Magnetism is a force that causes magnets and certain other objects to attract or repel (push away) each other. Magnets have two regions called poles. The two poles are called the north pole and a south pole. A basic property of magnets is that poles that are alike, such as two north poles, repel each other. Unlike poles, such as a north pole and a south pole, attract each other. As a result, when one magnet is near another, the magnets may show attraction (pulling together) or repulsion (pushing apart), depending on how their poles line up.
Magnetism is closely related to electricity. In fact, the force of magnetism is created by electric current. The force acts only on materials or substances that also carry a current. The charges may move on a small scale, as in the motion of charges inside an ordinary magnet. The charges may also move on a large scale, such as a current running through a loop of wire. A magnet produced by an electric current is called an electromagnet.
Magnets have a variety of uses and may come in many shapes. Magnets attract certain metals, making magnets useful in fasteners and latches. Electric tools, appliances, and trains require magnets to run their electric motors. All electric motors rely on an electromagnet spinning in the force of a stationary magnet. Huge electromagnets are used to pick up and move iron and steel scrap. Magnets in telephones, radios, and TV sets help to change electrical impulses into sounds. Most computer hard drives rely on magnets to record, store, and retrieve information. Many credit cards and passports hold information encoded on magnetic strips. Scientists use powerful electromagnets to hold hot electrically charged substances in nuclear research and to cool things to extremely low temperatures. Hundreds of electromagnets are also used to speed up and collide particles of matter in machines called particle accelerators.
Some rocks, minerals, and meteorites are natural magnets. Earth is a giant magnet, as are the sun and other stars and most of the planets in the solar system. Some insects, birds, and fish have tiny magnets in their bodies. Biologists think these magnets may help animals find their way when migrating.
People in ancient Greece and China independently discovered magnetism when they found that the mineral magnetite attracted iron. Scientists could not explain what caused magnetism, however, until the mid-1800’s. They did not understand it well until the 1900’s.
Together, electricity and magnetism make up a fundamental force called electromagnetism. Electromagnetic energy travels in the form of electromagnetic radiation. Visible light and radio waves are two familiar forms of electromagnetic radiation.
Magnets and their properties
Magnets are most commonly known for the simple ability to attract certain metals or to attract or repel other magnets. This ability follows certain basic rules.
Magnetic poles.
A magnet that can rotate freely, such as one suspended by a string, will align itself with one end pointing north and the other end pointing south. This happens because Earth itself is a large magnet, with its own magnetic force. The end of the magnet that aligns with north is called its north pole. The opposite end is called its south pole. A compass needle points north because it is a small magnet that aligns itself to the magnetic force of Earth.
Attraction and repulsion.
Magnetism causes unlike magnetic poles to attract each other and like poles to repel each other. If the north pole of a magnet comes near the south pole of another magnet, the magnetic force pulls the magnets together. But two north poles or two south poles repel each other.
Magnetization.
Magnets not only are attracted to other magnets but also can attract some materials that are not initially magnets. For example, a steel nail is not a magnet. But placed in contact with a strong magnet, the nail can become magnetic. This process is called magnetization. An object that has been magnetized, such as a nail, has its own magnetic field and can attract or repel other magnets through its magnetic force. Not all materials can be easily magnetized. Almost all magnetized materials lose their magnetization over time.
Magnetic fields.
The region around a magnet where the force of its magnetism affects other magnets is called the magnet’s magnetic field. The field is invisible to human eyes. One way to actually “see” the field is to place a piece of paper over a magnet and sprinkle iron filings on the paper. The filings are tiny magnets that respond to the magnetic force at each point where they land by aligning with that force. They bunch together near the poles and form a pattern around the magnet that corresponds to its magnetic field. The lines of this pattern are called magnetic field lines. They point outward from the north pole of a magnet, loop around, and return to the magnet at its south pole. The lines lie closest to one another near the poles, where the magnetic field is strongest.
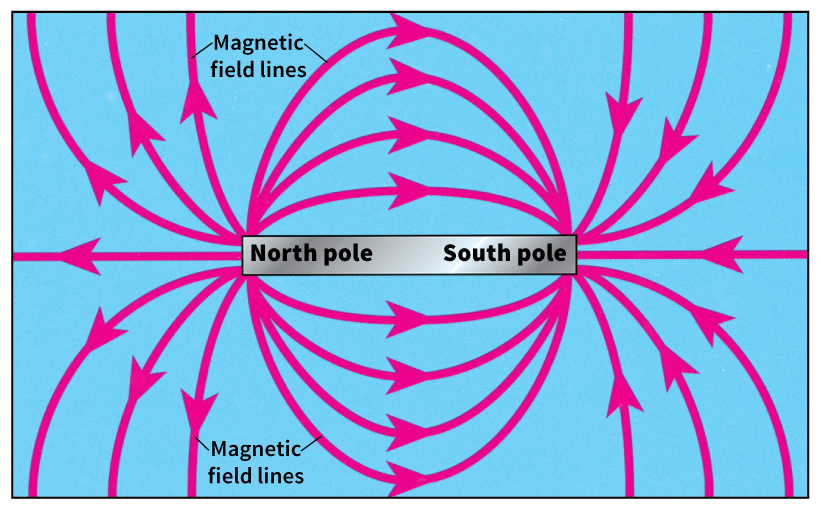
Strength of a magnetic field.
The strength of a magnetic field is measured in units called gauss or tesla. One tesla equals 10,000 gauss. Earth’s magnetic field at the planet’s surface is about 1/2 gauss. The field near the poles of a standard refrigerator magnet may be as high as a few hundred gauss. Fields of magnets used in industry may measure more than 20,000 gauss (2 tesla).
Electromagnetic fields.
When a magnetic field moves in combination with an electric field, the result is called an electromagnetic field. The two fields travel together as electromagnetic radiation, also called electromagnetic waves. Visible light is a type of electromagnetic wave. So are X rays, microwaves, and radio waves. Cell phones, wireless Internet connections, satellite TV, and radio all use electromagnetic waves invisible to the human eye to transmit and receive signals. Human eyes detect waves of light, resulting in our sense of vision.
Kinds of magnets
Objects can be divided into several different categories based on their magnetic properties. Temporary magnets can be magnetized in the presence of a magnetic field. Permanent magnets remain magnetic at all times. Most objects made of aluminum, concrete, copper, cotton, glass, gold, paper, plastic, rubber, silver, or wood are considered nonmagnetic. Magnets do not noticeably repel nor attract these substances. Magnetic fields do not easily cause them to become magnetic.
Temporary magnets
are made of such materials as steel and nickel. These materials are known as “soft” magnetic materials because they usually do not retain their magnetism outside a strong magnetic field. For example, a magnetized steel nail loses its magnetism if it is removed from a magnetic field.
Permanent magnets
keep their magnetism long after they have been magnetized. For this reason, they are known as “hard” magnetic materials. The earliest known permanent magnet is magnetite, also called lodestone. Magnetite is a combination of iron and oxygen. Many strong permanent magnets are alloys (mixtures) of iron combined with nickel, cobalt, or a group of metals known as lanthanide elements. Among the most common magnetic alloys are a group of compounds containing a mixture of aluminum, cobalt, copper, iron, nickel, and possibly titanium. These compounds are called Alnico alloys. One of the strongest magnets known today is a combination of iron, boron, and the lanthanide element neodymium. Another strong magnet is composed of samarium-cobalt, a mixture of cobalt and the lanthanide element samarium.
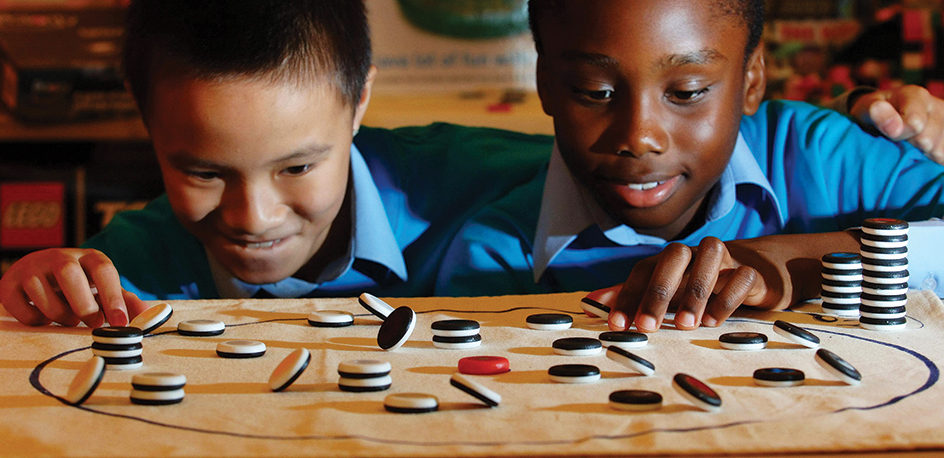
Some soft magnetic materials can be made into weak permanent magnets. For example, you can permanently magnetize the needle of a compass by stroking it in one direction with a magnet.
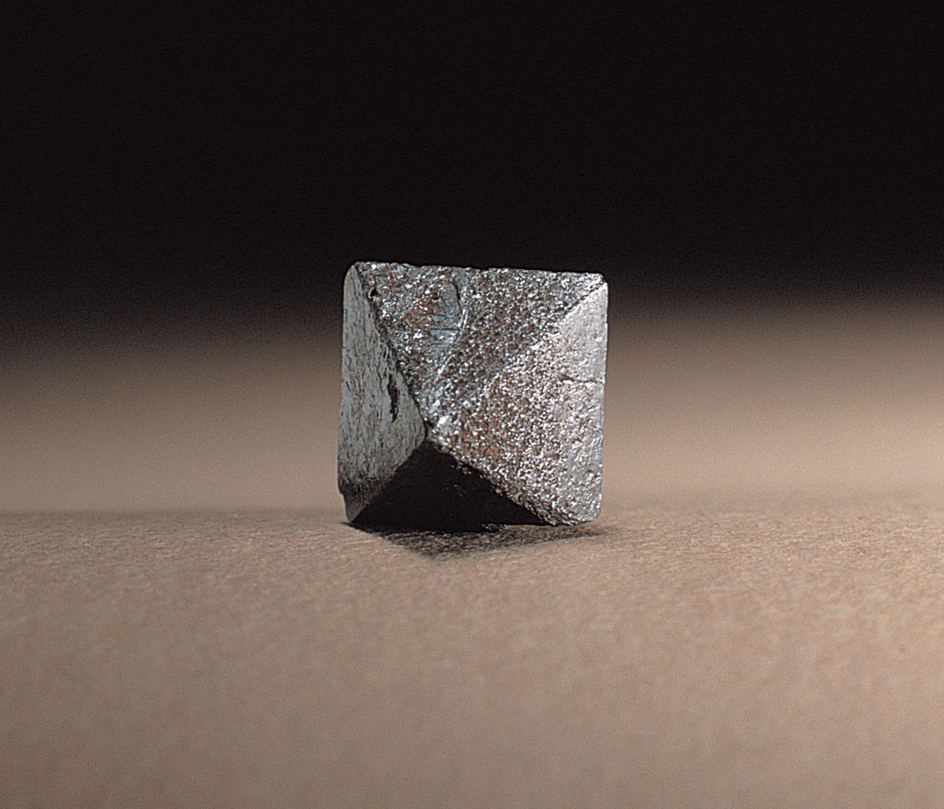
Electromagnets
are produced by electric current. The simplest electromagnets consist of a current flowing through a cylindrical coil of wire called a solenoid. One end of the solenoid becomes the north pole of the electromagnet. The other end becomes the south pole. The poles switch position if the direction of the current is reversed. If the current is stopped, the solenoid loses its magnetism. This characteristic of electromagnets makes them useful as switches. More importantly, it enables them to be used in electric motors. An electric motor has one or more electromagnets that cycle between being on and off. Each time an electromagnet cycles on, its magnetism can help turn a moving wheel.
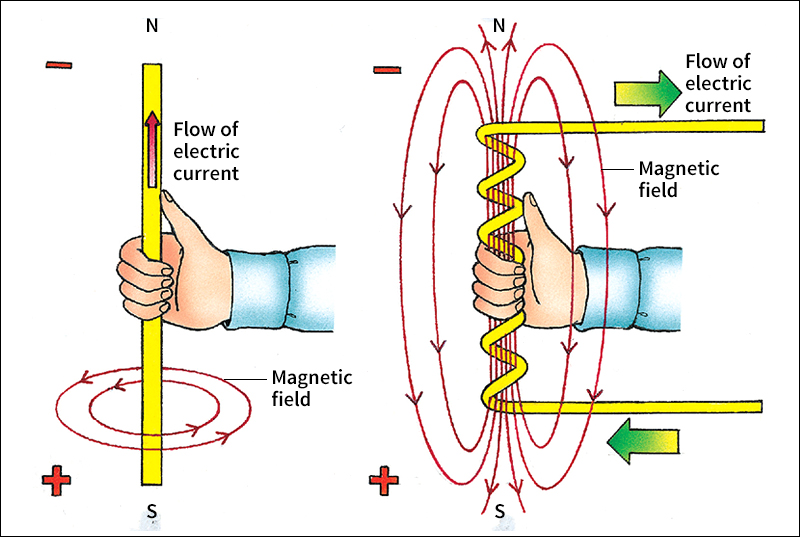
The strength of an electromagnet depends on the number of windings in the coil and the strength of the electric current. More windings and stronger current produce more intense magnetic fields. To further increase the strength of an electromagnet, a cylinder of a soft magnetic material, such as iron, can be placed inside the coil. The electromagnet then magnetizes the iron, and the two fields combine into one.
Uses of magnets
Many small household and large industrial machines make use of magnets. Magnets are also in the form of a magnetic strip on cards such as credit cards and identification cards. All three varieties—permanent, temporary, and electromagnets—are used in various ways.
In the home,
the attractive force between magnets makes them useful as latches on cabinet and refrigerator doors and as holders for knives or other cooking equipment. Magnets also serve as fasteners for holding papers on refrigerators and other metal surfaces. The most important use of magnets in the home, however, is in electric motors. All electric motors use electromagnets or a combination of electromagnets and permanent magnets. These motors run such machines as air conditioners, blenders, DVD players, hair dryers, refrigerators, vacuum cleaners, video game console and computer drives, and washing machines. They also power such tools as drills, hedge trimmers, and sanders. Any motorized device that is plugged into an electric socket or runs on battery power contains a magnetically driven motor. Magnets are also used in speakers to turn electric current into sound.
The hard drives of many computers store information by magnetizing a disk of magnetic material with a certain pattern. Every piece of information, such as words, pictures, or music, is encoded within the pattern. Another magnet “reads” the pattern to retrieve the information from the disk.
In industry and business.
Magnets in electric motors help run almost any machine that makes something move or rotate. Such devices include cranes, fax machines, machine tools, and photocopiers. Powerful electromagnets attached to cranes move scrap iron and steel. They also separate metals for recycling.
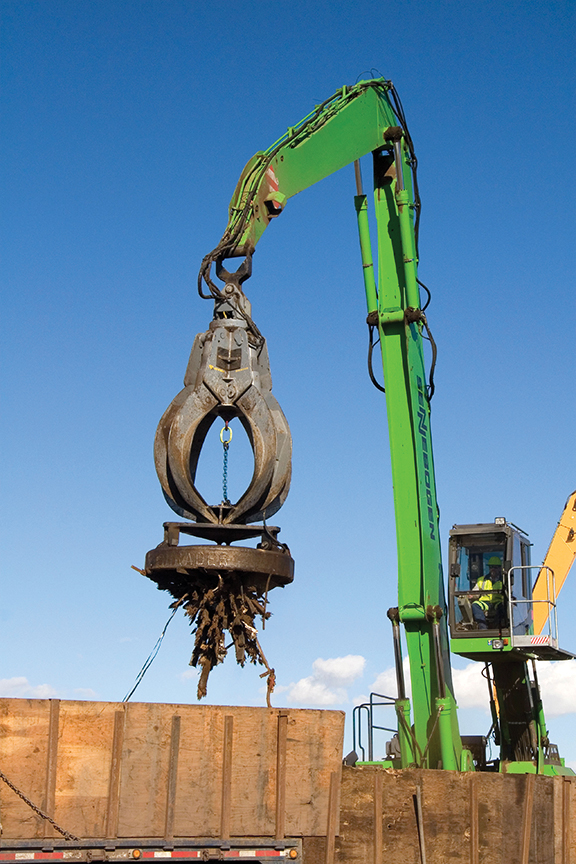
One of the most important uses of magnets is in electric power production. Generators in power plants rely on magnets to produce electric power. Generators have a similar construction to an electric motor. But instead of turning electric power into motion, a generator turns motion into electric power. Devices called transformers use electromagnets to change the high-voltage electric power carried by power lines to the lower voltage used in homes and businesses.
In identification.
Many ATM cards, credit cards, drivers’ licenses, gift cards, and passports contain a magnetic strip that stores information, much like the hard drives on many computers. A device with another magnet reads the information by decoding the magnetic pattern on the strip.
In transportation.
All electrified transportation systems depend on magnets in electric motors. These systems include cable cars, elevators, escalators, monorails, moving sidewalks, subways, trains, and trolleys. In automobiles, buses, and airplanes, electric motors operate door locks, electric windows and doors, windshield wipers, and other devices. Electromagnets also produce radio waves in radar systems, an important navigation aid for ships and airplanes.
Scientists and engineers have developed trains that use electromagnets to levitate (float) above a track without touching it. These trains, called magnetic levitation or maglev trains, eliminate the friction of wheels on the track. Thus, the trains can move at much higher speeds than ordinary trains do.
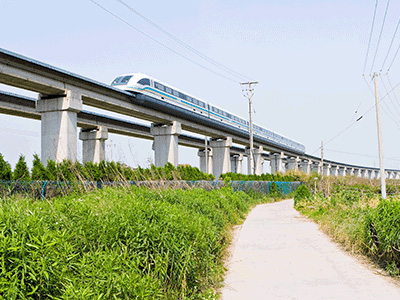
Cars that run on electric power are increasingly common. Some cars use conventional gas motors along with electric motors. These vehicles are called hybrid cars. Other cars are entirely run by electric motors. Both types of engines require electromagnets to operate.
In science and medicine.
Magnets and magnetic fields are widely used in scientific research. In electron microscopes, electromagnets focus a beam of electrons on a sample to be studied. Powerful magnets called bending magnets help control beams of atomic particles that have been boosted to high speed in particle accelerators. In nuclear energy research, physicists use magnetic fields to contain _plasmas—_hot electrically charged substances that behave much like gases. Magnets suspend the plasma away from the sides of the container. Otherwise, the heat from the plasma would melt any material it touched.
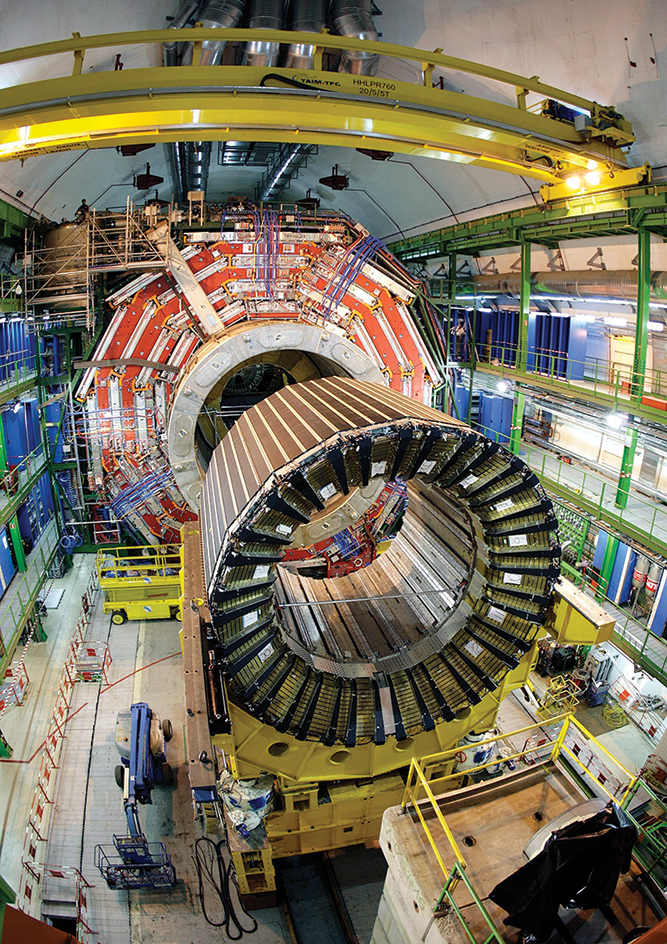
In medicine, many devices for diagnosing diseases use magnets. In a technique known as magnetic resonance imaging (MRI), the patient lies inside a large cylindrical magnet. MRI uses magnetic fields and radio waves to produce images of the head, spine, internal organs, and other body parts. Other diagnostic devices enable physicians to observe magnetic fields generated by the brain, heart, and other internal organs.
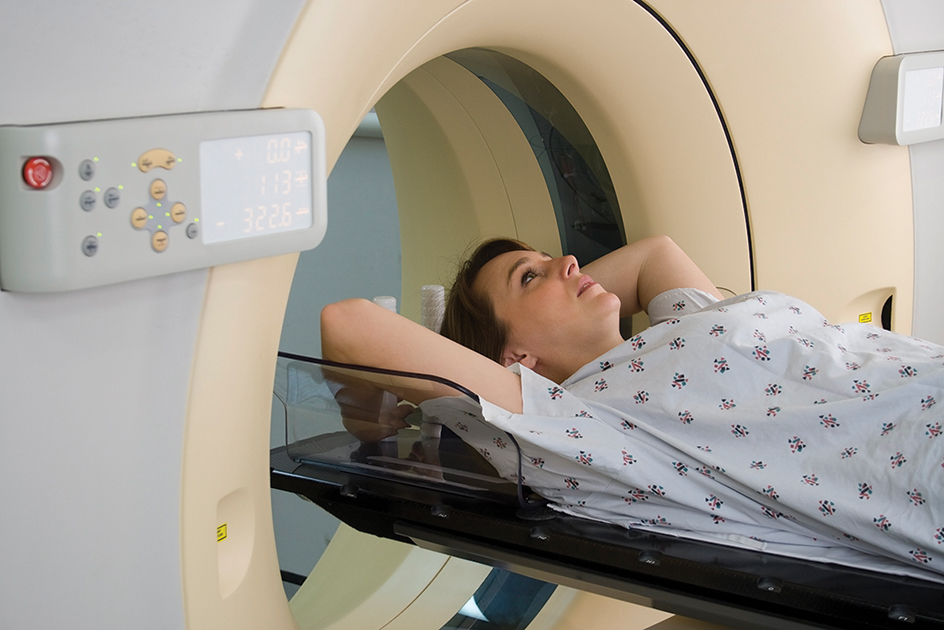
How magnetism works
Magnetism in a material results from magnetic fields produced within the material’s atoms. Atoms are the simple particles that make up common materials. However, not all atoms behave in such a way as to produce a magnetic field.
In atoms.
An atom has a small, dense center called a nucleus. The nucleus consists of protons, which have a positive electric charge, and neutrons, which have no charge. One or more lighter, negatively charged particles called electrons surround the nucleus. The negative electrons orbit the positively charged nucleus much as the planets of the solar system circle the sun. The orbits of electrons around the nucleus are more complicated than the revolution and rotation of the planets. But they bear some similarities. While not on the same scale as the orbits of electrons, protons also revolve within the smaller confines of the nucleus. Both electrons and protons also rotate or spin around their own axis.
Because a moving charge creates a magnetic field, electrons and protons create magnetism in two ways. First, the movement of an electron around the nucleus creates a field. This field is called the orbital magnetic field. Second, the spinning of the charged electron creates another field, called the spin magnetic field. These fields can add together or cancel each other out. All atoms except for the simplest atoms of the element hydrogen have more than one electron. For this reason, the fields from different electrons can cancel one another out or add together to make the magnetism stronger.
Protons can create magnetic fields in ways similar to electrons. Although the movement is confined to the nucleus, the motion of protons produces a magnetic field. Protons also produce a field by spinning on their axis. As with electrons, the fields from different protons can cancel one another out or add together. However, in most atoms, the magnetic fields produced by a proton are relatively weak compared to those produced by an electron. Therefore, electrons are generally more important than protons when determining the magnetic properties of a substance.
In some chemical elements, such as the group of elements known as the noble gases, each atom has a highly balanced configuration of electrons. In such atoms, the magnetic fields from the various electrons’ spins and orbits cancel entirely. As a result, these elements are nonmagnetic. Other elements, mostly metals, tend to have atoms with only partially canceled magnetic fields. These atoms therefore act like tiny magnets. The small magnetic field created by such an atom is called an atomic dipole or atomic dipole moment.
In materials.
All materials react to a magnetic field. However, only materials with a relatively strong dipole moment are generally considered a magnetic material. The materials that contain magnetic dipoles come in many forms, each form depending on how the dipoles point relative to each other.
Some substances have atomic dipoles that point in all different directions These randomly arranged dipoles cancel each other out. Such substances, called paramagnetic materials, do not produce a magnetic field on their own. However, if an external field is applied to them, the dipoles will align with the external field to produce a weak magnetic field in the substance. The dipoles will quickly return to a random arrangement when removed from the external field.
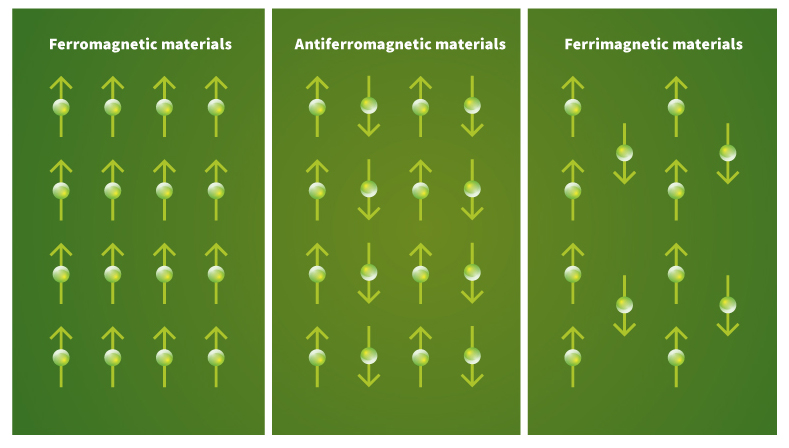
Certain materials known as ferromagnetic materials have groups of dipoles pointing in the same direction. Such groups of dipoles are called magnetic domains. However, the many domains in a substance usually point in many different directions, canceling one another out. Like the dipoles in paramagnetic materials, domains in ferromagnetic substances will align with an external field. Ferromagnetic materials can produce a strong magnetic field. This field may even be many times stronger than the external field. Unlike paramagnetic materials, ferromagnets retain their magnetism for a time after the external field is removed.
Ferromagnetic materials are split into two general types: hard and soft. Hard ferromagnetic materials can remain magnetized for long periods after being removed from the external field. Permanent magnets are often made of hard ferromagnetic substances. Soft ferromagnetic materials lose their magnetism quickly when removed from an external field and are often used for temporary magnets.
In other materials, each magnetic domain consists of an alternating pattern of dipoles pointing in opposite directions. This pattern, found in antiferromagnetic materials, causes the magnetic fields of the dipoles within a domain to cancel one another out. Like ferromagnetic material, the domains will also point in random directions in relation to one another. These materials thus have no magnetic field. Furthermore, neither the domains nor the individual dipoles will readily realign in an external field and therefore the material does not give rise to an overall magnetic field.
In some materials, the domains are composed of alternating dipoles that point in opposite directions, similar to those in antiferromagnets. However, in these materials, called ferrimagnetic materials, one kind of dipole is stronger than the ones pointing in the opposite direction. Because the dipoles have different strengths, they do not fully cancel one another out within each domain. Even though the domains in ferrimagnetic materials produce a magnetic field, the domains are arranged randomly within the material and tend to cancel one another out. Unlike antiferromagnetic materials, ferrimagnetic substances can produce strong magnetic fields when exposed to an external field.
All materials exhibit a weak form of magnetism called diamagnetism. The property is a result of an external field altering the way electrons orbit the nucleus. A diamagnetic field weakly repels the external field. The repulsion is similar to the repulsion between two like poles. But the repulsion occurs no matter which pole of a magnet is brought near the material. Diamagnetic fields are extremely weak and are easily overpowered by other forms of magnetism.
In all magnetic materials with an orderly alignment of dipoles, high temperatures can destroy the ordering. Above a certain temperature, ferromagnetic, antiferromagnetic, and ferrimagnetic materials become paramagnetic. They have completely random atomic dipole alignment and no overall magnetic field. The temperature at which ferromagnetic and ferrimagnetic materials become paramagnetic is called the Curie temperature. The temperature at which antiferromagnetic materials change over is called the Néel temperature. To be useful in the most applications, permanent magnets must have a Curie temperature higher than room temperature. Iron, the most common ferromagnetic element, has a Curie temperature of 1418 °F (770 °C).
Electromagnetism.
Magnetism and electricity together make up the force called electromagnetism, one of the basic forces in the universe. Just as current in a loop can produce a magnetic field, a changing magnetic field over a loop of wire can produce a current. Changing magnetic fields produce changing electric fields, and vice versa. When a magnetic field oscillates (moves back and forth), it creates an electric field oscillating in a perpendicular direction. Together, the two fields form an electromagnetic wave. These waves are also called electromagnetic radiation or electromagnetic energy.
The combined magnetic and electric field can oscillate at different frequencies (rates), but the radiation always travels at the speed of light. Certain frequencies of electromagnetic radiation are visible to the human eye and are often called visible light or simply light. Other frequencies are invisible to the eye but can be detected by other means. Radio waves and microwaves oscillate at a slower rate than does visible light. Ultraviolet light and X rays oscillate faster. The faster the oscillation, the more energy the wave carries.
The magnetism of astronomical bodies
Many large bodies in the solar system act as giant magnets. These bodies include the planets and the sun.
Earth
is a huge magnet with poles called the north magnetic pole and the south magnetic pole. These poles are near, but are different from, the geographic North and South poles.
The magnetic field at the surface of Earth, known as the geomagnetic field, has a strength of about 1/2 gauss. Earth’s inner structure creates the geomagnetic field. Part of the core (center) of Earth is composed of iron that is still hot enough to remain liquid. The rotation of Earth spins the liquid iron and creates an electric current. The current produces Earth’s geomagnetic field.
Lava contains small magnetic particles that align with Earth’s magnetic field as they cool. Thus, rocks that form from lava record the alignment of Earth’s field at the time when the lava cooled. By studying this evidence, scientists have found that the geomagnetic field does not stay the same. For example, the magnetic poles “drift” and even reverse at times—that is, Earth’s north and south magnetic poles switch places. Such reversals have happened at random intervals, from as little as 10,000 years to as long as 50 million years apart. Scientists do not yet understand why these reversals occur.
Earth’s magnetic field also extends into space beyond the atmosphere. There, it is called the magnetosphere. The magnetosphere interacts with a flow of charged particles from the sun called the solar wind. This interaction creates displays of light called auroras, including the so-called “northern lights.” The interaction also produces two zones of charged particles around Earth known as the Van Allen belts.
The sun
has an overall magnetic field of about 1 to 2 gauss. But it also has stronger magnetic fields concentrated in cooler areas of its surface called sunspots. These regions have magnetic fields of 100 to 5,000 gauss. Other solar features associated with strong magnetic fields include bright bursts of light called flares and huge arches of gas known as prominences.
Other astronomical bodies.
The moon has virtually no magnetic field because it does not have a liquid core. But moon rocks brought to Earth by astronauts show that the moon at one time may have had a stronger magnetic field. This evidence suggests that the moon once had a liquid core. Mercury, Venus, and Mars all have fields weaker than Earth’s. But the outer planets Saturn, Jupiter, Neptune, and Uranus have strong magnetic fields and magnetospheres.
Some types of stars have magnetic fields much stronger than the sun’s. These stars include burned out stars called white dwarfs, which can have magnetic fields of more than 100 million gauss. A type of collapsed star called a neutron star typically has a field as strong as 1 to 10 trillion gauss. One specific type of neutron star, called a magnetar, may have a field up to 100 times as powerful as that of a typical neutron star.
The study of magnetism
The discovery of magnetite or a similar magnetic substance probably prompted the study of magnetism. However, it was not until the 1800’s that scientists gained a fuller understanding of magnetism and electricity.
Early discoveries.
People in ancient Greece and China independently discovered that natural lodestone magnets attracted iron. The Chinese also found that a piece of lodestone would point in a north-south direction if it was allowed to rotate freely. They used this characteristic of lodestone to tell fortunes and as a guide for building. By A.D. 1200, Chinese and European sailors used magnetic compasses to steer their ships.
In 1269, a French soldier named Pierre de Maricourt, known in English as Peter Peregrinus, mapped the magnetic field around a lodestone sphere with a compass. He discovered that the sphere had two magnetic poles. William Gilbert, a physician of Queen Elizabeth I of England, concluded in 1600 that Earth is a giant magnet with north and south poles.
Electricity and magnetism.
In 1820, Hans Christian Oersted, a Danish physicist, observed that an electric current flowing in a wire caused the needle of a magnetic compass to rotate. His discovery proved that electricity and magnetism were related. The French physicist André-Marie Ampère worked out the mathematical relationship between current and the strength of the magnetic field during the 1820’s. He also proposed that electric current in atoms caused magnetism. In the early 1830’s, the English scientist Michael Faraday and the American physicist Joseph Henry independently discovered that a changing magnetic field created a current in a coil of wire. In 1864, James Clerk Maxwell, a Scottish scientist, developed the mathematical theory—now called Maxwell’s equations—that describes the laws of electricity and magnetism. These laws are among the most fundamental of the laws of physics.
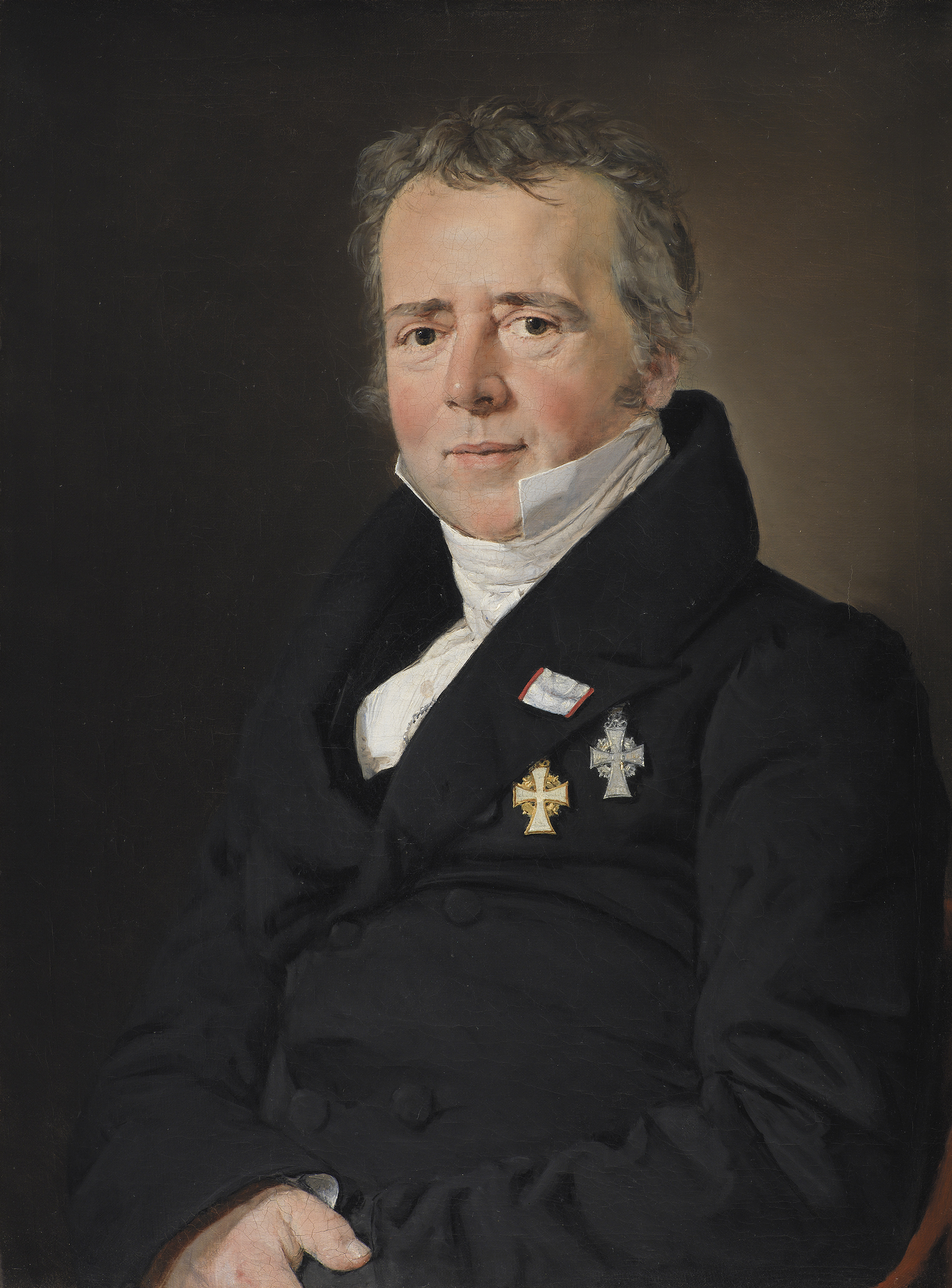
Magnetic properties of materials.
In the late 1800’s, the French physicist Pierre Curie found that ferromagnetic materials lose their ferromagnetism above a certain temperature. This temperature became known as the Curie temperature.
It was not until the early 1900’s that scientists began to truly understand magnetism in materials. They gained this understanding through the development of quantum mechanics, a branch of physics that describes the interaction of electrons with each other and with electromagnetic fields. Scientists discovered the first antiferromagnets, now among the most common of magnetic materials, during the 1930’s.
Magnetic resonance.
In the 1940’s, two physicists, the American Edward M. Purcell and the Swiss-born American Felix Bloch, independently developed a way to measure and manipulate the magnetic field of nuclei. This discovery became known as nuclear magnetic resonance (NMR). In the 1970’s, NMR was further developed into MRI technologies largely by the American physicist Paul C. Lauterbur and the British physicist Peter Mansfield. The two men shared the 2003 Nobel Prize in Physiology and Medicine for their work.
Different nuclei respond differently to external magnetic fields. MRI can detect the difference between healthy cells and cancerous ones. MRI can also find differences in the composition of tissues. Physicians use MRI to image and study the entire human body as well as many nonliving materials.
Magnets in living things.
Scientists have discovered that many animals—including bats, honey bees, mole-rats, pigeons, salmon, tuna, and sea turtles—can detect Earth’s magnetic field. Even some bacteria have that ability. Animals may use Earth’s magnetic field to help find their way as they travel from place to place. Scientists have found particles of magnetite in the body tissues of some of these animals. They suspect that the particles form part of a system that senses the geomagnetic field. The particles may act much like a compass needle.
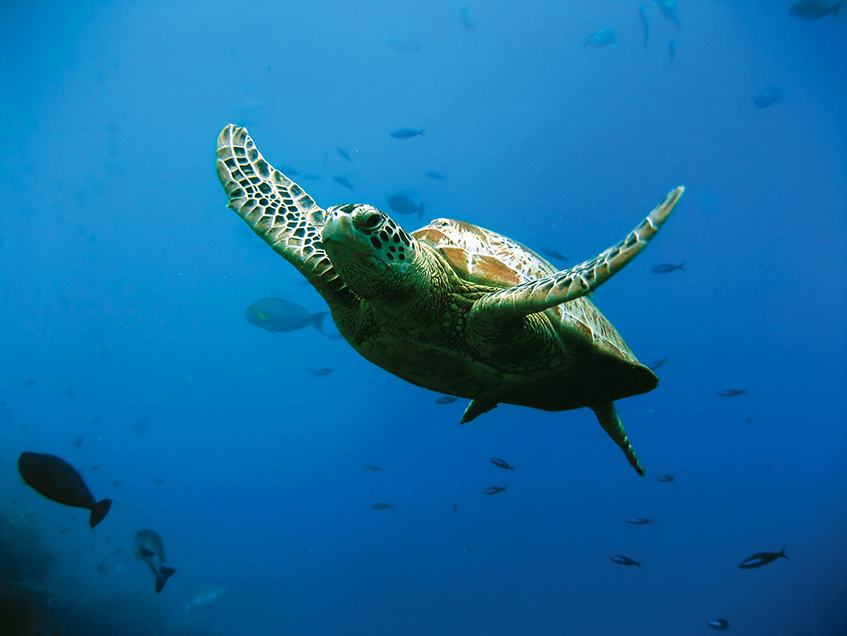
Superconductivity.
Electric power passing through a metal wire encounters resistance and loses a fraction of its energy as heat. Superconductivity is a phenomenon in which a substance carries current without energy loss. It occurs only at low temperatures and only in some materials. No known material can superconduct at room temperature. The “warmest” superconductor has a transition temperature—the temperature at which it begins to superconduct—colder than –200 °F (–129 °C). Electromagnets made of superconducting wire form some of the strongest magnets. They are used in high-speed magnetic levitation trains and particle accelerators.
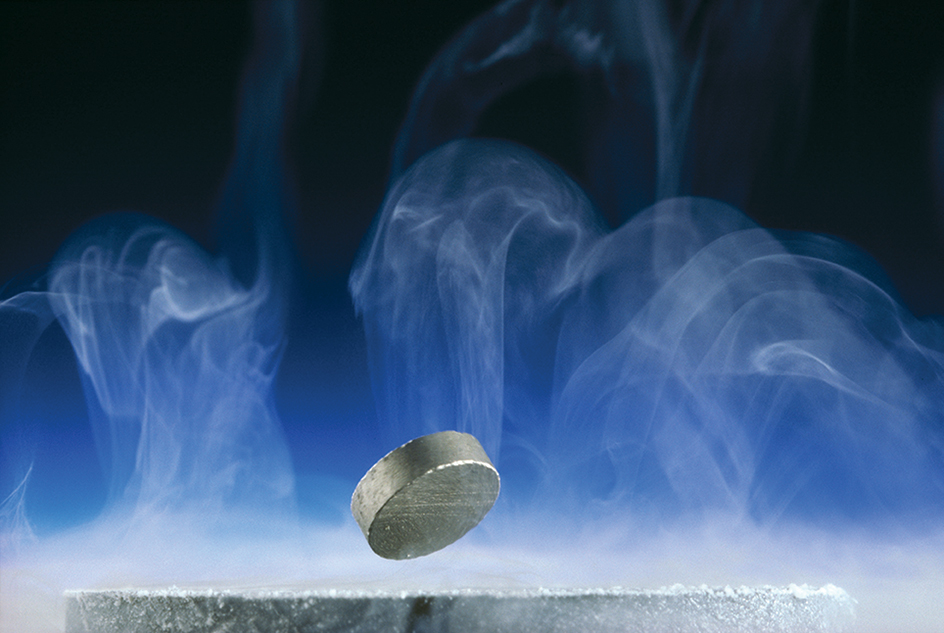
An interesting characteristic of superconductors is that they expel (push out) magnetic fields. If a magnet is placed on top of a superconducting material at room temperature, the magnetic field lines of the magnet penetrate the superconductor. When the superconductor is cooled below its transition temperature, it pushes the field lines away from itself. This pushing causes the magnet to levitate. Scientists have caused magnets large enough for a human to stand on to levitate above a superconducting disk.
Magnetic monopoles.
Although electricity and magnetism are related and have many of the same properties, magnets do not have monopoles (lone poles) as charges do. That is, electric charges can be either positive or negative. The existence of a positive charge does not require a negative one. However, magnets have north and south poles that cannot be separated. Cutting a magnet in half results in two magnets, each with its own north and south pole. Most scientists now accept the lack of true magnetic monopoles, but for many years researchers tried to find them.
In the 2000’s, scientists found monopole-like objects in a material called spin ice. Spin ice is composed of magnetic ions arranged like hydrogen ions in water ice. When cooled to extremely low temperatures, the spin-ice ions form small packets of charge. The packets, which behave like north and south magnetic poles, can be separated from one another, creating something like magnetic monopoles. However, these “monopoles” exist in equal numbers of both types and only within the spin ice. Because of the equal number of opposite charges spread evenly throughout the spin ice, the substance has no overall magnetic field.