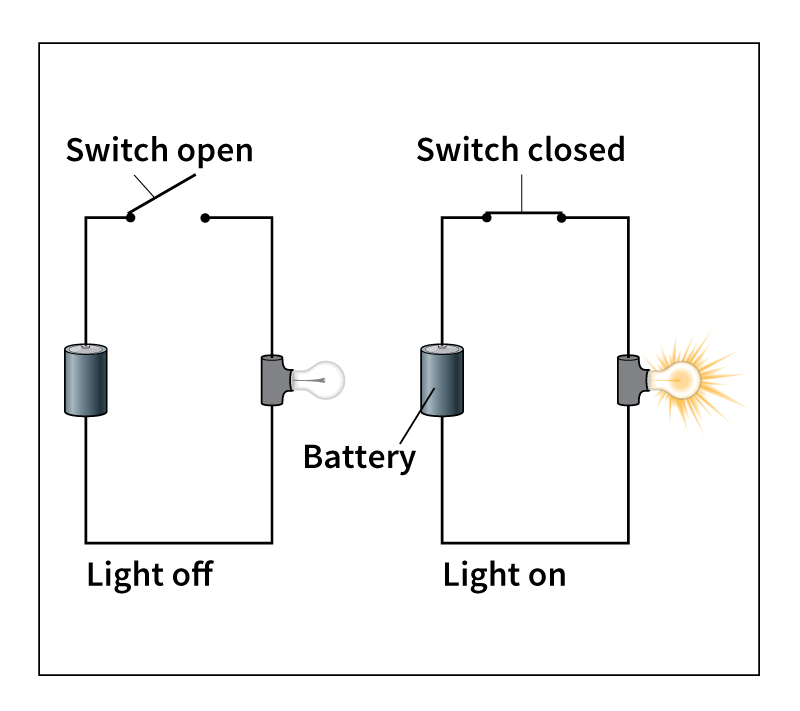
Simple circuits.
Suppose a person wanted to make a battery-powered light bulb shine. Electric current will flow to the bulb only if there is a complete circuit that leads from the battery to the bulb and back. To complete the circuit, the person must connect a wire from one terminal (end) of the battery to the light bulb. Then, the person must connect another wire from the light bulb to the battery’s other terminal. Electrons will then flow from the battery, through the light bulb, and back to the battery. The light bulb converts some of the current’s energy into light, causing the bulb to glow.
Series and parallel circuits.
A single battery or other power source often supplies power to more than one electric device. These instances require more complex arrangements such as series circuits and parallel circuits.
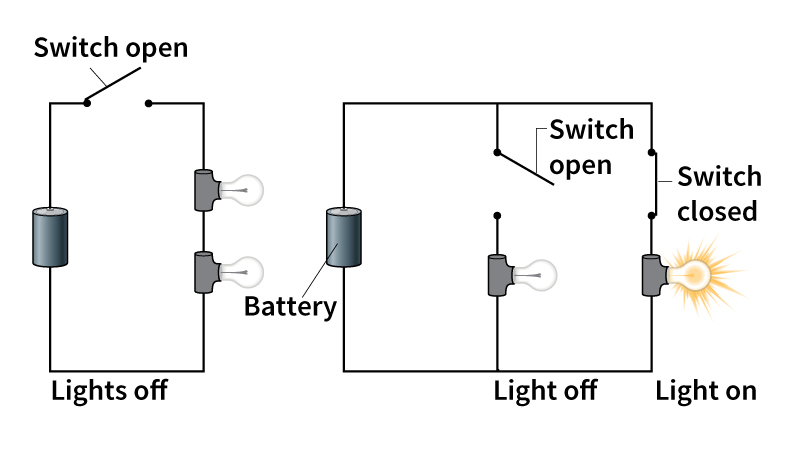
A series circuit features only one path. The same current flows from the energy source, through each of the devices on the circuit in turn, and back to the energy source. A simple series circuit might involve a battery and two light bulbs. A wire connects one terminal of the battery to one light bulb. Another wire connects that bulb to the second bulb. A third wire connects the second bulb to the battery’s other terminal.
Series circuits are used in flashlights, some decorative holiday lights, and other simple devices. One weakness of a series circuit is that the failure of any part of the circuit will disrupt the entire circuit. In the example, if one light bulb burns out, the path is broken, and current cannot flow. The other light bulb will go dark as well. Also, adding more devices, such as another bulb, to the circuit lowers the current to each device.
In a parallel circuit, the current splits to follow two or more paths. One example is a circuit in which two light bulbs are attached to both terminals of a battery by separate wires. The current in such a circuit splits, with half the electrons flowing through one bulb and half flowing through the other before returning to the battery.
Unlike in a series circuit, a parallel circuit provides the same level of current to all devices, no matter the number. Also, the failure of one part of the circuit will not knock out the entire circuit. In the example, if one light burns out, the other could continue to shine. Household lights and appliances are connected in parallel circuits.
Many circuits include some parts that are series and some that are parallel. A complex circuit, like that in a computer or TV, can have millions of parts connected in various series and parallel combinations.
Switches.
The simplest way to control the flow of current through a circuit is with a switch. A basic switch consists of two electrical conductors that can be separated to create a gap in a circuit. When the gap is open, the switch is off. No current flows. When the switch is on, the conductors are connected, and current flows.
Alternating and direct current
Current can flow through a circuit in two different ways. Direct current (DC) flows in only one direction. Batteries produce direct current. In a simple DC circuit, electrons flow from one battery terminal, through the circuit, to the other terminal. Alternating current (AC) rapidly reverses direction. In an AC circuit, individual electrons move back and forth in the wires, not traveling the entire circuit.
The current in household wiring is alternating current. In the United States and Canada, household current reverses direction 120 times per second, completing 60 full cycles. In other parts of the world, such as Australia, Europe, and South Africa, 50 cycles is the standard.
Static electricity
Electrons are part of the atoms that make up matter. Sometimes, the atoms in an object gain or lose electrons. When this happens, the entire object can take on a stationary electric charge until it is discharged. The term static electricity describes such situations.
Static electricity occurs, for example, when a person rubs a balloon on a shirt. The rubbing between the cloth and the balloon causes electrons to transfer from the shirt to the balloon. Electrons have a negative electric charge, so the extra electrons give the balloon an overall negative charge. The shirt, which has lost electrons, gains a positive electric charge. The opposite charges attract, enabling the two to stick together. If the conditions are right, the balloon may stick to a neutrally charged object, such as a wall.
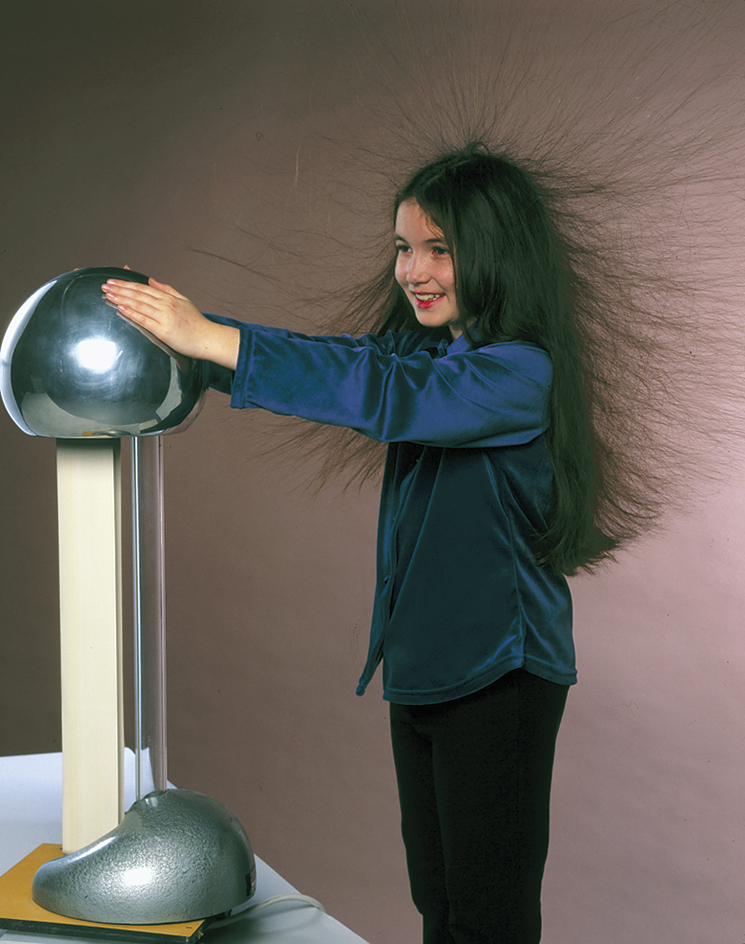
Similarly, when a person walks across a rug on a dry day, rubbing between the shoes and the rug can transfer electrons from the rug to the person’s body. The transfer gives the body a negative charge. If the person touches a doorknob or other metal object, electrons may jump from the body to the object. The person may see a spark and feel a slight shock.
Static electricity also causes lightning. Most scientists think that collisions among various forms of water and ice build up areas of electric charge within a storm cloud. Parts of the cloud become positively charged. Other parts become negatively charged. Charge may eventually jump between two areas, including from cloud to cloud or from the cloud to the ground. The result is the huge electric spark we call lightning.
Static electricity has many uses in homes, businesses, and industries. For example, electrostatic copying machines make duplicates of printed or written material by attracting negatively charged particles of toner (powdered ink) to positively charged paper. Static electricity is also used in air cleaners called electrostatic precipitators. These devices put a charge on airborne particles, such as dust, smoke, bacteria, or pollen. Oppositely charged plates then attract the particles out of the air.
Electrical safety
Most people know that electricity can be dangerous. Understanding why can help you to avoid injury and to use electric power safely.
Electric shock
occurs when an electric current passes through the body. The body relies on natural electrical and chemical signals that travel along nerves, carrying information to and from the brain. These signals regulate the beating of the heart and other vital functions. Currents flowing through the body can disrupt these signals. The currents may cause muscle contractions, heart and respiratory failure, and death. Electric current can also burn skin and other body tissues.
The danger presented by electricity depends in part on its voltage. Voltage measures the “push” that a source of electric energy supplies to move a charge through a circuit. For a given circuit of set resistance, the higher the voltage, the stronger the current. The voltage of a flashlight or radio battery is usually too low to generate enough current to cause serious injury. But the 120 volts available at most household outlets can create a current strong enough to severely injure or even kill a person. The danger of electric shock is much greater when a person’s skin is wet. Water lowers the body’s resistance to the flow of electricity. A given voltage can then pass a greater current through the body.
Most electric devices have safety features to help prevent shock. Many appliances and tools have a plug with a third prong. This prong connects the metal parts of the device to a wire leading to the ground. If the wiring in the device becomes defective, the third prong usually causes the current to flow harmlessly to the ground.
Outdoor dangers.
If you climb a tree near an electric power line, you may get a shock if the tree touches the line. Storms sometimes knock down electric power lines. You could be injured or killed if you touch a fallen line when the power is still on.
Lightning discharges may involve about 100 million volts. This voltage is more than enough to kill a person. You can avoid lightning dangers by staying indoors during a storm, away from metal plumbing and fixtures, as well as corded appliances. If you get caught outdoors, stay away from open fields, open water, and high places. A forest is usually safer than open land. But do not stand under a tall or isolated tree, which is more likely to be struck. The inside of a car is generally safer than outdoors. If the vehicle is struck by lightning, its metal body will often conduct the electric charge around the outside of the car. The interior of the car will be unharmed.
Electrical fire
is another danger. When an electric current passes through a conductor, it can cause the conductor to become hot. Sometimes the heat is desirable. For example, the wires in a toaster heat up to brown bread. But overheating in electrical cords or in household wiring can cause a fire. Electrical fires destroy many homes every year. To avoid fires, do not plug too many devices into the same outlet. Never use electric devices with worn or frayed cords.
Electricity and matter
The electron is the smallest free particle of negative charge. Another particle, called a proton, has the exact same amount of charge as the electron, but the proton’s charge is positive. Along with an uncharged particle called the neutron, protons and electrons are the building blocks of matter.
Atoms.
Protons, neutrons, and electrons combine to form atoms. Protons and neutrons join to form a core, called the nucleus. The nucleus is positively charged because of the protons. It is also relatively heavy, because neutrons and protons are much larger and heavier than electrons. The positively charged nucleus attracts the negatively charged electrons. The electrons are light and fast and move quickly around the nucleus.
Each type of atom has a different number of protons in its nucleus. For example, hydrogen, the simplest atom, has only 1 proton in its nucleus. An oxygen atom has 8 protons. Iron has 26. Uranium has 92. Normally, an atom has an equal number of protons and electrons. As a result, the negative charges of the electrons exactly balance the positive charges of the protons. The atom is, therefore, electrically neutral overall.
Ions.
Sometimes an atom loses or gains one or more electrons. If it gains an electron, the atom takes on a negative charge. If it loses an electron, the atom takes on a positive charge. Atoms that carry an electric charge are called ions. Positive and negative ions attract each other and can combine to form solid materials. Ordinary table salt, for example, consists of sodium and chlorine. Each sodium atom gives up one electron to form a positive sodium ion. The chlorine takes on this electron to become a negative chloride ion. Strong electrical attraction between the two types of ions makes salt a solid material with a high melting point.
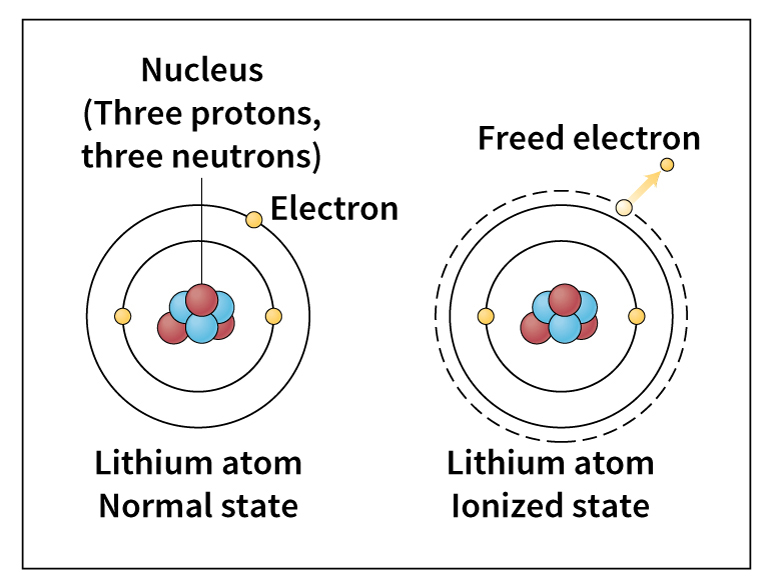
Molecules.
Neutral atoms often come together to share electrons. If the two atoms are not identical, the electrons often spend more time near one atom than the other, making one atom slightly more positive and the other atom more negative. Electrical attraction between the positive and negative atoms causes them to join into molecules. For example, two hydrogen atoms can share electrons with one oxygen atom to make a water molecule. The electrons spend more time near the oxygen atom, giving it a slight negative charge. The two hydrogen atoms take on slight positive charges. Attraction between the atoms holds the water molecule together.
Like lone atoms, molecules can also lose or gain electrons. When this happens, the molecule itself takes on an overall charge, becoming an ion.
Conductivity
To be a conductor, a material must have charged particles that are “free” to move throughout, carrying charge with them. In most conductors, the free particles are loosely held electrons. Most metals are good conductors, because they have a large number of free electrons. In some conductors, the current is a flow of ions, rather than electrons. Salt water, for example, is a conductor because it contains sodium and chloride ions that are free to move about, forming a current.
In an insulator, on the other hand, electrons are tightly bound to atoms and are not free to move around. If electric charge is applied to an insulator, the charge will stay in place and not move through the material.
Resistance.
A material’s opposition to the passage of electric charges is called resistance. Resistance occurs because electrons moving in the material collide with atoms and one another, losing energy and slowing down. The energy lost by the electrons is converted to heat. A good conductor, such as copper, has low resistance. Insulators, such as glass or wood, have such high resistance that it is extremely difficult for electric charges to flow through them.
Resistance depends not only on the type of material but also on its size and shape. For example, a thin copper wire has greater resistance than a thick one. A long wire has greater resistance than a short one. A material’s resistance may also vary with temperature. For metals, resistance typically increases with temperature.
Semiconductors
are materials that conduct charges more readily than do insulators but not as well as do conductors. Silicon is the most commonly used semiconductor. By adding small amounts of other substances to a semiconductor, engineers can adjust its capacity to conduct electric charge. Semiconductors are essential to the operation of computers, television sets, electronic games, and many other devices.
Semiconductors generally have a higher resistance than conductors. For semiconductors, resistance generally decreases with temperature.
Plasma.
If a gas is greatly heated, many electrons may be freed from their atomic nuclei (the plural of nucleus). When this happens, the gas becomes a plasma. The freed electrons make plasmas excellent conductors. The hot glowing substance inside a fluorescent light is one example of a plasma conductor. The hot materials that make up the sun and other stars are also plasma.
Superconductors.
In some materials, called superconductors, electrons move freely, without resistance. Superconductors can thus carry current with no loss of energy. However, known superconductors function only at extremely low temperatures. For this reason, superconductors are used only in special situations. Engineers are working to develop higher temperature superconductors for use in highly efficient motors, generators, and power lines.
In superconductors, the electrons pair up in a special arrangement known as Cooper pairs. This arrangement is named for its discoverer, the American physicist Leon Cooper. Single electrons bump into one another while traveling through a material. But Cooper pairs can travel together in unison without colliding. This means that current in a superconducting wire never loses energy. A loop of such a wire could carry a current that travels around and around forever.
As electrons move through a normal conductor, they create an invisible region of electric influence called an electric field. The electric field, in turn, produces a region of magnetic influence called a magnetic field. This magnetic field exists inside and outside the material. In a superconductor, the magnetic field is expelled from (pushed out of) the material.
Sources of electric energy
For current to flow, a positive charge must be applied to one end of a conductor and a negative charge to the other end. In a wire, the negative charge repels electrons, and the positive charge attracts them, causing electrons to flow from the negative end to the positive end. To maintain the difference in charges, a continuous source of energy is required. The energy may come from chemical action, motion, sunlight, or heat.
Batteries produce electric energy by means of chemical action. A simple battery has two structures called electrodes. Each electrode is made from a different chemically active material. Between the electrodes, the battery contains a liquid or paste called an electrolyte. The electrolyte conducts ions but not free electrons. The electrolyte also helps promote chemical reactions at each electrode. At one electrode, called the anode, the reactions separate atoms into free electrons and ions. The positively charged ions move through the electrolyte to the other side of the battery, called the cathode. But the freed electrons cannot follow because the electrolyte is an insulator to them. The electrons instead flow out of the battery and into a circuit connected to the anode and cathode. Having traveled the circuit, the electrons reenter the battery at the cathode. There, they reunite with ions to form neutral atoms or molecules.
Eventually, a battery runs out of chemical energy. Then it can no longer produce electric energy. Some worn-out batteries can only be discarded. Others, called rechargeable batteries, can be charged again by passing electric current through them in the opposite direction.
Electric generators change mechanical energy, the energy of motion, into electric energy. Generators basically consist of coils of wire and a magnet. A source of mechanical energy spins either the magnet or the coil. The relative motion causes the magnet to force electrons through the wire, producing a current. The mechanical energy is often supplied by the motion of steam, water, or wind turning a turbine. For a detailed discussion of the sources of electric power, see Energy supply.
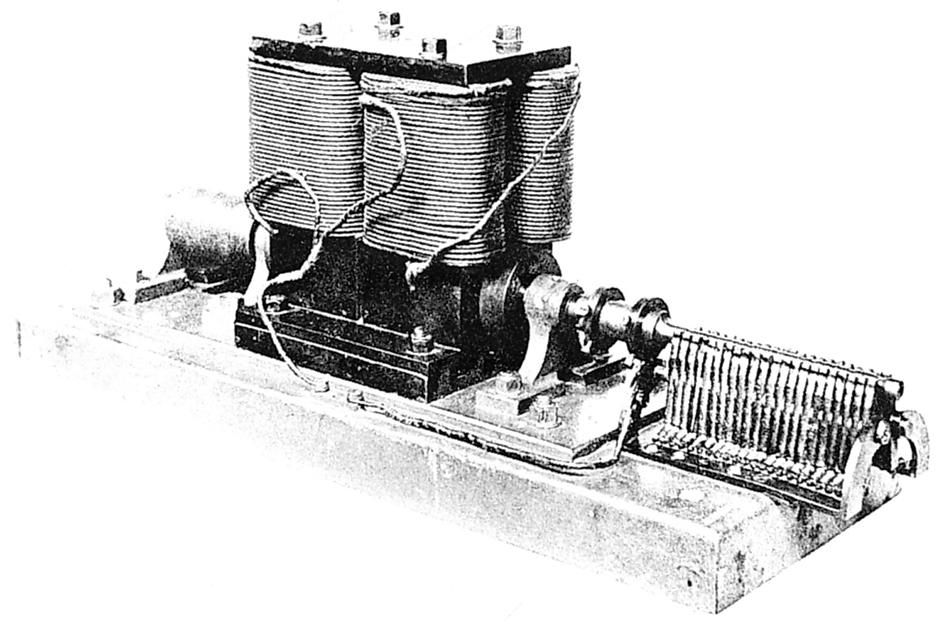
Generators furnish most of the electric power people use. A large generator in an electric power plant can provide enough electric energy for over 1 million people. Electric current from the generator reaches homes, factories, and offices through networks of power lines. A small generator called an alternator produces electric energy in an automobile. The energy is used to recharge the battery and power the electrical systems, including providing the spark that ignites the fuel in a gasoline engine. The motion of the engine turns the alternator.
Solar cells, also called photovoltaic cells, power most artificial satellites and other spacecraft. They also power some outdoor lighting, traffic cameras, calculators, and watches. Photovoltaic cells are made from semiconductors, usually specially treated silicon. Energy from the sun separates negative and positive charges in the semiconductor, causing current to flow through a circuit.
Some nonmetallic minerals develop electric charge along their surfaces when stretched or compressed. These materials are called piezoelectric crystals. Quartz is the most common piezoelectric crystal. Some microphones use piezoelectric crystals to convert sound vibrations into electrical signals for recording or radio broadcasting. Modern appliances, such as gas ranges and water heaters, have piezoelectric crystals instead of pilot lights. When compressed, the crystals produce electric sparks that ignite the gas.
Controlling current
Wires and electric devices become dangerously hot when they carry too much current. Switches called fuses and circuit breakers protect the wiring in most buildings. If too much current flows into an outlet, a fuse or circuit breaker will shut off the current. Many individual electric devices also contain fuses.
Sometimes people need to vary the strength of current. One way to adjust current strength is to vary resistance within the circuit. For example, using a light dimmer operates a device called a variable resistor or rheostat. This device adjusts the circuit’s resistance, making the light brighter or dimmer.
Traditional switches and variable resistors cannot change current quickly enough for certain tasks. Tiny semiconductor devices called transistors can adjust current more rapidly. Transistors act as high-speed switches, turning on and off billions of times each second. Some devices contain millions of transistors on a single tiny chip of silicon, called an integrated circuit or chip. Integrated circuits form the heart of computers, video-game consoles, and many other devices.
Electronic devices carry electrical signals that can be varied in some way to represent information. Electronic devices include capacitors, diodes, inductors, integrated circuits, and transistors. Signals may represent sounds, pictures, numbers, letters, computer instructions, or other information. For example, transistors in a music player provide a continuous range of currents that strengthen electrical signals representing the sounds being played.
Electromagnetism
The attraction and repulsion between electric charges results from the existence of electric fields. An electric field surrounds each charged particle. A charged particle that enters the field of another charged particle will feel a force. If the charges are alike, the force will push them apart. But if the charges are opposite, the force will pull them together. Electric fields are how charged particles interact, even without touching. The field around a charged particle goes on almost forever, but gets weaker and weaker with distance.
Similarly, a magnet is surrounded by a magnetic field. Interaction between magnets and magnetic fields causes attraction or repulsion between magnets.
A still electric charge produces an electric field, and a still magnet produces a magnetic field. However, a moving electric field produces a magnetic field, and a moving magnetic field produces an electric field. The two fields together make up a fundamental force of the universe called electromagnetism.
Any time a charge moves, an electromagnetic field is produced. For example, passing an electric current through a coil of wire makes the coil a temporary magnet called an electromagnet. The electric current creates a magnetic field around the coiled wire. As long as the current flows, the coil will act as a magnet.
Magnetism can, in turn, produce electric current. For example, a coil of wire moving near a magnet causes an electric current to flow in the wire. The current flows as long as the movement continues. Generators can produce electric current in this way.
Electric and magnetic fields can change together to make electromagnetic waves, also called electromagnetic radiation. These waves carry energy known as electromagnetic energy at the speed of light. Visible light, radio and TV signals, and microwaves all consist of electromagnetic waves. The infrared rays that cause a person to feel heat when standing near a hot stove are also electromagnetic in nature. The ultraviolet rays that cause sunburn consist of electromagnetic waves. The X rays that doctors use to see inside the body are electromagnetic waves. The powerful gamma rays that come from nuclear reactors and from outer space are also electromagnetic waves.
History
Several thousand years ago, the ancient Greeks observed that amber (fossilized resin) attracts bits of lightweight material, such as feathers or straw, after being rubbed with cloth. Amber is a good electric insulator, so it easily holds a static charge. Although the Greeks did not know about electric charge, they were actually experimenting with static electricity. The Greek word for amber is elektron. The English words electricity and electron come from this word.
A number of peoples, including the ancient Greeks and Chinese, knew of a different substance that could attract metal. It was a black rock called lodestone or magnetite. Lodestone is a natural magnet. It attracts iron objects, which tend to be heavy. In contrast, amber attracts only light things, such as straw. In 1551, the Italian mathematician Girolamo Cardano, also known as Jerome Cardan, realized that the attracting effects of amber and of magnetite must be different. Cardano was the first to note the difference between electricity and magnetism.
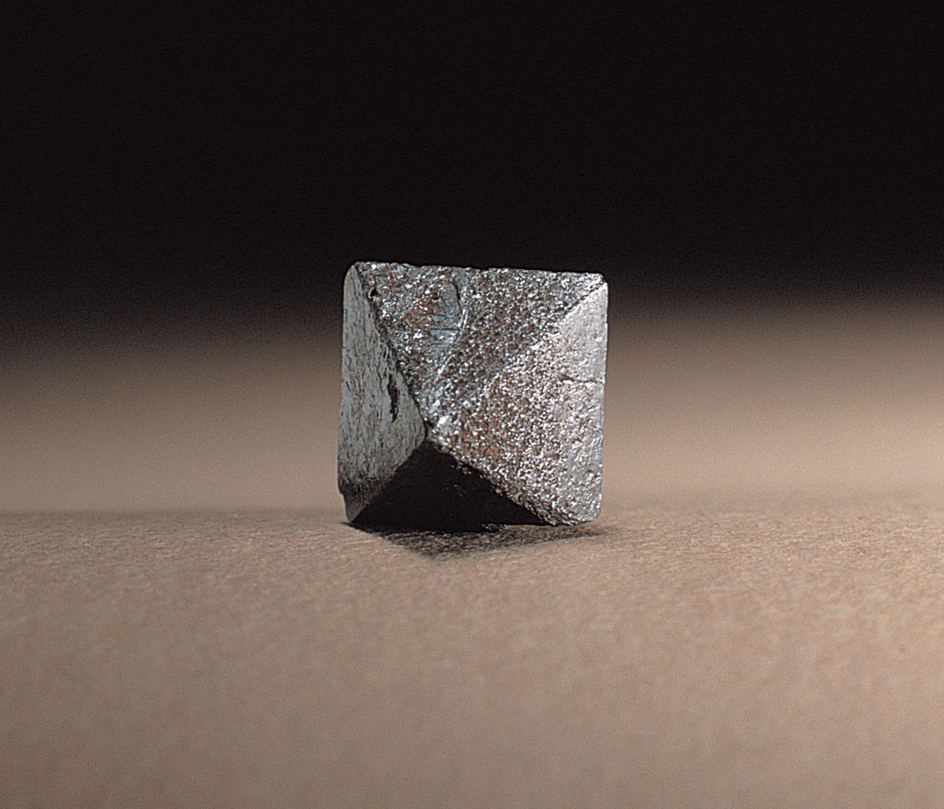
In 1600, the English physician William Gilbert reported that such materials as glass, sulfur, and wax behaved like amber. When rubbed with cloth, they too attracted light objects. Gilbert called these materials electrics. He studied the behavior of electrics and concluded that their effects must be due to some kind of fluid. Today, we know that what Gilbert called electrics are actually materials that are good insulators.
Experiments with electric charge.
In the 1730’s, the French scientist Charles Dufay found that charged pieces of glass attracted amberlike substances but repelled other glasslike substances. Dufay decided that there must be two kinds of electricities. He called them vitreous (for glasslike substances) and resinous (for amberlike substances). Dufay had found negative and positive electric charge. However, he thought of them as two kinds of “electric fluid.”
The American scientist and statesman Benjamin Franklin began to experiment with electricity in 1746. Franklin thought that there was only one kind of electric fluid. He theorized that objects with too much fluid would repel each other, but they would attract objects with too little fluid. If an object with an excess of fluid touched an object deficient in fluid, the fluid would be shared. Franklin’s idea explained how opposite charges cancel each other out when they come in contact.
Franklin used the term positive for what he thought was an excess of electric fluid. He used the term negative for a deficiency of fluid. Franklin did not know that electricity is not a fluid. Rather, electricity is associated with the charges of electrons and protons. Today, we know that most positively charged objects actually have a deficiency of electrons. Negatively charged objects have an excess of electrons.
In 1752, Franklin said he performed an experiment by flying a kite in a thunderstorm. Franklin said that the kite and string became electrically charged. He therefore concluded that the storm clouds were themselves charged. He became convinced that lightning was a huge electric spark. Experiments such as the one Franklin described are dangerous. Lightning can electrocute people who fly kites in storms.
In 1767, the English scientist Joseph Priestley described a mathematical law that shows how the attraction between oppositely charged objects weakens with distance. In 1785, the French scientist Charles Augustin de Coulomb confirmed Priestley’s law. Coulomb showed that the law also held true for the repulsive force between objects with the same charge. Today, the principle is known as Coulomb’s law.
In 1771, Luigi Galvani, an Italian anatomy professor, found that the leg of a recently killed frog would twitch when touched with two different metals at the same time. Galvani’s work attracted much attention. In the late 1790’s, Alessandro Volta, an Italian physicist, offered an explanation. Volta showed that chemical action occurs in a moist material in contact with two different metals. The chemical action results in an electric current. The flow of current had made Galvani’s frog twitch. Volta gathered pairs of disks, each pair consisting of one silver and one zinc disk. He separated the pairs with paper or cloth moistened with salt water. By stacking such disks in a pile, Volta built the first battery, called a voltaic pile.
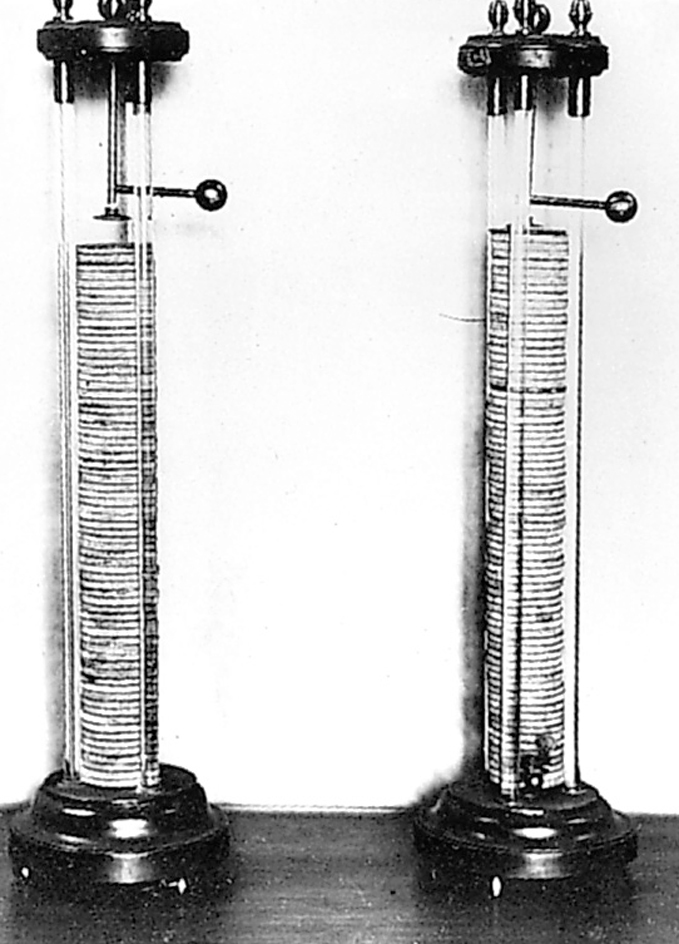
There followed many experiments with Volta’s battery and electric circuits. The German physicist Georg S. Ohm devised a mathematical law to describe the relationships among current, voltage, and resistance for certain materials. Ohm described his law in a treatise published in 1827. According to Ohm’s law, a larger voltage can push a larger current through a given resistance. In addition, a given voltage can push a larger current through a smaller resistance.
Electricity and magnetism.
In 1820, the Danish physicist Hans C. Oersted found that an electric current flowing near a compass needle would cause the needle to move. Oersted was the first to show a definite connection between electricity and magnetism. During the 1820’s, André-Marie Ampère of France discovered the mathematical relationship between currents and magnetic fields. That relationship, called Ampere’s law, is one of the basic laws of electromagnetism.
In the early 1830’s, the English scientist Michael Faraday and the American physicist Joseph Henry independently discovered that moving a magnet near a coil of wire produced an electric current in the wire. Further experiments showed that electrical effects occur any time a magnetic field changes.
The Scottish scientist James Clerk Maxwell combined all the known laws covering electricity and magnetism into a set of four equations. Maxwell’s equations were published in 1865. They describe completely how electric and magnetic fields arise and interact. Maxwell made a new prediction that a changing electric field would produce a magnetic field. That prediction led him to propose the existence of electromagnetic waves.
In the later 1880’s, the German physicist Heinrich R. Hertz showed how to generate and detect radio waves. Hertz thus proved Maxwell correct. In 1901, the Italian inventor Guglielmo Marconi and his staff sent and received electromagnetic waves across the Atlantic Ocean. Their success set the stage for radio, TV, satellite communications, and cell phones.
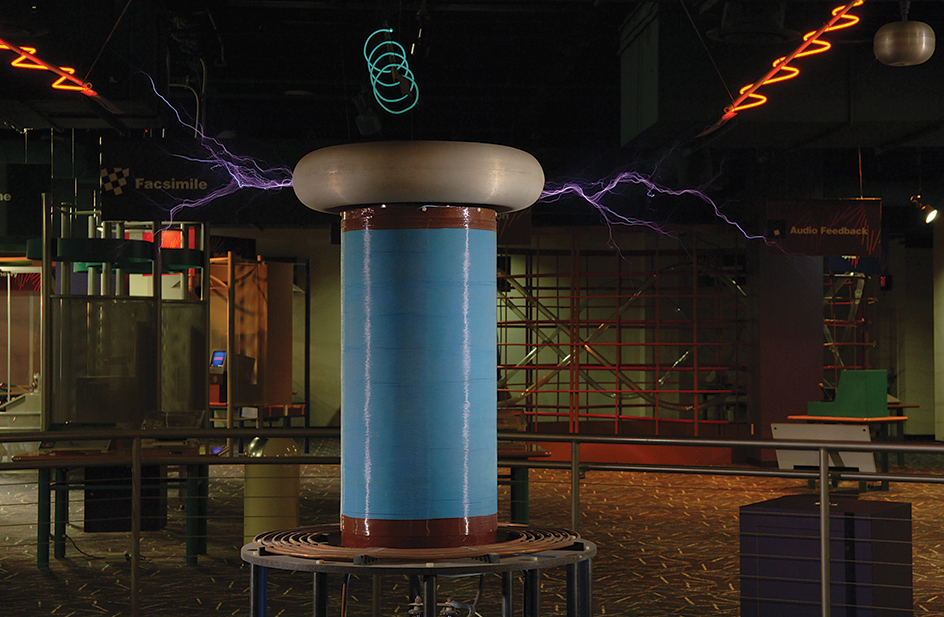
The electronic age.
The Irish physicist G. Johnstone Stoney believed that electric current was actually the movement of small, electrically charged particles. In 1891, he suggested that these particles be called electrons. In 1897, the English physicist Joseph John Thomson proved the existence of electrons. Thomson also showed that all atoms contain electrons. In research published in 1913, the American physicist Robert A. Millikan accurately measured the electron’s charge.
In the late 1800’s, scientists discovered that electrons could be dislodged from a metal surface in a vacuum tube. A vacuum tube is a glass tube with most of the air removed. The tube contains electrodes with wires that extend through the glass. Linking batteries to the electrodes causes a current of electrons to flow within the tube. The current can be modified by adjusting the voltage. Vacuum tubes can amplify, combine, and separate weak electric currents. This invention helped make radio, TV, and other technologies possible.
In 1947, the American physicists John Bardeen, Walter H. Brattain, and William Shockley invented the transistor. Transistors do the same jobs as vacuum tubes, but they are smaller and more durable. They also use far less energy. By the 1960’s, transistors had replaced vacuum tubes in most electronic equipment. Since then, electronics companies have developed ever smaller transistors. Today, millions of interconnected transistors fit on a single chip called an integrated circuit.
Demand for power
increases every year. Most of the electric energy we use comes from power plants that burn fossil fuels, such as coal, oil, or natural gas. Some electric energy comes from nuclear and hydroelectric (water power) plants. Smaller amounts come from solar cells, windmills, and other sources.
Many people are concerned that Earth’s supply of fossil fuel is limited. Someday, it will run out. Another problem is that present methods of generating electric power may harm the environment. In response, scientists, engineers, and power companies are trying to develop alternative sources of electric energy. Such sources include solar energy, geothermal energy (energy from Earth’s heat), wind energy, and tidal energy (energy from ocean waves and currents).
Many scientists hope that new electric devices will actually help curb the growing demand for electric energy. Computers, for example, can control the lighting, air conditioning, and heating in buildings to reduce energy use. Gas-based light bulbs called compact fluorescent lamps (CFL’s) provide the same light as traditional incandescent bulbs but use only about one-fourth the energy. A newer kind of lighting based on devices called light-emitting diodes (LED’s) is even more efficient. Many home appliances are now designed to conserve energy, and houses are being built to need less heating and cooling. Computers and communication systems enable people to work at home, often saving energy they would have used for transportation.