Energy, in physics, is a quantity related to work. Energy has many forms. For example, you use mechanical energy to toss a ball, heat energy warms a room, electric energy makes a light bulb glow, and chemical energy provides the driving force for an automobile.
Solar energy, energy from the sun, warms and illuminates Earth and its atmosphere. Almost all of that energy arrives at the top of the atmosphere as electromagnetic waves. From the least energetic to the most energetic, those waves include radio waves; infrared rays, which we feel as heat; visible light; ultraviolet rays, which can cause sunburn; and small amounts of X rays and gamma rays. The atmosphere blocks some of the visible light and infrared rays, almost all the radio waves and ultraviolet rays, and all the X rays and gamma rays. The term solar energy also refers to energy supplied by solar panels and other devices that use energy from the sun.
Two forms of energy are fundamental—that is, any other form consists of one or both of them. The two forms are: (1) kinetic energy, which is energy of motion; and (2) potential energy, which can be thought of as “stored” energy. For instance, the mechanical energy involved in picking up and dropping a ball is partly potential energy and partly kinetic energy. Pick up the ball, and you give it potential energy. Drop the ball, and the potential energy of the ball turns into kinetic energy.
Energy conversion
Energy can be converted from one form to another. For example, our bodies change chemical energy in food to mechanical energy that we use when we move about. A coal-burning power plant converts chemical energy to electric energy.
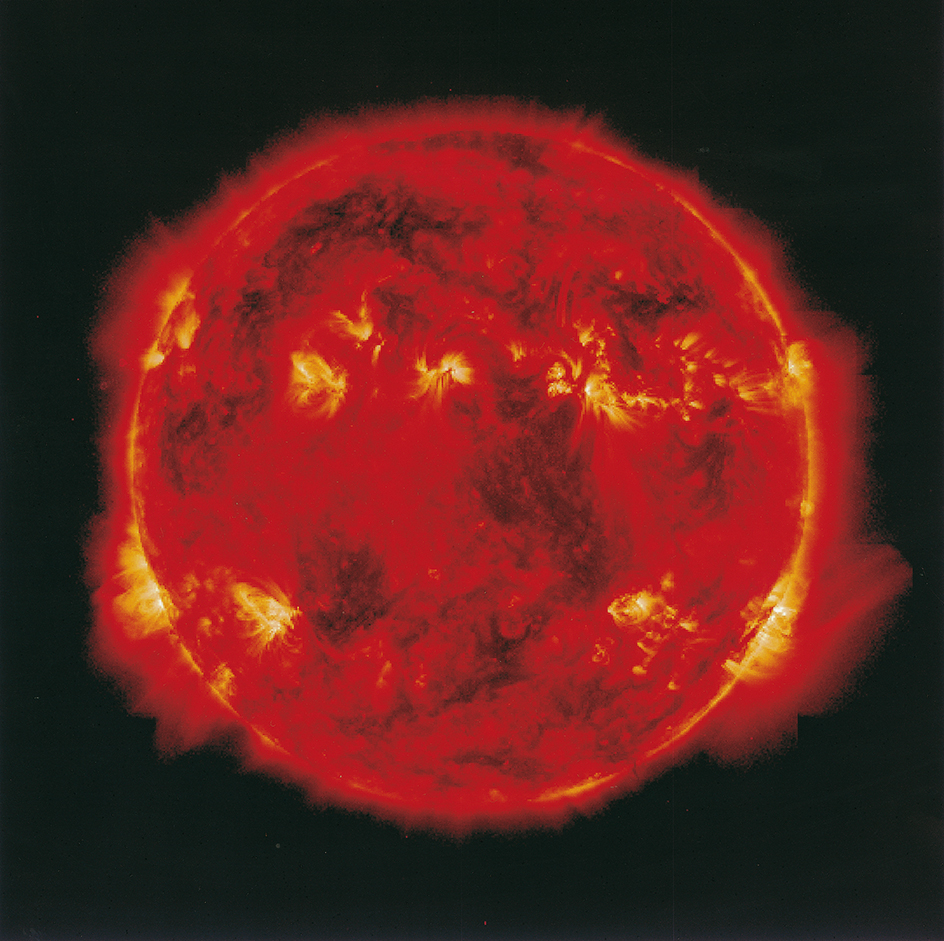
Energy conversion at such a power plant occurs in several stages. The following description of that process may help you understand various forms of energy and how they can be converted.
The coal has potential energy in the form of chemical energy. That energy is stored in electrons of atoms that make up molecules in the coal. Each atom consists of one or more negatively charged electrons in orbit around a positive nucleus. Electrons in neighboring atoms form pairs that bond (join) the atoms together.
When the coal burns, its molecules change as bonds break and other bonds form. As the molecules change, electrons release energy, which is immediately converted to heat energy. Heat energy is the kinetic energy of the atoms and molecules of a solid object, a liquid, or a gas; the hotter an object, the greater the kinetic energy of its atoms and molecules.
In the power plant, the molecular changes that occur when the coal burns create hot gases. In the next stage of the process, heat energy in the gases changes to heat energy in the metal that makes up a boiler, then to heat energy in molecules of water inside the boiler. As the water molecules absorb heat energy, they move more and more rapidly. Eventually, they move so rapidly that water turns to steam.
The steam flows from the boiler through pipes to a device known as a steam turbine. The turbine has several wheels, each with fanlike blades. The steam rushes through the blades, pushing against them and thereby spinning the wheels. In this stage, heat energy of the steam is converted to mechanical energy of the turbine.
The turbine is connected to a machine called an electric generator. In the final stage of the process, the generator converts mechanical energy of the spinning turbine to electric energy (see Electric generator). That energy is partly kinetic and partly potential.
Conservation of energy
In 1847, the British physicist James Prescott Joule demonstrated that mechanical energy can be converted into heat. Soon, scientists learned that any form of energy can be converted to any other form. They also found that, in energy conversions, the total quantity of energy is conserved—that is, the quantity of energy that exists at the end of any process is the same as the quantity that existed when the process began.
Some of the energy at the end of every process is waste energy. For example, in an automobile, much heat energy goes out the exhaust pipe, and friction between parts of the engine creates heat energy that is wasted. But the automobile’s heating system can use waste heat to warm the passengers on cold days.
Energy and matter
Almost 60 years after Joule’s demonstration, the German-born American physicist Albert Einstein expanded the idea of conservation of energy. The expanded idea was an outgrowth of Einstein’s special theory of relativity, published in 1905. Einstein explained that matter can be converted to energy and vice versa. The energy available from a conversion of matter is sometimes called the energy-equivalent of mass. Mass is the amount of matter in an object. An energy-equivalent of mass is potential energy.
The conversion of matter releases energy in the sun and other stars. The sun’s core is so hot that atomic nuclei and electrons move about independently. The most common nucleus is that of the simplest form of hydrogen—a single proton. The protons have so much kinetic energy that they sometimes collide and fuse (join), producing a hydrogen nucleus consisting of a proton and an electrically neutral neutron. But the mass of that nucleus is less than the sum of the masses of a proton and a neutron when they are separate. The “missing matter” has been converted to energy.
Conversion of matter to energy also produces the heat energy that is used to generate electric energy in nuclear power plants. In addition, matter-to-energy conversion provides the tremendous destructive force of nuclear weapons.
Calculating quantities of energy
Scientists use Newtonian equations and relativistic equations to calculate quantities of energy. Newtonian equations are based on Newton’s laws of motion, which were discovered by the English scientist Isaac Newton. The laws were published in 1687 in Philosophiae naturalis principia mathematica (Mathematical Principles of Natural Philosophy), a work usually called simply Principia or Principia mathematica.
Relativistic equations are based on Einstein’s special theory of relativity, and they apply to realms of physics unknown in Newton’s time. For example, scientists use a relativistic equation for the kinetic energy of an object traveling at more than a few percent of the speed of light in a vacuum. (In a vacuum, light travels at 186,282 miles—or 299,792 kilometers—per second.) But a Newtonian equation will give an extremely accurate value of the kinetic energy of an object moving at a speed typical of objects seen in our everyday lives. The equations used in this article are Newtonian.
Units of work and energy.
Due to the relationship between energy and work, energy is measured in units of work. In its scientific sense, work is the movement of an object against a resisting force. For example, a person does work by lifting an object. Lifting an object requires work because the lifter must overcome the force of gravity on the object. The amount of work done, and therefore the amount of energy used, equals that force multiplied by the distance the object is lifted. The kinetic energy used to lift the object is “stored” in the object as potential energy.
In the inch-pound system of units customarily used in the United States, work and energy are commonly measured in foot-pounds. In the International System of Units (SI), the modern metric system, work and energy are measured in units called joules—named for James Prescott Joule. One joule equals 0.738 foot-pound.
Another unit of energy is the thermochemical calorie, which is usually referred to as simply the calorie. This unit is not part of the SI, but scientists commonly use it in studying heat. The calorie equals exactly 4.184 joules. The thermochemical calorie is not the same calorie used in the field of nutrition. Instead, nutritionists use the kilocalorie, which equals 1,000 thermochemical calories. But the kilocalorie is also referred to as the calorie, and sometimes as the Calorie—with a capital “C” to distinguish it from the thermochemical calorie.
Energy and power.
People sometimes confuse energy with power. Power is the rate at which energy is delivered—so power equals energy divided by time. In the inch-pound system, one unit of power is the horsepower, which equals 550 foot-pounds per second. In the SI, the unit for power is the watt, named for the Scottish inventor James Watt. One watt equals 1 joule per second. The watt is much smaller than the horsepower. One kilowatt (1,000 watts) equals 1.341 horsepower.
Weight and mass.
The amount of energy used to lift an object can also be defined as the weight of the object multiplied by the distance the object is lifted. In this definition, weight is used in its scientific and technological sense to mean the force of gravity on the object. But this usage can be confusing because, in commercial and everyday use, weight means mass.
Weight, in the scientific and technological sense, is proportional to mass. The two quantities are related by the equation Fg = mg, where Fg is force due to gravity (that is, weight), m is mass, and g is the acceleration that a falling object undergoes due to gravity. Scientists typically work in metric units. The metric units that apply to this equation are newtons—named for Isaac Newton—for Fg, kilograms for m, and meters per second per second for g. At Earth’s surface, g is about 9.8 meters per second per second.
Suppose you wanted to find the weight of a rock that has a mass of 2 kilograms. You would insert that value and the value of g into the equation and multiply: Fg = (2)(9.8) = 19.6 newtons.
Potential energy of a raised object.
Suppose a person raised the rock to a height of 2 meters. What would be the potential energy of the rock?
The equation for the potential energy of a raised object is Ep = Fg s. In this equation, Ep is energy in joules, Fg is force due to gravity in newtons, and s is the distance the object has been raised in meters. We insert the values of Fg and s into the equation and multiply: Ep = (19.6)(2) = 39.2 joules.
Kinetic energy.
Suppose the person threw the rock at a velocity (speed in a particular direction) of 12 meters per second. What would be the kinetic energy of the rock?
The equation for kinetic energy is Ek = (1/2)mv2. In this equation, Ek is energy in joules, m is mass in kilograms, and v is velocity in meters per second. The 2 beside the v indicates that v is to be squared (multiplied by itself). So the calculation is Ek = (1/2)(2)(12)(12) = 144 joules.