Heat is the energy that flows from a warmer object to a cooler one. When we think of heat, we tend to think of things that feel hot to us, such as fire or a warm summer day. But even cool things can give off heat, and heat plays a much wider role in the world around us.
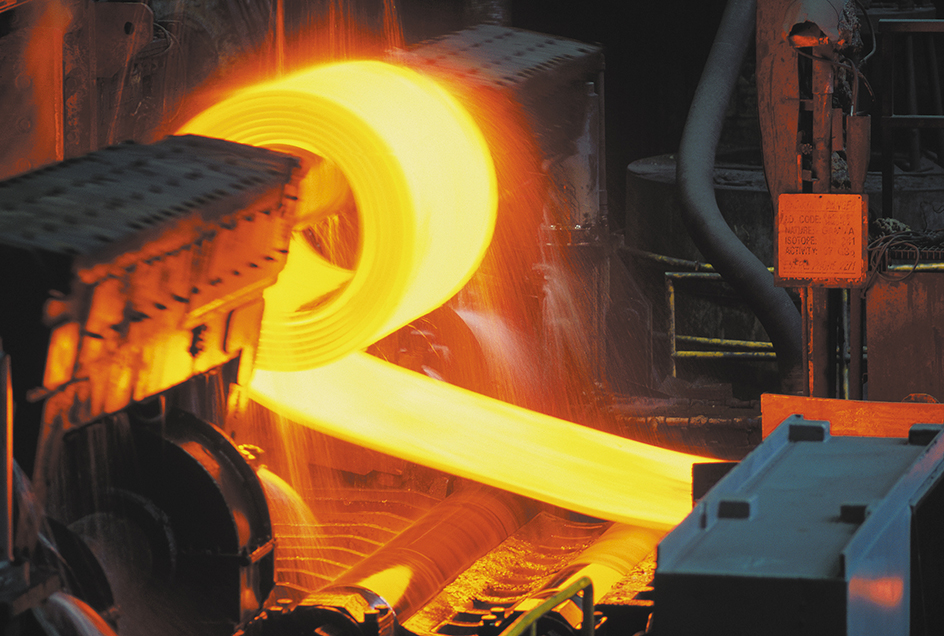
All objects have an energy associated with their temperature. This type of energy is known as internal energy or thermal energy. Heat is simply thermal energy as it moves from a warmer object to a cooler one.
Our bodies must carefully maintain a certain thermal energy or “body heat” to live. We use the food we eat to produce the thermal energy that keeps our temperature at about 98.6 °F (37 °C). If our temperature rises too far above normal—or falls too far below normal—we can die. In cold weather, we wear heavy clothes to hold in thermal energy. During warm weather, we wear light clothes to let unneeded thermal energy escape as heat.
Temperature is a measure of thermal energy. No one knows how high temperatures can climb. The temperature inside the hottest stars is many millions of degrees. The lowest possible temperature, called absolute zero, is –459.67 °F (–273.15 °C).
In our homes, we use heat in many ways. Heat warms our living spaces. It cooks our food. It also provides hot water. Heat dries the laundry.
In industry, heat has almost endless uses. Heat is used to separate metals from their ores. Heat helps refine crude oil. It is used to melt, shape, and harden metals. Heat joins metals together. Heat is also used to make or process foods, glass, and many other products.
Heat and thermal energy also drive our machinery. For example, an engine may burn a fuel, such as gasoline or natural gas, transforming its chemical energy into thermal energy. The engine then converts thermal energy into the mechanical energy that can move an airplane or automobile. The same process can cause the wheels of giant turbines to spin, driving generators that produce electric power. Electric power provides light. It also powers all kinds of equipment—from personal computers to electric trains.
Sources of heat
The heat that we use or that affects life and events on Earth comes from six main sources. They are (1) the sun, (2) Earth, (3) chemical reactions, (4) nuclear energy, (5) friction, and (6) electric power.
We can control some of these sources. Others we cannot. We use the sources we can control, such as electric power and nuclear energy, to heat buildings and do other work. But the sources we cannot control also benefit us. For example, heat from the sun makes life possible. All sources of heat, even those that we normally control, can do damage if they get out of control. For example, fires, which are chemical reactions, destroy much property every year.
The sun
ranks as our most important source of heat. If the sun should ever cool, Earth would become cold and lifeless. Only a tiny fraction of the heat given off by the sun strikes Earth. Yet it provides the warmth that keeps us—and all other living things on Earth—alive.
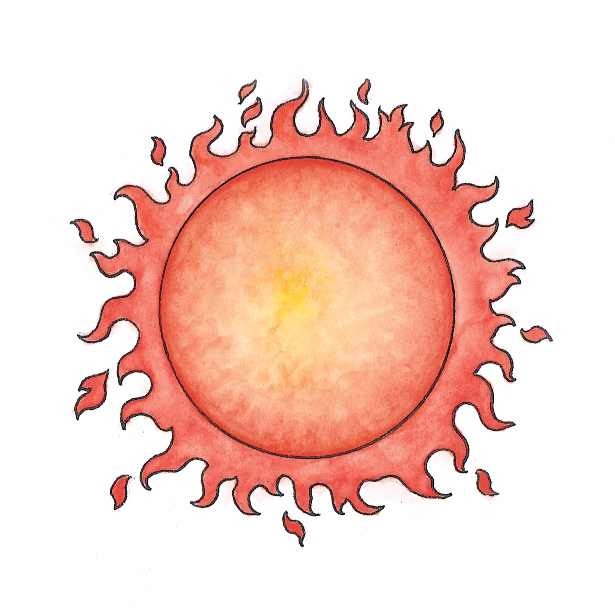
The sun’s heat is absorbed by the seas, the ground, living things, and the atmosphere. Its heat can be collected using such devices as solar furnaces. These furnaces have mirrors that reflect the sun’s rays from a wide area onto one spot. Some solar furnaces can collect enough heat to melt steel. Smaller ones can gather enough heat to cook food.
Earth
itself contains much thermal energy deep inside. When a volcano erupts, some of this energy escapes to the surface. The lava from a volcano is rock melted by the transfer of heat deep within Earth. Some of Earth’s thermal energy also escapes in geysers. These springs shoot forth boiling water that has been heated by hot rocks within Earth. Thermal energy gathered from Earth’s interior is called geothermal energy. It is used to generate electric power and heat houses.
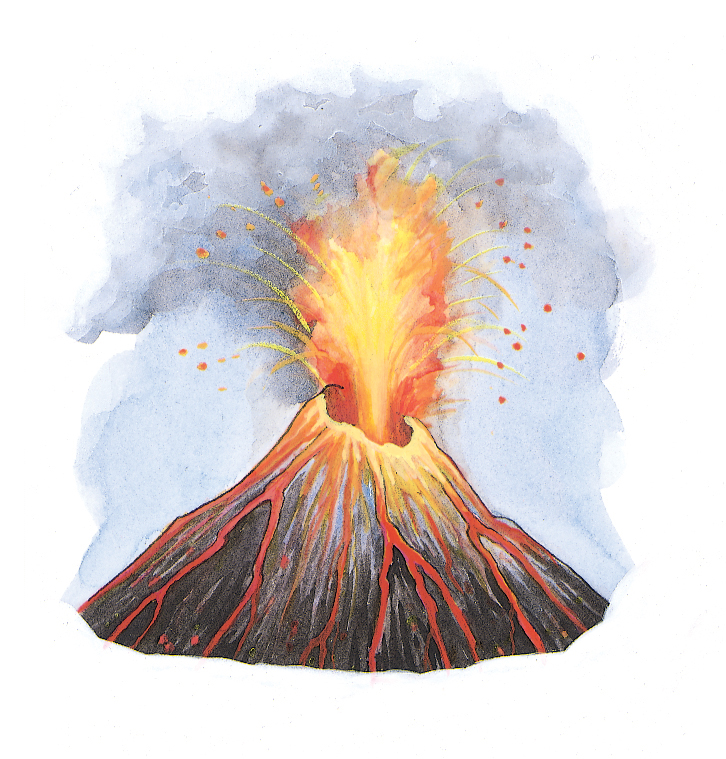
Chemical reactions
can convert chemical energy stored in certain substances into thermal energy. One example is a chemical reaction called oxidation, in which a substance combines with oxygen. Rapid oxidation produces thermal energy readily enough to cause a flame. When coal, wood, or any other fuel burns, substances in the fuel combine with oxygen in the air to form other compounds. This chemical reaction, known as combustion, produces fire. Fires give off a type of heat called thermal radiation.
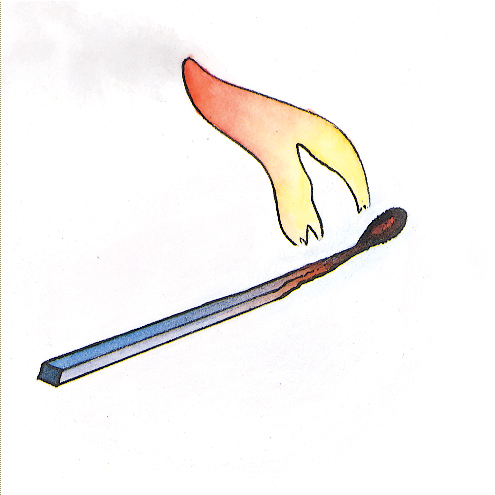
People use fire in many ways. Fire in a gas stove produces heat to cook food. Coal, oil, or gas fires in furnaces and boilers heat buildings. Fire heats metals red-hot so that they can be shaped into a variety of forms. Special cutting torches can produce flames hot enough to cut through metal.
Another example of combustion is the burning of gasoline in an automobile engine. This process produces thermal energy that causes the gases in the engine’s cylinders to expand, moving parts that make the engine work. Only a small portion of the energy produced in an engine is transformed into power. Most of the energy is lost as heat, through the exhaust or through the heating of the engine itself.
The rusting of iron is also an example of oxidation. Unlike fire, however, rusting occurs so slowly that little heat and no flames are produced.
The mixing of certain kinds of chemicals also produces thermal energy and heat. For example, if sulfuric acid and water are rapidly combined, the mixture can become boiling hot.
Living things convert food into energy and living tissue through a set of chemical processes called metabolism. Some of this energy is thermal energy. It may be used to maintain body temperature or released into the environment as heat.
Nuclear energy
can produce great quantities of heat. Nuclear energy comes from splitting or combining the nuclei (cores) of atoms. Nuclear weapons make so much heat so quickly that they destroy virtually everything around them. But in a device called a nuclear reactor, heat can be produced from nuclear energy slowly enough to generate electric power.
Friction.
When one object rubs against another, heat is produced. Friction is usually an unwanted source of heat because it may damage objects. In a machine, for example, the heat created as the moving parts rub against one another may cause those parts to wear down. For this reason, people apply oil between moving machinery parts. The oil reduces friction and so decreases the generation of heat.
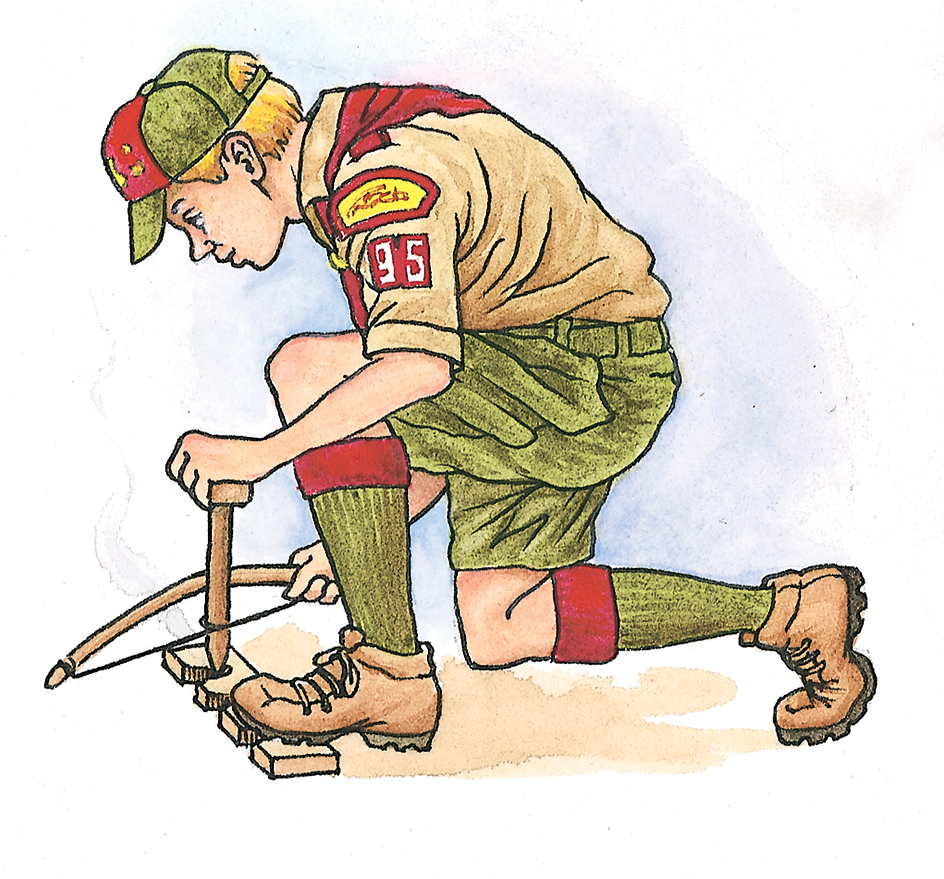
Electric power.
The flow of electric current through metals, alloys, and other conductors (substances that carry electric current) generates heat. People make use of this heat in the operation of many appliances. These appliances include dryers, electric furnaces, heaters, irons, ovens, ranges, and toasters.
What heat is
Heat is the flow of thermal energy from one location to another. Heat and thermal energy cannot be seen, but the work they do can. For example, the burning of fuel in the jet engine of an airplane creates hot gases, which have much thermal energy. The energy causes the gases to expand, providing the power to move the plane.
Temperature and heat.
All things are made up of atoms or molecules, which are always moving. The motion gives every object internal energy. The level of an object’s internal energy depends on how rapidly its atoms or molecules move. If they move slowly, the object has a low level of internal energy. If they move violently, it has a high level of energy. Hot objects have higher internal energy levels than do cold objects. The words hot and cold refer to an object’s temperature.
Temperature is a measure of this internal energy, called thermal energy. A thermometer is used to measure temperature. Thermometers have a numbered scale so that temperature can be expressed in degrees. The two most common scales are the Fahrenheit and the Celsius scales. The Celsius scale is sometimes called the centigrade scale. See Temperature.
The temperature of an object determines whether that object will gain or lose energy when it comes into contact with another object. If a hot rock and a cold rock touch, some of the thermal energy in the hot rock will pass into the cold rock as heat. If a thermometer were placed on the hot rock, it would show the rock’s temperature falling steadily. A thermometer on the cold rock would show a steadily rising temperature. Eventually, the two thermometers would show the same temperature. Then, no further flow of heat would occur.
Just as water flows only downhill, so heat flows only down a “temperature hill.” Heat only passes from an object at a higher temperature to an object at a lower one. The greater the difference in temperature between two objects, the faster the heat will flow between them.
It is important to recognize that temperature and heat are not the same thing. Temperature is simply an indication of the level of thermal energy that an object has. Heat, on the other hand, is the passage of energy from one object to another.
Three units are commonly used to measure heat. They are (1) the calorie, (2) the British thermal unit (Btu), and (3) the joule.
The calorie
is the quantity of heat needed to raise the temperature of 1 gram of water by 1 Celsius degree at standard atmospheric pressure. The calorie is often used in the sciences. Calories are also used to measure the energy content of food. The calorie used in this way is often called a food calorie or written Calorie with a capital C. It is actually equal to 1,000 calories.
The British thermal unit
is the quantity of heat needed to raise the temperature of 1 pound of water by 1 Fahrenheit degree. The Btu is generally used in engineering. One Btu equals about 250 calories.
The joule
can be used for measuring all forms of energy, including heat. One joule is the amount of energy used—or work done—when a force of 1 newton moves an object 1 meter in the direction of the force. One calorie equals about 4 joules.
Disorder.
Temperature and internal energy give us only a partial understanding of heat. For a more complete understanding, we must learn what happens to an object’s atoms or molecules as heat flows into it.
As heat enters an object, the object’s atoms or molecules move around more. The more heat that flows in, the more the atoms or molecules move. The more they move, the more disorderly they become. For example, the water molecules in a snowflake have an orderly pattern. But if a snowflake is taken into a warm room, it will melt and become a drop of water. In water, the molecules move around in a more disorderly manner. Heat thus changes the orderly pattern of the snowflake into a more disordered one. Scientists use the term entropy to describe the amount of disorder in an object.
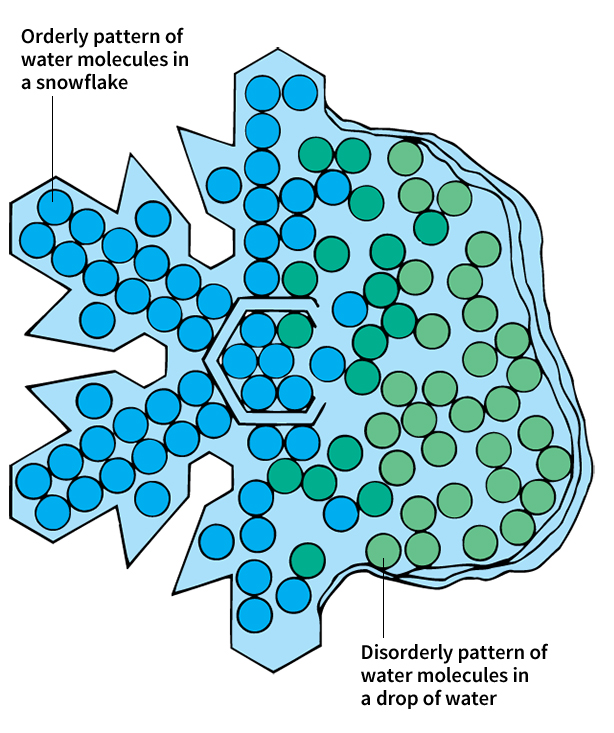
Heat flowing into an object increases the thermal energy and entropy in that object. Usually, the added heat also raises the temperature of the object. On the other hand, heat flowing out of an object decreases the thermal energy and entropy in that object. Usually, the heat loss also lowers the temperature of the object.
How heat travels
Heat passes from one object or place to another by three means. They are (1) conduction, (2) convection, and (3) radiation. Insulation reduces the flow of heat.
Conduction
is the spreading of thermal energy through a material by collisions between atoms. When energy travels by conduction, it moves through a material without carrying any of the material with it. For example, the end of a copper rod placed in a fire quickly becomes hot. The atoms in the hot end begin to vibrate faster and strike neighboring atoms. These atoms then vibrate faster and strike adjoining atoms. In this way, the energy travels from atom to atom until it reaches the other end of the rod. But during the process, the atoms themselves do not move from one end to the other.
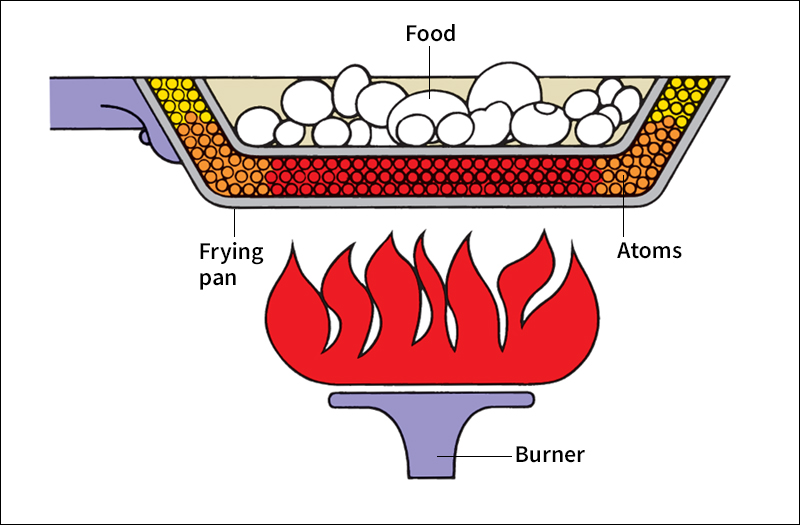
Convection
is the transfer of thermal energy by the circulation of a liquid or gas. For example, a hot stove in a room heats the air around it by convection. The warmed air expands and so is lighter than the colder air surrounding it. The warm air rises, and cooler air replaces it. Then the cooler air near the stove becomes warm and rises. This movement of warm air away from a hot object and the flow of cooler air toward that object is called a natural convection current. The current of air carries thermal energy to all parts of the room.
Convection occurs in liquids as well as in gases. For example, convection currents will form in a pan of cold water on a hot stove. As the water near the bottom of the pan warms and expands, it becomes lighter than the cold water near the top. This cold water sinks and forces the heated water to the top. The convection current continues until all the water reaches the same temperature.
Engineers can use forced convection to increase heat transfer. In forced convection, a device such as a compressor, fan, or pump increases the motion of a fluid or gas. The faster-moving substance exchanges more heat than it would through natural convection alone. Forced convection is used in machines called heat exchangers.
Radiation.
In conduction and convection, moving particles transmit heat. But in radiation, heat can travel through a vacuum, which has no particles. In any object, the moving atoms or molecules give off heat as thermal radiation. Much of this radiation is in the form of infrared rays. Hot objects give off more infrared rays than do cold objects. Infrared rays travel through space as packets of energy called photons. When the radiation strikes an object, it speeds up the atoms or molecules in that object. Thermal radiation from the sun, for example, travels through space and warms Earth’s surface.
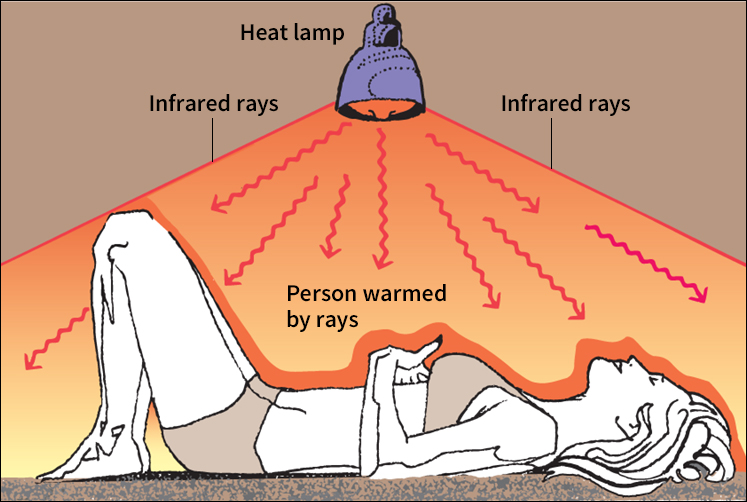
Insulation
is a way to control the movement of thermal energy by keeping it in or out of a place. For example, houses are insulated to keep the heat inside in winter and outside in summer. People use three methods of insulation because thermal energy can travel in any one of three ways.
Certain materials, such as plastic and wood, make good insulators against heat conduction. This fact explains why many pots and pans have plastic or wood handles. The metal utensil itself heats rapidly by conduction. But insulation keeps the handle cool.
The spread of thermal energy through the air by convection can be controlled by blocking the space between a hot and cold area with a region of air that cannot circulate readily, sometimes called “dead air space.” For example, the layer of air between a storm window and the inner window acts as an insulator.
Surfaces that reflect infrared rays can insulate a space against heat traveling by radiation. For example, shiny metal roofs reflect the sun’s rays.
The effects of heat
When heat passes into or out of a substance, the substance’s thermal energy changes. Heat may also change the temperature, size, and state of the substance.
Changes in temperature
are a common result of heat. The specific heat capacity of a substance is the amount of heat needed to raise the temperature of the substance by a certain temperature. It is often expressed as the number of calories or joules needed to raise 1 gram of a substance 1 degree Celsius.
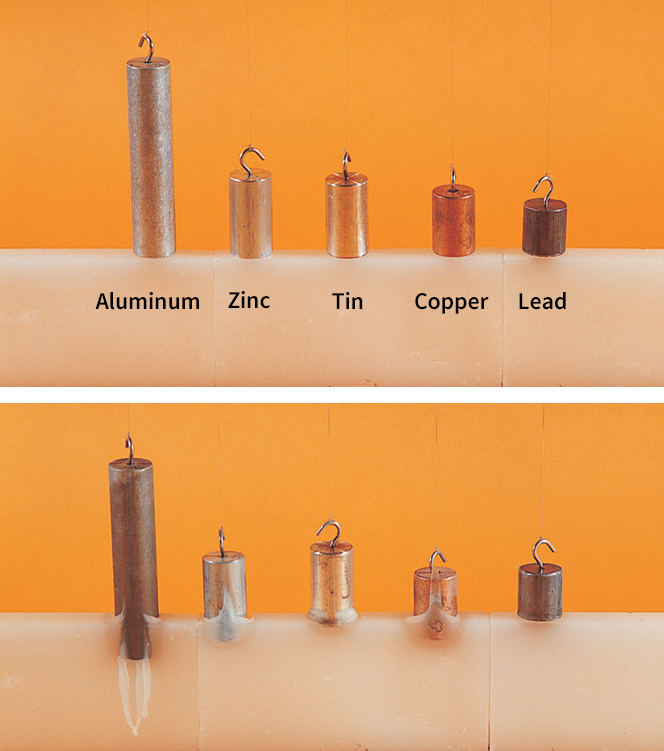
Equal masses (amounts of matter) of two substances with different specific heat capacities require different amounts of heat to reach the same temperature. If both substances receive the same amount of heat, the temperature of the substance with a low specific heat capacity will increase more than will that of the substance with a high specific heat capacity. For example, it takes 10 calories of heat to raise 1 gram of water 10 Celsius degrees. But 10 calories will raise the temperature of 1 gram of copper 111 degrees. Copper has a much lower specific heat capacity of 0.09 compared with 1 for water.
Changes in size.
When a substance’s thermal energy increases, the motion of the atoms or molecules in the substance increases. As a result of their increased motion, the atoms or molecules take up more space, and the substance expands. The opposite occurs when thermal energy decreases. The atoms or molecules move more slowly. They therefore take up less space, and the substance contracts.
All gases and most liquids and solids expand when heated. But they do not expand equally. If a gas, a liquid, and a solid receive enough heat to raise their temperatures the same amount, the gas will expand the most, the liquid will expand much less, and the solid will expand the least.
Thermometers, thermostats, and many other devices work on the principle of expansion and contraction. Many thermometers contain a liquid, such as alcohol or mercury, that expands and contracts evenly as the temperature changes. A rise or fall in temperature causes the volume of the liquid to expand or contract only slightly. But because the liquid is in a narrow glass tube, the tiny change in volume results in a noticeable change in the height of the column of liquid.
Changes in temperature also cause expansion and contraction in the materials that are used in bridges, buildings, and other structures. This expansion and contraction can cause problems if the builders do not allow for it. For example, the steel beams used in a building will bend or break if they do not have room to expand. For this reason, structures have expansion joints. Such joints allow extra space for the materials to expand and contract without damage as temperature changes.
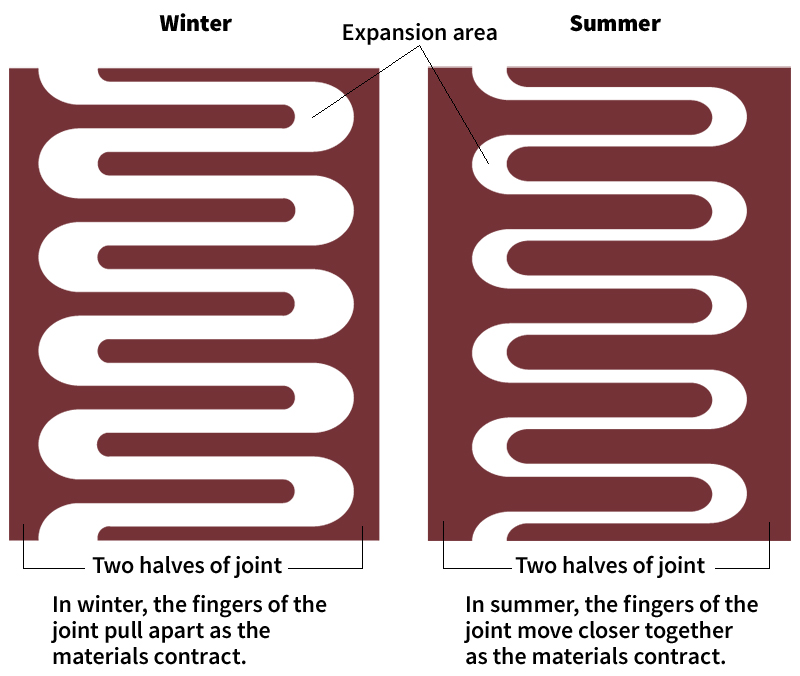
Engineers can often determine by how much the length of an object will increase as its temperature rises. To do so, they need to know the material’s coefficient of linear expansion. The coefficient indicates how much longer the material will become if its temperature increases by 1 Celsius degree. For iron, the coefficient of linear expansion at room temperature is 0.000012. Thus, each meter of an iron bar becomes 12 microns (millionths of a meter) longer with each degree Celsius increase in its temperature.
Changes in state.
Ordinarily, the temperature of an object rises as heat flows into it. But under certain circumstances, the addition of heat causes no increase in an object’s temperature. Instead, the disorder of the atoms or molecules in the object increases, causing the material to change state.
If heat is added to a block of ice that is colder than 0 °C, the temperature of the ice will rise until it reaches 0 °C, ice’s melting point. Then the temperature will stop increasing for a time, even if more heat flows into the ice. The additional heat will increase the disorder of the molecules in the ice, causing the ice to melt. Until all the ice has melted, the water will remain at 0 °C. The heat needed to change ice to water is called the heat of fusion. Each gram of ice at 0 °C requires 80 calories of energy to melt it to water at the same temperature.
As more heat flows into the water at 0 °C, the temperature of the water will again rise until it reaches 100 °C, water’s boiling point. Under normal atmospheric pressure, additional heat will not raise the temperature any further. Instead, some of the water will change into steam. Not until all the water has become steam will additional heat cause the temperature to rise again. The heat needed to change water at 100 °C into steam at the same temperature is called the heat of vaporization. Each gram of water at 100 °C takes 540 calories of energy to become steam. Once all the water is steam, more heat will raise its temperature above 100 °C.
A liquid can also become a gas at a temperature below its boiling point, through a process called evaporation. Evaporation occurs at the surface of a liquid. The molecules at the surface break free from those below and enter the air as a gas. The speed at which evaporation occurs depends on the kind of liquid, its temperature, and the amount of gas present above the liquid.
The energy used to change a material from a solid to a liquid or from a liquid to a gas is known as latent heat. Latent heat must be removed to change a gas back to a liquid or a liquid back to a solid. That is, 540 calories of energy must be removed from each gram of steam at 100 °C to turn it to water. This process is called condensation. Eighty calories must be removed from each gram of water at 0 °C to produce ice, the process of freezing. The boiling and condensation points of a substance are at the same temperature, as are the melting and freezing points.
Latent heat may also be associated with changes in the structure of the crystals that make up a solid. In general, much less latent heat is needed for such changes than for melting or vaporization.
Working with heat
Many devices work with heat to perform a useful function. Some devices change heat into motion. Others remove unwanted heat, providing refrigeration.
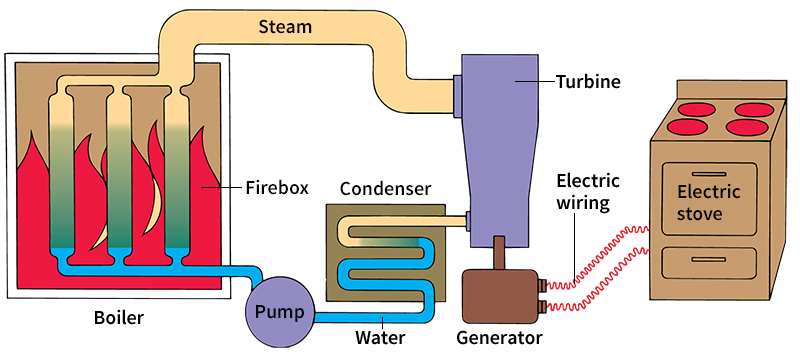
Changing heat into motion.
Mechanical energy can be converted into thermal energy, and vice versa. For example, friction between moving parts in a machine can transform mechanical energy into thermal energy. Devices called heat engines, on the other hand, change thermal energy into mechanical energy.
Heat engines can be divided into two groups. They are (1) external-combustion engines and (2) internal-combustion engines.
External-combustion engines
produce hot gases that transfer heat to another fluid. It is the thermal energy in this fluid that is changed into mechanical energy. External-combustion engines include gas and steam turbines and reciprocating steam engines.
A steam turbine is a good example of an external-combustion engine. Heat from burning fuel or from a nuclear reactor changes water in a boiler to steam. Pipes carry the steam into the turbine. The turbine has a series of bladed wheels attached to a shaft. The high-temperature steam expands as it rushes through the turbine. The expanding steam pushes on the blades, causing them to turn the shaft. The spinning shaft can drive an electric generator, move a ship’s propeller, or do other work.
Internal-combustion engines
produce hot gases whose thermal energy is changed directly into mechanical energy. The thermal energy is not transferred to another fluid.
The gasoline engine in an automobile is a good example of an internal-combustion engine. The burning of gasoline in the cylinders of the engine produces hot gases. The gases expand, pushing against the pistons in the cylinders. The motion of the pistons moves other parts of the car that turn the car’s wheels.
Refrigeration.
The simplest way to lower the temperature of an object is to bring it into contact with a colder object. The temperature difference causes heat flow from the warmer object into the colder one. For example, ice put in an insulated chest keeps food cold by drawing heat from the food.
Sometimes, however, a colder object is not available. The most common way to remove heat from an object without using a colder object is mechanical refrigeration. Mechanical refrigeration works by changing a substance, called a refrigerant, from a gas to a liquid and back to a gas again. In a refrigerator, for example, a compressor squeezes a gaseous refrigerant into a small space. The resulting increase in pressure raises the temperature of the refrigerant. The hot gas then flows through a system of pipes. These pipes allow the gas to give off heat to the cooler air around them. As the gas cools under high pressure, it condenses into a liquid. The compressed liquid then expands through a valve leading to pipes in parts of the refrigerator that are insulated. As the gas expands, its pressure falls. This causes the temperature of the refrigerant to fall. The cooler refrigerant draws heat from the air and food inside of the refrigerator, lowering the temperature of the contents and warming the refrigerant. Under lower pressure, the warmed refrigerant once again becomes a gas. The gas flows through pipes back to the compressor. There, the cycle begins again, providing continuous refrigeration.
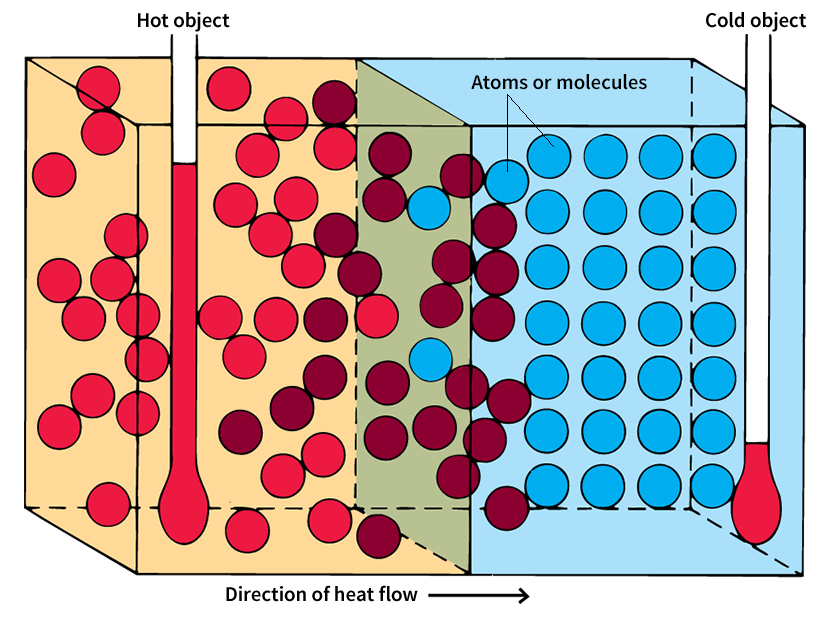
Theoretically, the lowest temperature to which a substance can be refrigerated is absolute zero (–459.67 °F or –273.15 °C). At this temperature, matter would have the least possible internal energy and almost no disorder.
The study of heat
Until the late 1700’s, many scientists believed that heat was an invisible fluid called caloric. This idea is called the caloric theory. Scientists thought an object became warm when caloric flowed into it and grew cold when caloric flowed out of it. Because objects weighed the same whether they were hot or cold, the scientists reasoned that caloric had no weight and therefore could not be a kind of matter.
Near the end of the 1700’s, the work of two scholars raised questions about heat that the caloric theory could not answer. These scholars were the American-born scientist Benjamin Thompson and the British chemist Sir Humphry Davy.
In 1798, Thompson, also known as Count Rumford, observed the manufacture of cannons in Munich, Germany. He noted that the drills used to bore the cannons produced frictional heat even after the drills became dull and no longer cut out metal. The caloric theory could not account for the release of heat unless a drill actually cut metal. Yet an unlimited quantity of heat resulted as long as a drill turned against metal.
In 1799, Davy melted two pieces of ice by rubbing them together in a container at a temperature below the freezing point of water. Again, the caloric theory could not account for the heat produced. Thompson’s and Davy’s observations raised doubts about the caloric theory. But no one proposed another explanation of heat.
Heat and energy.
The idea that heat is a form of energy was proved during the mid-1800’s. The proof was developed largely by three scholars. They were Julius Robert von Mayer, a German physician and physicist; Hermann von Helmholtz, a German physicist; and James Prescott Joule, a British physicist.
Mayer observed that people in warm and cold climates needed different amounts of energy from food to maintain normal body temperature. He presented his findings in 1842. But his ideas did not receive scientific recognition for many years. In 1847, Helmholtz completed a work on heat and energy. He stated that heat is a form of energy. The idea won rapid acceptance.
During the 1840’s, Joule measured the amount of mechanical energy needed to raise the temperature of a certain quantity of water. The relationship between mechanical energy and heat energy is called the mechanical equivalent of heat. Joule’s early experiments showed that 838 foot-pounds of mechanical energy produced 1 Btu of heat. Later scientists made more precise measurements. They found that the mechanical equivalent of heat is 778 foot-pounds per Btu. In the metric system, it is 4.184 joules per calorie. The joule was named for James Prescott Joule.
Thermodynamics
is the study of the relationship between heat and other forms of energy. It is based on three laws (principles).
The first law of thermodynamics
is also known as the law of conservation of energy. It states that energy is never created or destroyed. Energy may change form, for example from thermal energy to the mechanical energy of motion. But the total quantity of energy in any closed system remains the same. A closed system is a group of objects that cannot exchange energy with its surroundings.
The second law of thermodynamics
holds that all spontaneous events act to increase the entropy within a closed system. Spontaneous events are those that occur without forcing or help. Under this law, a closed system’s overall entropy increases as the system performs work. Once the system reaches its maximum entropy, it can no longer do useful work.
The third law of thermodynamics
states that the entropy of a perfect crystal approaches zero as the absolute temperature of the crystal approaches zero. One consequence of this law is that it is impossible to reduce the temperature of any system to absolute zero.