Mars is the fourth planet from the sun and the next planet beyond Earth in our solar system. Of all the planets in our solar system, Mars has the surface environment that most closely resembles that of Earth. Mars has weather and seasons and landforms that appear familiar. Salty water may flow just below the planet’s surface. Like Earth, the sun, and the rest of the solar system, Mars is about 4.6 billion years old.
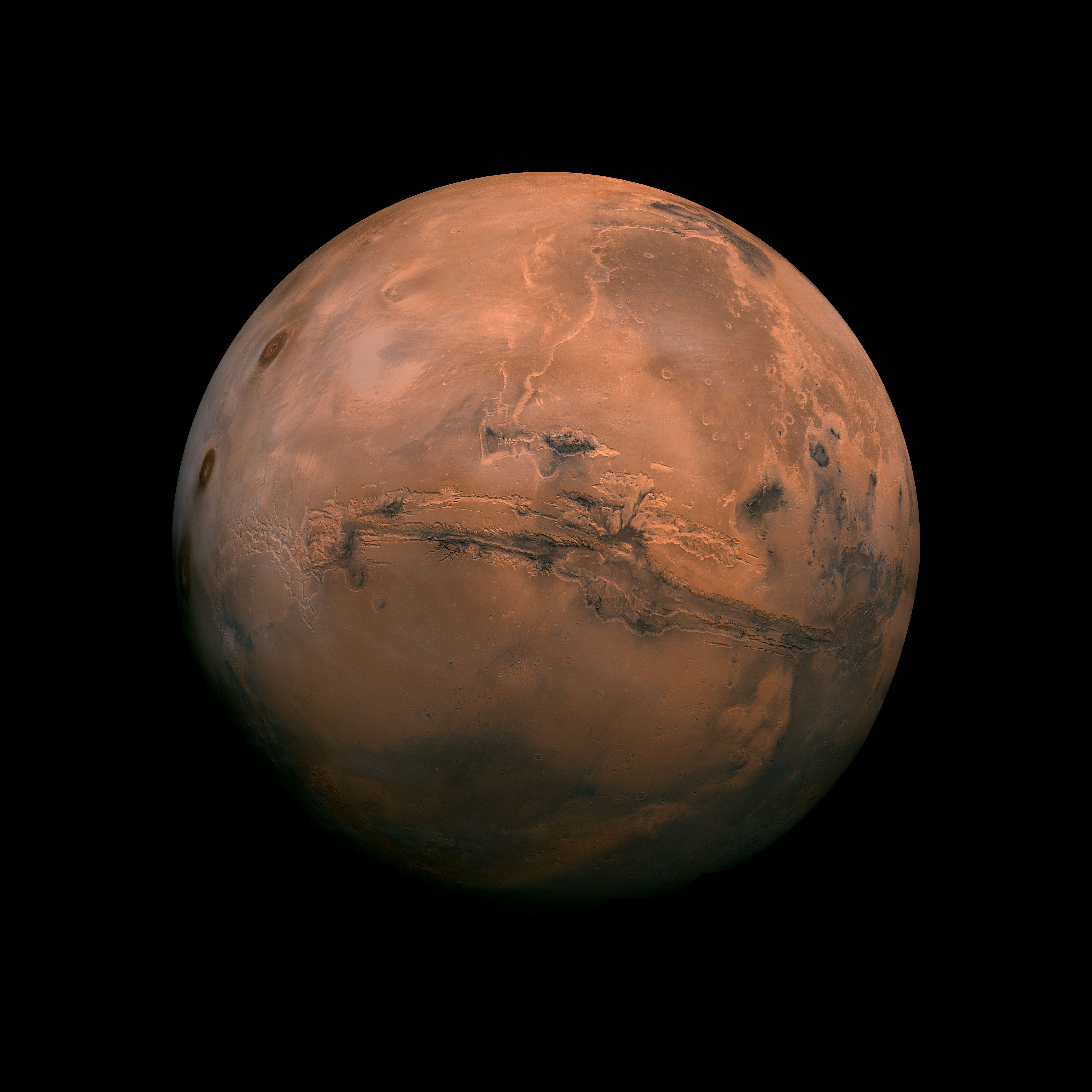
Mars has a reddish color that is visible from Earth with the unaided eye. The color comes from weathered iron-rich minerals present in the Martian dust and surface rocks. Many ancient peoples associated the planet with war and conflict because of its blood-red appearance. Indeed, Mars is the Latin name for the ancient Roman god of war (see Mars). Today, Mars is nicknamed “the Red Planet” in reference to its color.
Mars has a special place in popular culture, one that is unique among the planets. This fascination with Mars probably developed because the planet is relatively close to and similar to Earth. Early observations with Earth-based telescopes inspired popular speculation that Mars was home to all kinds of life, even alien civilizations. Many works of science fiction have played upon these ideas, showing humans visiting Mars or malevolent Martians invading Earth.
In the 1960’s, the U.S. Mariner spacecraft showed Mars to be a cold, desert planet devoid of visible life. But Mars continues to fascinate people with evidence of past surface water and the possibility that microscopic life once existed or still exists there. Experts think Mars will be the first planet other than Earth to be visited by human explorers.
Despite their similarities, Mars and Earth differ in key respects that make Mars less hospitable to life. Mars is roughly half again as far from the sun as is Earth. The extra distance from the sun helps make the surface of Mars much colder, about -80 °F (-60 °C) on average. Water can thus only exist on the Martian surface as ice. Mars is also about half the diameter of Earth and has a much lower mass (amount of matter). As a result, Mars has a much lower gravitational pull, which has allowed much of its atmosphere to escape into space over time. Today, the atmosphere of Mars is about 1/100 as dense as that of Earth. Even if temperatures were higher, therefore, most liquid water on Mars would boil away due to the greatly reduced air pressure.
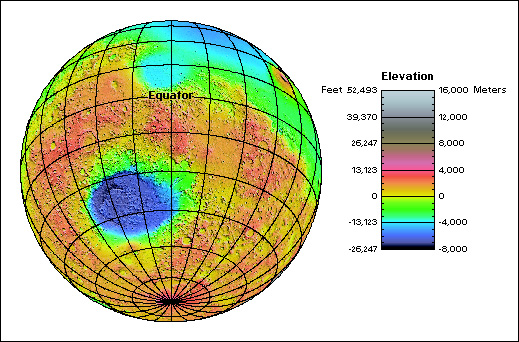
Scientists think that in the past, however, Mars was more like Earth. Shortly after the formation of the solar system, Mars would have been warmer and had a thicker atmosphere, so liquid water could have flowed on its surface. Such conditions seem more favorable to the development of life. If life did form on Mars, it may have gone extinct as the planet cooled. Or life could have migrated below the surface and may remain there today. No conclusive evidence has been found that Mars ever harbored life. Determining whether life did develop on Mars will help scientists better estimate how common—or how rare—life is in the universe.
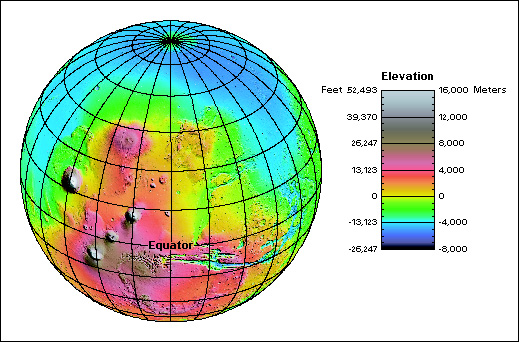
Characteristics of Mars
Orbit and rotation.
Like the other planets in the solar system, Mars travels around the sun in an elliptical (oval-shaped) orbit. The orbits of the other planets in our solar system are nearly circular, but the orbit of Mars is slightly more elongated (stretched out). The average distance from Mars to the sun is about 141,620,000 miles (227,920,000 kilometers), but the planet can be as close to the sun as about 128,390,000 miles (206,620,000 kilometers) or as far away as about 154,860,000 miles (249,230,000 kilometers). Mars travels around the sun once every 687 Earth days, the length of the Martian year.
The distance between Earth and Mars depends on the positions of the two planets in their orbits. It can be as small as about 34,600,000 miles (55,700,000 kilometers) or as large as about 249,000,000 miles (401,300,000 kilometers).
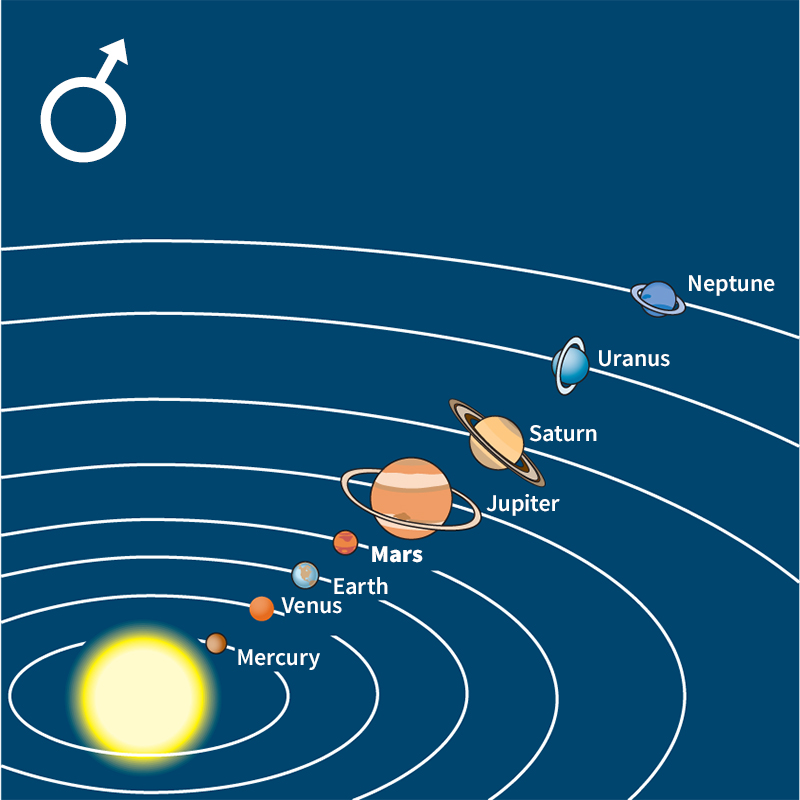
Like Earth, Mars rotates on its axis from west to east. A solar day is the length of time a planet takes to turn around once with respect to the sun. The Martian solar day, sometimes called a sol, is 24 hours 39 minutes 35 seconds long, slightly longer than Earth’s solar day of 24 hours.
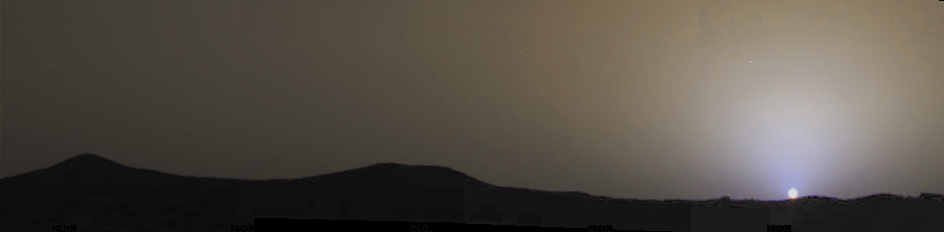
The axis of Mars is not exactly perpendicular to the planet’s orbital plane, an imaginary plane that includes all points in its orbit around the sun. Rather, the axis is tilted from the perpendicular position. The angle of the tilt, called the planet’s obliquity, is 25.19°, similar to Earth’s obliquity of 23.45°. The obliquity of Mars, like that of Earth, causes the amount of sunlight falling on certain parts of the planet to vary widely during the year. As a result, Mars, like Earth, has seasons.
Size, mass, and density.
The average diameter of Mars is 4,212 miles (6,779 kilometers). But like Earth, Mars is not perfectly round. It bulges slightly at its equator due to its rotation, making a shape called an oblate spheroid. The diameter of Mars at its equator is 4,220 miles (6,792 kilometers), whereas the diameter through the poles is about 4,196 miles (6,752 kilometers).
Mars has a mass about 1/10 that of Earth. Mars’s average density (mass divided by volume) is about 3.934 grams per cubic centimeter. This figure is roughly 70 percent of the average density of Earth.
Gravitational force.
Because Mars is so much smaller and less dense than Earth, the force due to gravity at the Martian surface is only about 38 percent that on Earth. Thus, a person standing on Mars would feel as if their weight had decreased by 62 percent, or nearly two-thirds. If that person dropped a rock, the rock would fall to the surface more slowly than the same rock would on Earth.
Physical features of Mars
Interior.
Scientists once relied entirely on indirect knowledge to inform their ideas about the interior of Mars, such as calculations involving the planet’s mass, density, and gravity. Today, scientists can use data from landers to directly study the interior. With an instrument called a seismometer, a lander can record tremors (earthquakes). Tremors on Mars are sometimes called marsquakes. Scientists analyze patterns in the tremors to deduce the interior structure of the planet. Mars has three main layers, as does Earth: (1) a rocky crust, (2) a mantle of denser rock beneath the crust, and (3) a core made mostly of iron.
Crust.
Seismic measurements show the crust of Mars has multiple layers, some of which seem to be nearly global in extent. Analyses of marsquake and gravity data indicate that the thickness of the crust varies widely, but the average thickness is about 25 miles (40 kilometers).
Much of the crust probably consists of a volcanic rock called basalt << buh SAWLT >>. Basalt is also common in the crusts of Earth and the moon (see Basalt). Some Martian crustal rocks, particularly in the northern hemisphere, may be a form of andesite. Andesite is also a volcanic rock found on Earth, but it contains more silica than basalt does. Silica is a compound of silicon and oxygen (see Silica).
Mantle.
The mantle of Mars is probably similar in composition to upper layers of Earth’s mantle. Most of Earth’s upper mantle rock is peridotite << puh RIH duh tyt >>, which is made up chiefly of silicon, oxygen, iron, and magnesium. The most abundant mineral in peridotite is olivine << OL uh veen >>.
The main sources of heat inside Mars must be the same as those inside Earth. The first heat source is heat left over from the early formation of the planet. The second source is radioactive decay, the breakdown of the nuclei (cores) of atoms of elements such as uranium, potassium, and thorium. The average temperature of the Martian mantle today may be roughly 2700 °F (1500 °C).
Core.
Because the average density of Mars is much greater than that of any rock, scientists have known since the mid-1900’s that the planet must have a core similar to Earth’s core, made up primarily of iron and nickel with some lighter elements such as sulfur. Using measurements of seismic waves (from marsquakes) that bounce off or travel through the core, we know that the core measures between 2,250 and 2,311 miles (3,620 and 3,720 kilometers) in diameter and that up to 18 percent of the core is sulfur. These seismic measurements also confirm that the core is still molten. It is still molten at the relatively cool temperatures within Mars because the presence of such elements as sulfur lowers the temperatures at which the core can remain liquid.
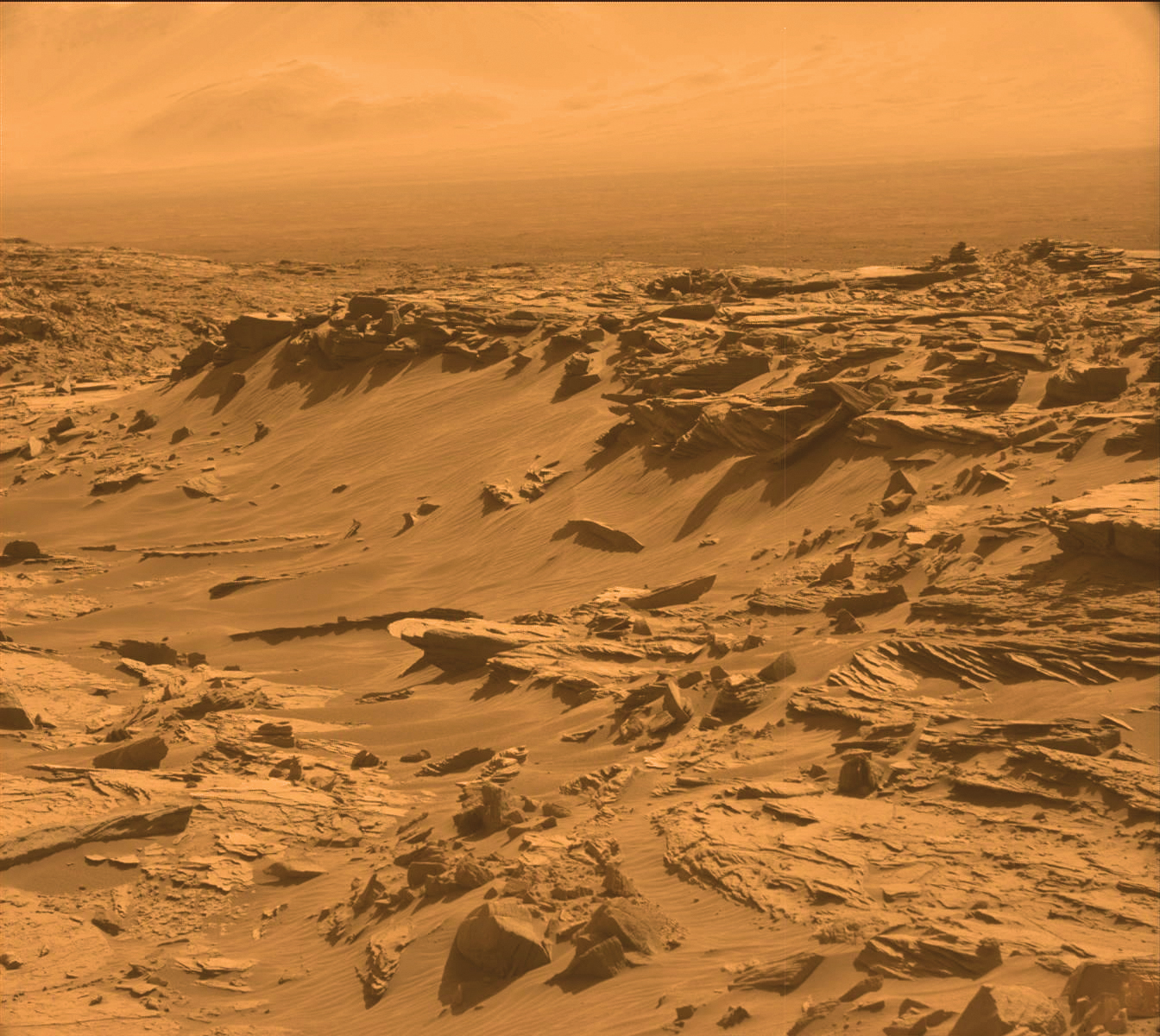
Evidence suggests that the planet’s core produced a strong magnetic field in the distant past. A magnetic field is a region of magnetic influence that surrounds a magnetic object, in this case Mars. Large-scale magnetic fields in planets are thought to result from particular flow patterns of molten metal in a planet’s core. This type of flow is caused by the cooling of a molten core in a spinning planet. Data from a probe called the Mars Global Surveyor show that some of the oldest Martian rocks formed in the presence of a strong magnetic field. However, measurements from the same probe show that Mars no longer has a strong magnetic field. Although the core remains molten, it is apparently now cooling too slowly to generate a magnetic field.
Surface features.
Mars has many of the kinds of surface features that are common on Earth. These include plains, canyons, volcanoes, valleys, gullies, and polar ice. But craters also cover the surface of Mars, whereas they are rare on Earth. In addition, fine-grained reddish dust covers almost all the Martian surface.
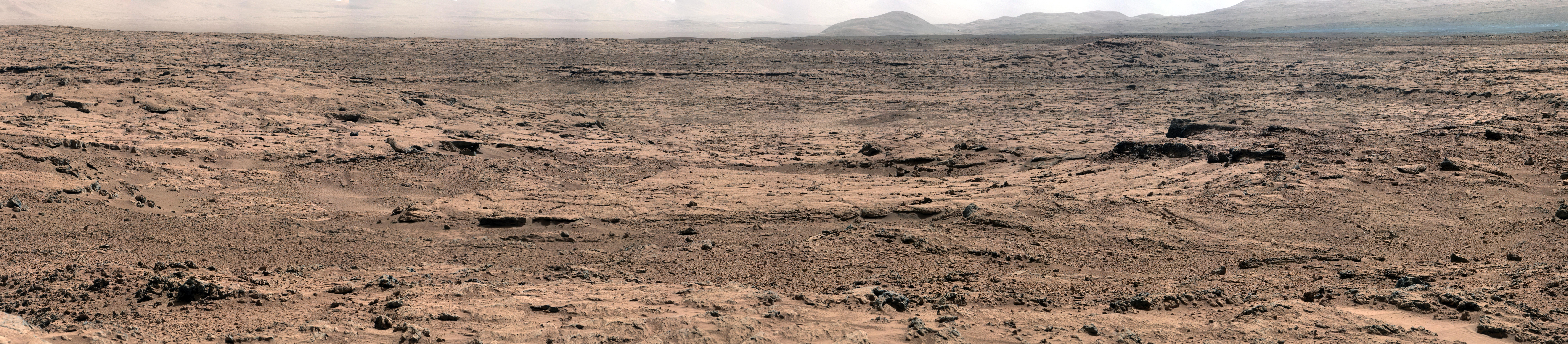
Large-scale features.
The geography of Mars is dominated by two continent-scale features, the Northern Lowlands and the Tharsis Plateau. The Northern Lowlands are relatively smooth plains that cover more than a third of the planet. They have an average elevation about 3 miles (5 kilometers) lower than that of the rougher, heavily cratered south. It is thought that a huge impact formed the Northern Lowlands early in Mars’s history.
The Tharsis Plateau is a roughly circular volcanic region about 5,000 miles (8,000 kilometers) across and up to nearly 6 miles (10 kilometers) high. It covers almost a quarter of the planet.
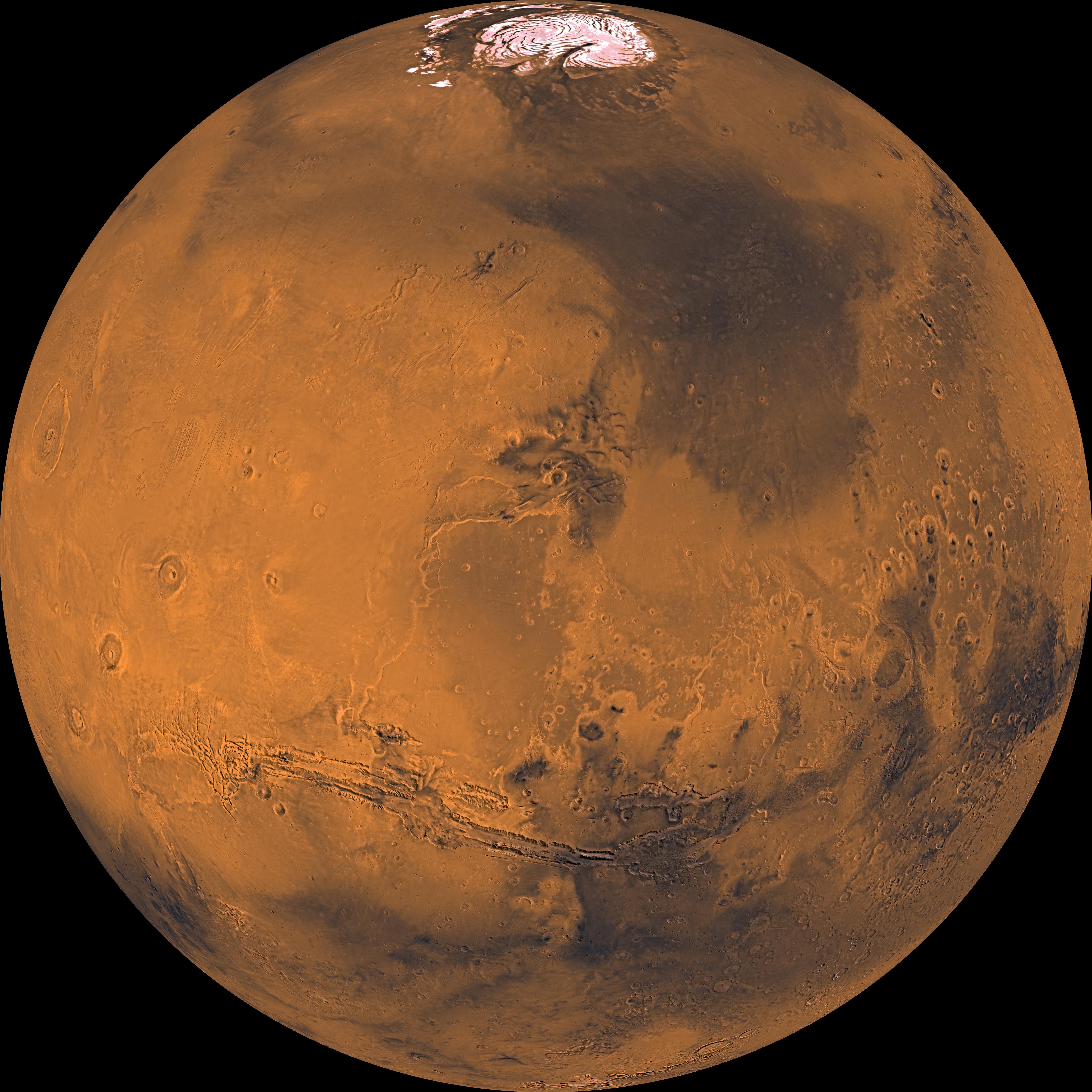
Polar features.
Both poles of Mars feature permanent caps of water ice that are more than 1 mile (1.6 kilometers) thick. In addition, the southern cap has a roughly 25-foot (8-meter) thick permanent covering of frozen carbon dioxide (CO2). The northern cap has a diameter of about 625 miles (1,000 kilometers), and the southern cap has a diameter of about 220 miles (350 kilometers). In the wintertime, additional seasonal caps form from layers of CO2 frost that condense from the atmosphere. The growth and shrinkage of these seasonal caps is clearly visible through Earth-based telescopes. During the coldest part of winter in each hemisphere, the frost extends from the pole nearly halfway to the equator.
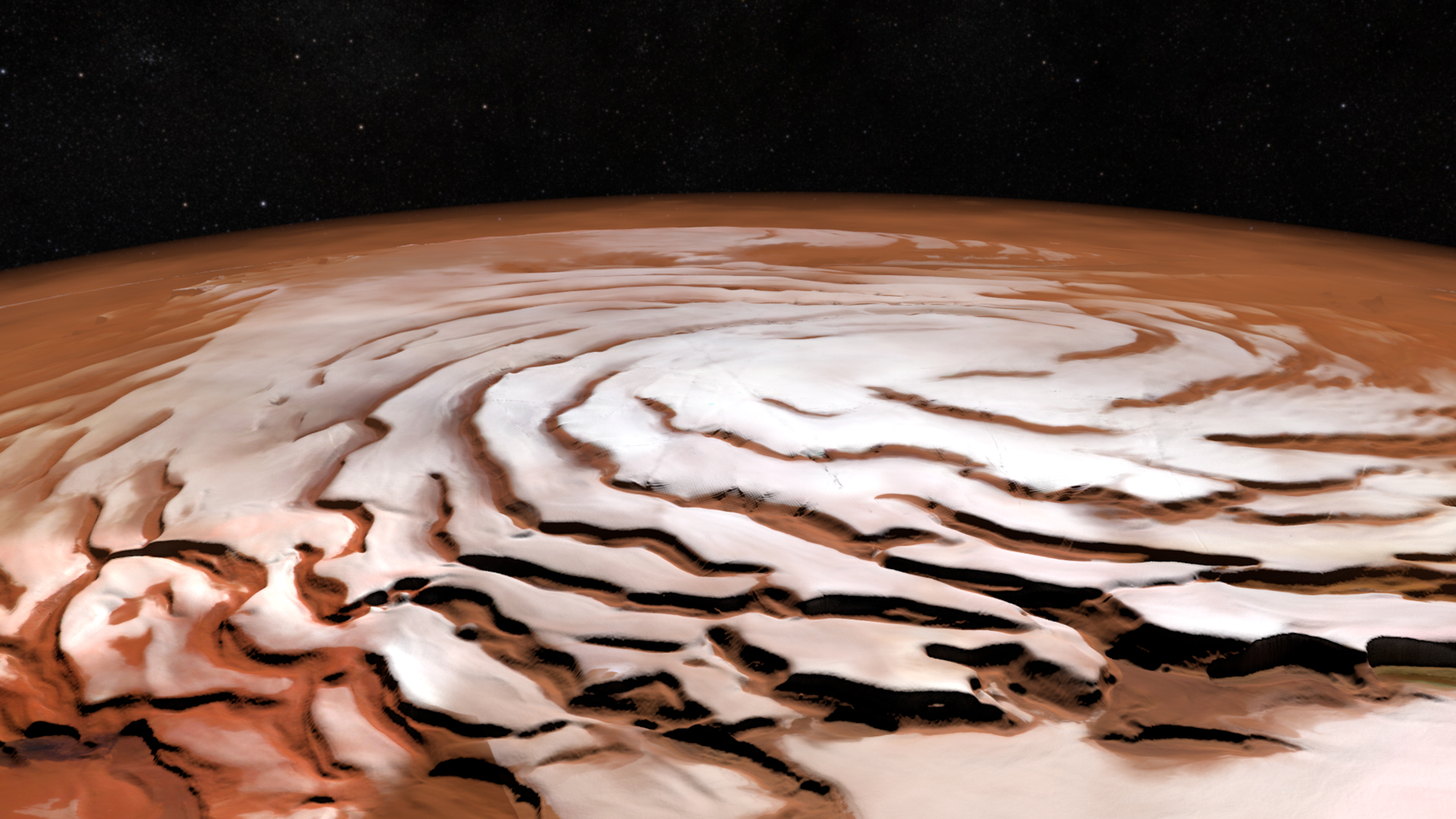
Another unique feature of the polar regions is polar layered deposits. These deposits are thick stacks of finely layered material. Scientists think that the layers consist of mixtures of water ice and dust. The deposits occur within about 400 miles (650 kilometers) of the pole in both hemispheres.
The atmosphere probably deposited the layers over long periods. Past climate change could have affected the rate at which the atmosphere deposited dust and ice into layers. Therefore, the layers may provide evidence of seasonal weather activity and long-term changes in the Martian climate.
Craters,
formed by the impacts of meteorites and asteroids over time, are the most common geological feature on Mars. Martian craters are similar to craters on Earth’s moon, the planet Mercury, and other objects in the solar system. The craters have deep, bowl-shaped floors and raised rims. Large craters can also have central peaks that form when the crater floor rebounds after an impact.
On Mars, craters range in size from a few feet (about 1 meter) to thousands of miles or kilometers in diameter. The largest craters, with diameters greater than about 200 miles (300 kilometers), are often referred to as impact basins. Utopia Planitia (planitia is a Latin word that can mean low plain or basin) is the largest confirmed impact basin in the solar system, with a diameter of 2,100 miles (3,300 kilometers). Despite its large size, it is only about 1 mile (1 ½ kilometers) deep. Hellas Planitia is somewhat smaller (1,400 miles, or 2,300 kilometers in diameter) but much deeper, with a floor nearly 6 miles (10 kilometers) below the surrounding plains.
Some of the impact craters have unusual-looking deposits of ejecta, material thrown out of the craters at impact. These deposits resemble mudflows that have solidified. This appearance suggests that the impacting bodies may have encountered water or ice beneath the ground.
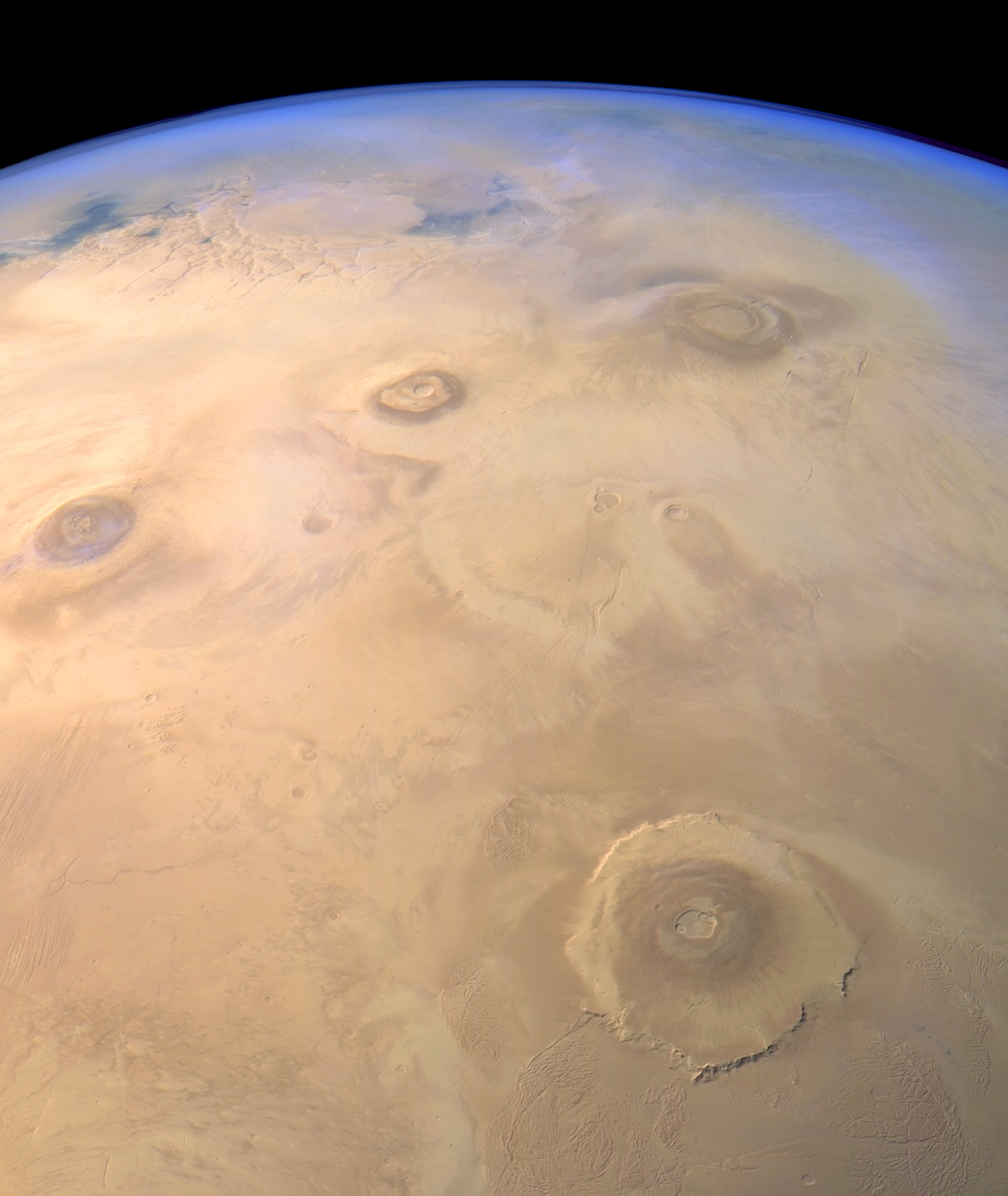
Volcanoes.
Mars has the largest volcanoes in the solar system. The tallest one, Olympus Mons (Latin for Mount Olympus), rises about 16 miles (25 kilometers) above the surrounding plains. It is more than 370 miles (600 kilometers) in diameter. Three other large volcanoes, called Arsia Mons, Ascraeus Mons, and Pavonis Mons, sit atop the Tharsis Plateau.
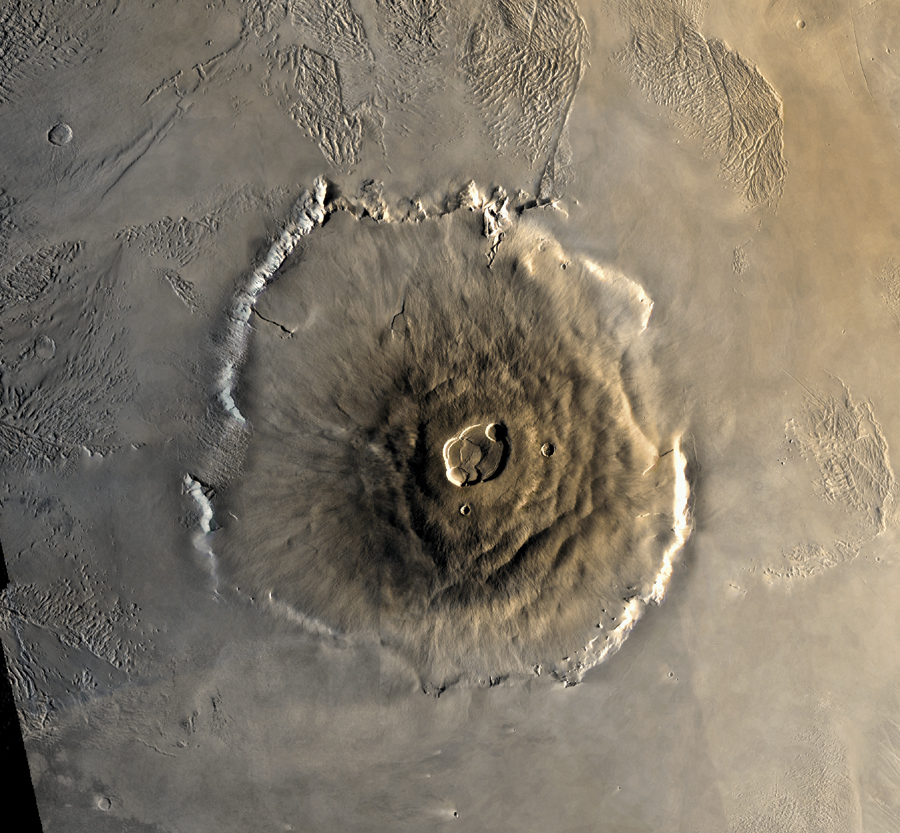
All these volcanoes have slopes that rise gradually, much like the slopes of Hawaiian volcanoes. Both the Martian and Hawaiian volcanoes are shield volcanoes. They formed from eruptions of basalt lavas that can flow for long distances before solidifying.
Mars also has many other types of volcanic landforms. These range from small, steep-sided cones to enormous plains covered with hardened lava. Although most of the volcanic landforms are extremely old, some minor eruptions may still occur.
Canyons.
Along the equator of Mars lies one of the most striking landforms in the solar system—a system of canyons known as the Valles Marineris. The name is Latin for Mariner Valleys. A space probe called Mariner 9 discovered the canyons in 1971. The canyons run roughly east and west for about 2,500 miles (4,000 kilometers), which is close to the width of Australia or the distance from Philadelphia to San Diego. The depth of the canyons is enormous, reaching 5 to 6 miles (8 to 10 kilometers) in some places. Scientists believe that the Valles Marineris formed mostly through rifting, a splitting of the crust due to being stretched.
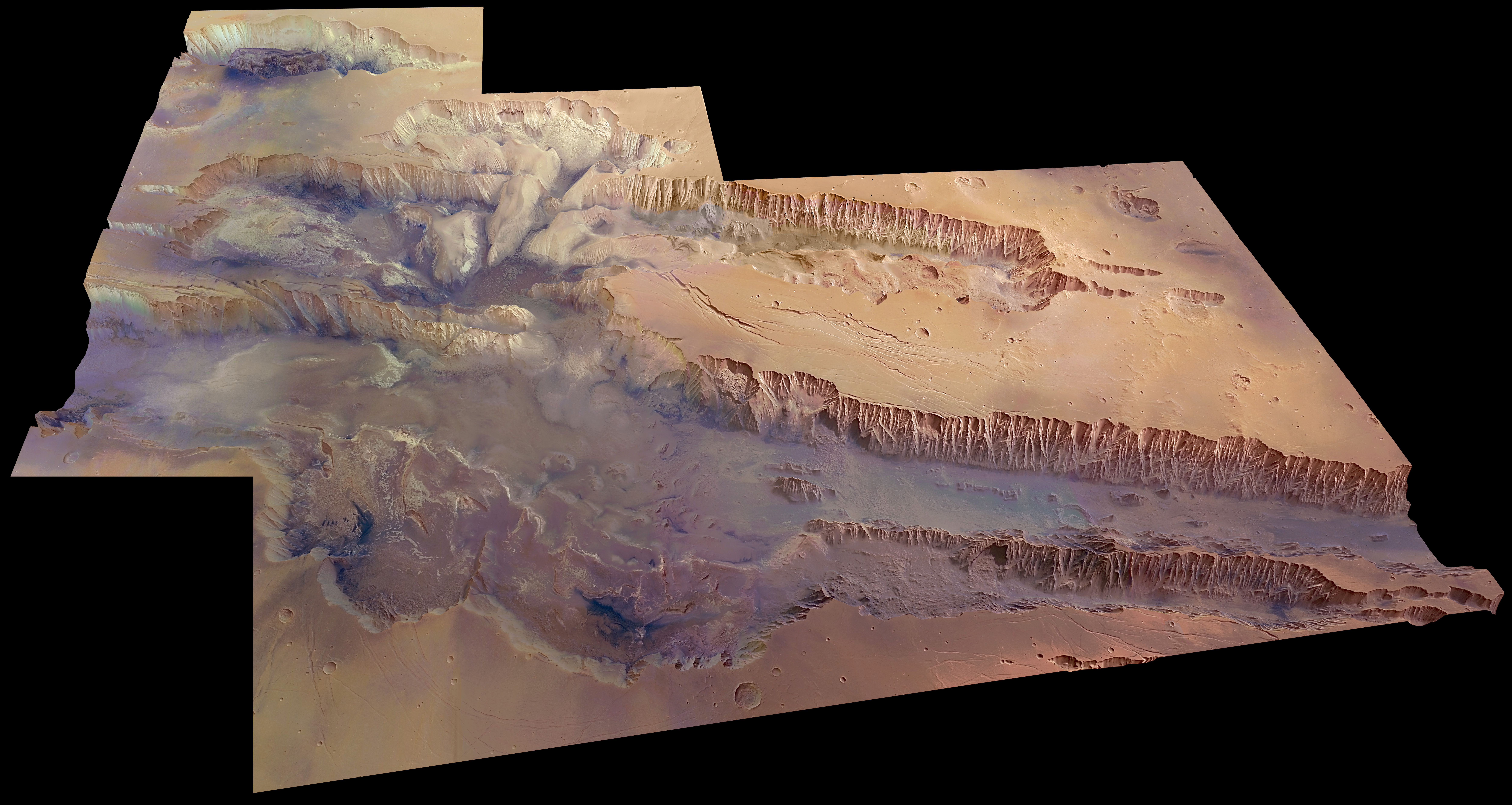
Large channels emerge from the eastern end of the canyons, and some parts of the canyons have layered sediments. The channels and sediments indicate that the canyons may once have been partly filled with water.
Channels, valleys, gullies, and lineae.
A variety of features occur on Mars that are thought to be the result of erosion by water. The most striking of these features are known as outflow channels. These channels can be as wide as 60 miles (100 kilometers) and as long as 1,200 miles (2,000 kilometers). They appear to have been carved by enormous floods that rushed across the surface. Many of the channels do not look like river systems on Earth, in which the main river forms from smaller rivers and streams. Rather, the Martian channels arise fully formed from low-lying areas. In many cases, the water appears to have escaped suddenly from underground.
Other regions of Mars have much smaller features called valley networks. These networks look more like river systems on Earth. Martian valley networks are up to a few miles or kilometers wide and up to a few hundred miles or kilometers long, with individual valleys measuring a few tens to hundreds of feet or meters across. The networks appear to be very ancient. They suggest that the Martian climate may once have been warm enough to enable liquid water to exist on the planet’s surface.
Features called recurring slope lineae, or RSL, form on some hillsides and mountain slopes. Lineae is a Latin term that astronomers use to refer to linear features. Scientists discovered these features using orbital photographs taken of the same areas at different times of year. The lineae tend to form on warm slopes in warm seasons and to follow an annual cycle of gradual lengthening and then fading away as the weather turns cooler. They are about 1 ½ to 15 feet (0.5 to 5 meters) wide and can be hundreds of feet or meters long. Some locations display more than 1,000 individual features at a time. Most RSL are found in the southern hemisphere between 48° and 32° of latitude, although some occur in equatorial regions, most commonly in the Valles Marineris.
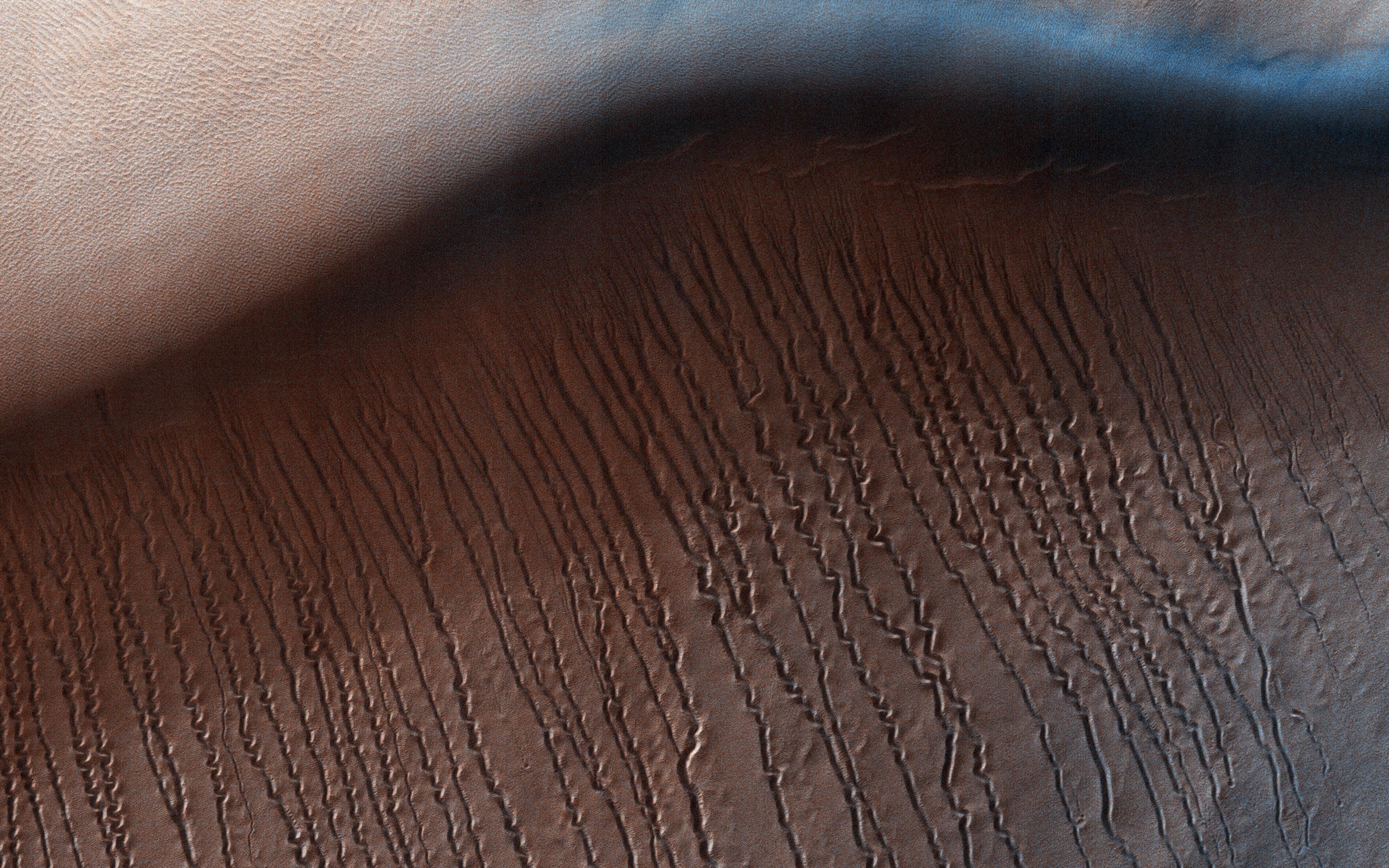
These features could form from dry flows of dust or sand down steep slopes. Or they may be created by salty water seeping to the surface near the top of a slope, when the water becomes warm, and quickly evaporating while flowing down the slope.
Atmosphere.
Carbon dioxide accounts for 95.3 percent of Mars’s atmosphere. The Martian atmosphere contains much less oxygen (O2) than that of Earth. Its O2 content is only 0.13 percent, compared with 21 percent in Earth’s atmosphere. Other gases include nitrogen (N2), 2.7 percent; argon (Ar), 1.6 percent; carbon monoxide (CO), 0.08 percent; and water vapor (H2O), 0.03 percent.
Pressure.
At the surface of Mars, the atmospheric pressure (also called barometric pressure) is typically only about 0.10 pound per square inch (0.7 kilopascal). This is roughly 0.7 percent of the atmospheric pressure at Earth’s surface.
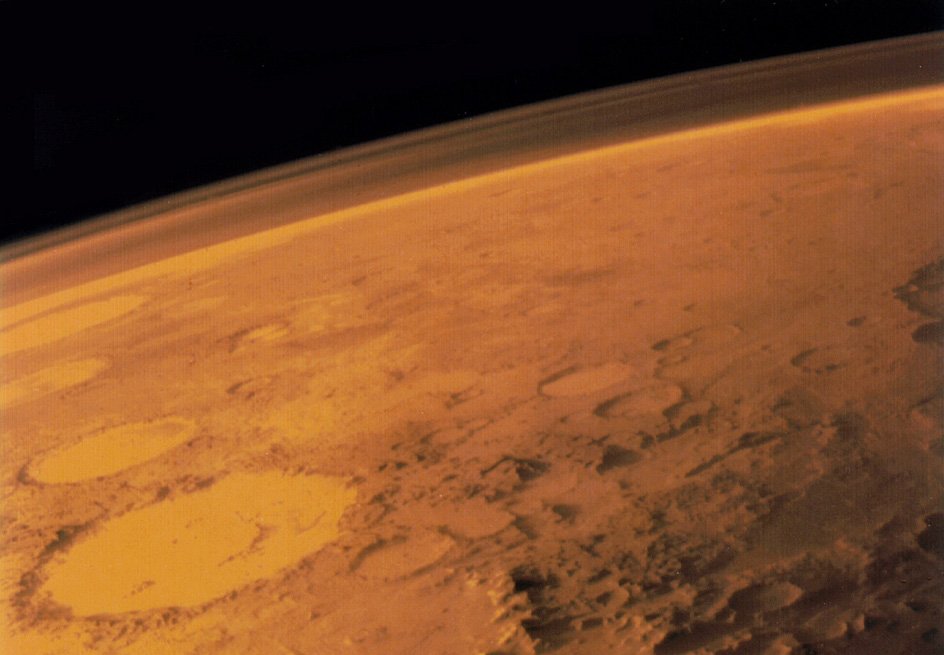
In both the northern and southern hemispheres, the condensation of CO2 at the poles during the winter removes much gas from the atmosphere. When this happens, the atmospheric pressure decreases sharply. The opposite process occurs each summer. Because the northern winter occurs when Mars is farthest from the sun, the northern polar cap has a larger effect on atmospheric pressure than does the southern cap. This variation causes seasonal fluctuations in atmospheric pressure of 20 to 30 percent. In addition, the atmospheric pressure varies as the weather changes during the day, much as on Earth.
Temperature.
The atmosphere of Mars is coldest at high altitudes, from about 40 to 78 miles (65 to 125 kilometers) above the surface. At those altitudes, typical temperatures are below –200 °F (–130 °C). The temperature increases toward the surface, where daytime temperatures of –20 to –40 °F (–30 to –40 °C) are typical. In the lowest few miles or kilometers of the atmosphere, the temperature varies widely during the day. It can drop to –150 °F (–100 °C) late at night, even near the equator. Atmospheric temperatures can be warmer than normal when there are high dust levels in the Martian air. The dust absorbs sunlight and then transfers much of the resulting heat to the atmospheric gases.
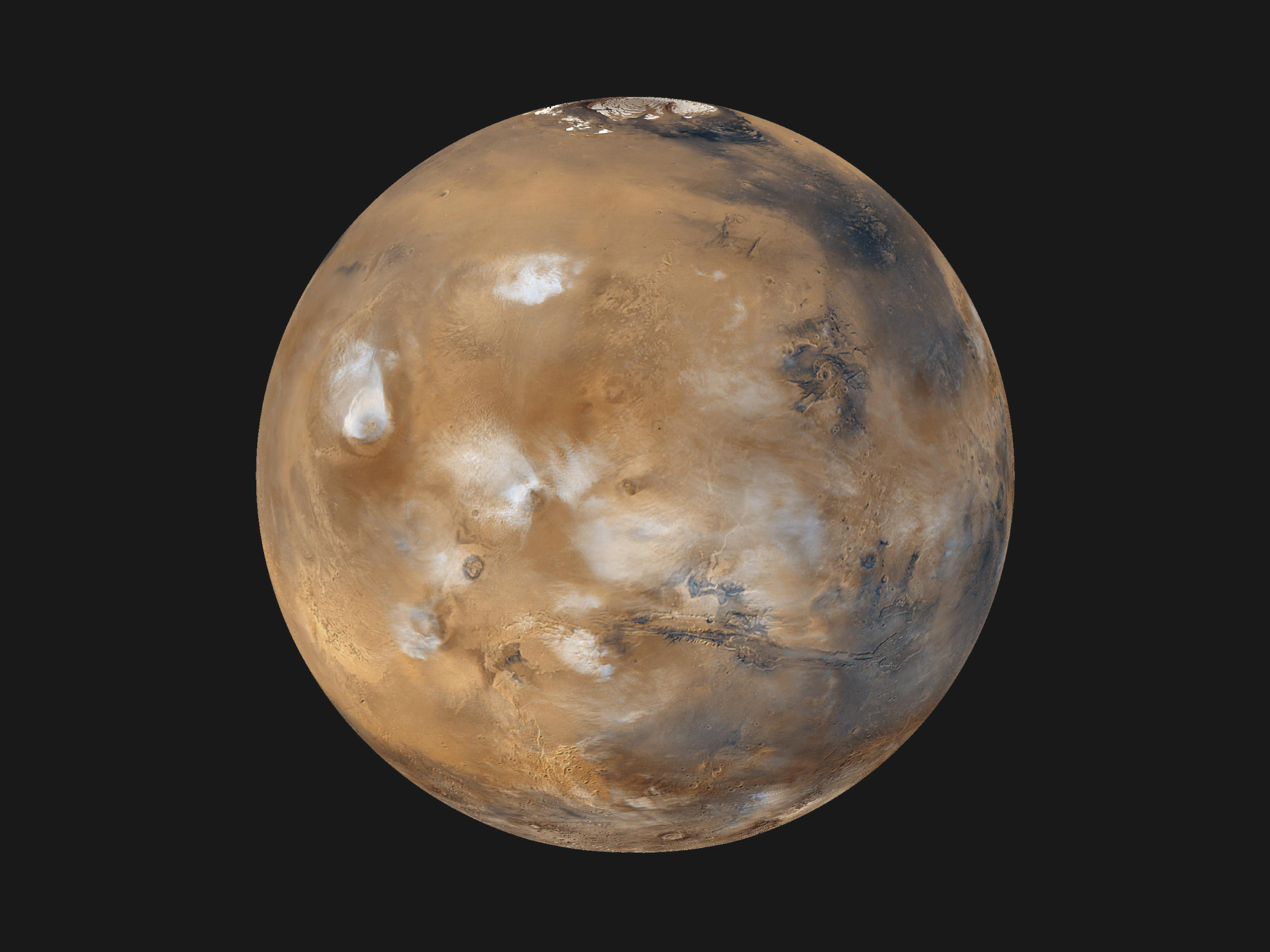
Clouds.
In the Martian atmosphere, thin clouds made up of particles of frozen CO2 can form at high altitudes. In addition, the atmosphere can host clouds, haze, and fog composed of particles of water ice. Haze and fog are especially common in the early morning. At that time, temperatures are the lowest, and water vapor is therefore most likely to condense.
Wind.
The Martian atmosphere, like that of Earth, has a general circulation, a wind pattern that occurs over the entire planet. Scientists have studied the global wind patterns of Mars by observing the motions of clouds and changes in the appearance of wind-blown dust and sand on the surface.
Global-scale winds occur on Mars as a result of the same process that produces such winds on Earth. The sun heats the atmosphere more at low latitudes than at high latitudes. At low latitudes, the warm air rises, and cooler air flows in along the surface to take its place. The warm air then travels toward the cooler regions at higher latitudes. At the higher latitudes, the cooler air sinks and then travels back toward the equator.
On Mars, the general circulation is influenced by the condensation and evaporation of CO2 at the poles. When winter begins, atmospheric CO2 condenses at the pole, and more CO2 flows toward the pole to take its place. When spring arrives, CO2 frost evaporates, and the resulting gas flows away from the pole.
Surface winds on Mars are mostly gentle, with typical speeds of about 6 miles (10 kilometers) per hour. Scientists have observed wind gusts as high as 55 miles (90 kilometers) per hour. However, because the Martian atmosphere is more than 100 times thinner than Earth’s, the gusts there exert much less force than do such high winds on Earth.
Dust storms.
Some of the most spectacular weather occurs on Mars when dust blows in the wind. Small, swirling winds can lift dust off the surface for brief intervals. These winds create dust devils, tiny storms that look like tornadoes.
Large dust storms begin when wind lifts dust into the atmosphere. The dust then absorbs sunlight, warming the air around it. As the warmed air rises, more winds occur, lifting still more dust. As a result, the storm becomes stronger.
At larger scales, dust storms can blanket areas from more than 200 miles (320 kilometers) to a few thousand miles or kilometers across. The largest storms can cover the entire surface of Mars. Storms of that size are unusual, but they can last for months. The strongest storms can block almost the entire surface from view. Such storms occurred in 1971 and 2001.
Dust storms are most common when Mars is closest to the sun. More storms occur then because that is when the sun heats the atmosphere the most.
Satellites.
Mars has two tiny moons, Phobos and Deimos. The American astronomer Asaph Hall discovered them in 1877 and named them for the mythological sons of Ares, the Greek counterpart of the Roman god Mars. Both satellites are irregularly shaped. The largest diameter of Phobos is about 17 miles (27 kilometers). The largest diameter of Deimos is about 9 miles (15 kilometers). Phobos orbits Mars every 7 hours and 39 minutes at a distance of 5,800 miles (9,400 kilometers) from the planet’s center. Deimos orbits much farther from Mars, at 14,550 miles (23,450 kilometers), and takes 30 hours to circle the planet.
The two satellites have many craters that formed when meteoroids struck them. The surface of Phobos also has a complicated pattern of grooves. These may be cracks that developed when an impact created the satellite’s largest crater, called Stickney.
Scientists do not know where Phobos and Deimos formed. They may have come into existence in orbit around Mars at the same time the planet formed. Another possibility is that the satellites formed as asteroids near Mars and were later captured by Mars’s gravitational force. Observations by the United Arab Emirates’ Hope orbiter suggest that the composition of the surface of Deimos is more like that of Mars than like that of an asteroid. The question of the satellites’ origin may be answered by the Japanese Aerospace Exploration Agency (JAXA) Martian Moons eXploration (MMX) mission, which plans to bring back rock samples from the surface of Phobos.
Formation and development
Origin and early evolution.
Mars, like the other planets of the solar system, formed from a giant disk of gas and dust that was circling the sun about 4.6 billion years ago. Gravitational attraction caused particles within the disk to grow by the process of accretion (colliding and sticking together), with larger clumps growing faster as they swept up the smaller particles. Some of these larger clumps became the planets. As the early planets grew, they collided constantly with smaller bodies. Within the planets, radioactive elements such as uranium and thorium decayed, releasing energy in the form of heat. Both the impacts and radioactive decay heated the planets, causing them to partially or completely melt. As a planet melted, the heavier elements sank to its center and lighter compounds rose to the surface. This process, called differentiation, formed the basic structure of Mars and the other rocky planets.
Periods of geologic history.
Features that formed at various stages of Mars’s evolution still exist on different parts of the surface. Researchers have developed a theory of the planet’s past that accounts for the sizes, shapes, and locations of those features.
Researchers rank the relative ages of surface regions according to the number of impact craters observed. The greater the number of craters in a region, the older the surface there. However, scientists have not yet determined exactly when the various evolutionary stages occurred.
Scientists have divided the geologic history of Mars into three periods. From the earliest to the most recent, the periods are: (1) the Noachian << noh AY kee uhn >>, (2) the Hesperian, and (3) the Amazonian.
The Noachian Period
is the oldest period of Martian history. During the Noachian Period, a tremendous number of rocky objects of all sizes, ranging from small meteoroids to large asteroids, struck Mars. The impact of those objects created craters of all sizes. The Noachian was also a time of great volcanic activity. In addition, erosion by water during the Noachian Period probably carved the many small valley networks that mark Mars’s surface. Liquid water requires higher atmospheric pressure and warmer temperatures than are currently present on Mars. The presence of those valleys suggests that the climate may have been warmer during the Noachian Period than it is today.
The Hesperian Period.
The intense meteoroid and asteroid bombardment of the Noachian Period gradually tapered off, marking the beginning of the Hesperian Period. During the Hesperian Period, volcanic activity continued. Volcanic eruptions covered over Noachian craters in many parts of Mars. Most of the largest outflow channels on the planet are of Hesperian age. The Hesperian Period ended about 3 billion years ago.
The Amazonian Period
is characterized by a low rate of cratering. It began around 3 billion years ago and continues to this day. Volcanic activity has occurred throughout the Amazonian Period, and some of the largest volcanoes on Mars are of Amazonian age. The youngest geologic materials on Mars, including the ice deposits at the poles, are also Amazonian. There is little evidence for the existence of liquid water on the surface during this period, except for small gullies and lineae.
Possibility of life
Mars might once have harbored life, and living things might exist there even today. Mars almost certainly has three ingredients that scientists think are necessary for life: (1) chemical elements such as carbon, hydrogen, oxygen, and nitrogen that form the building blocks of living things, (2) a source of energy that living organisms can use, and (3) liquid water.
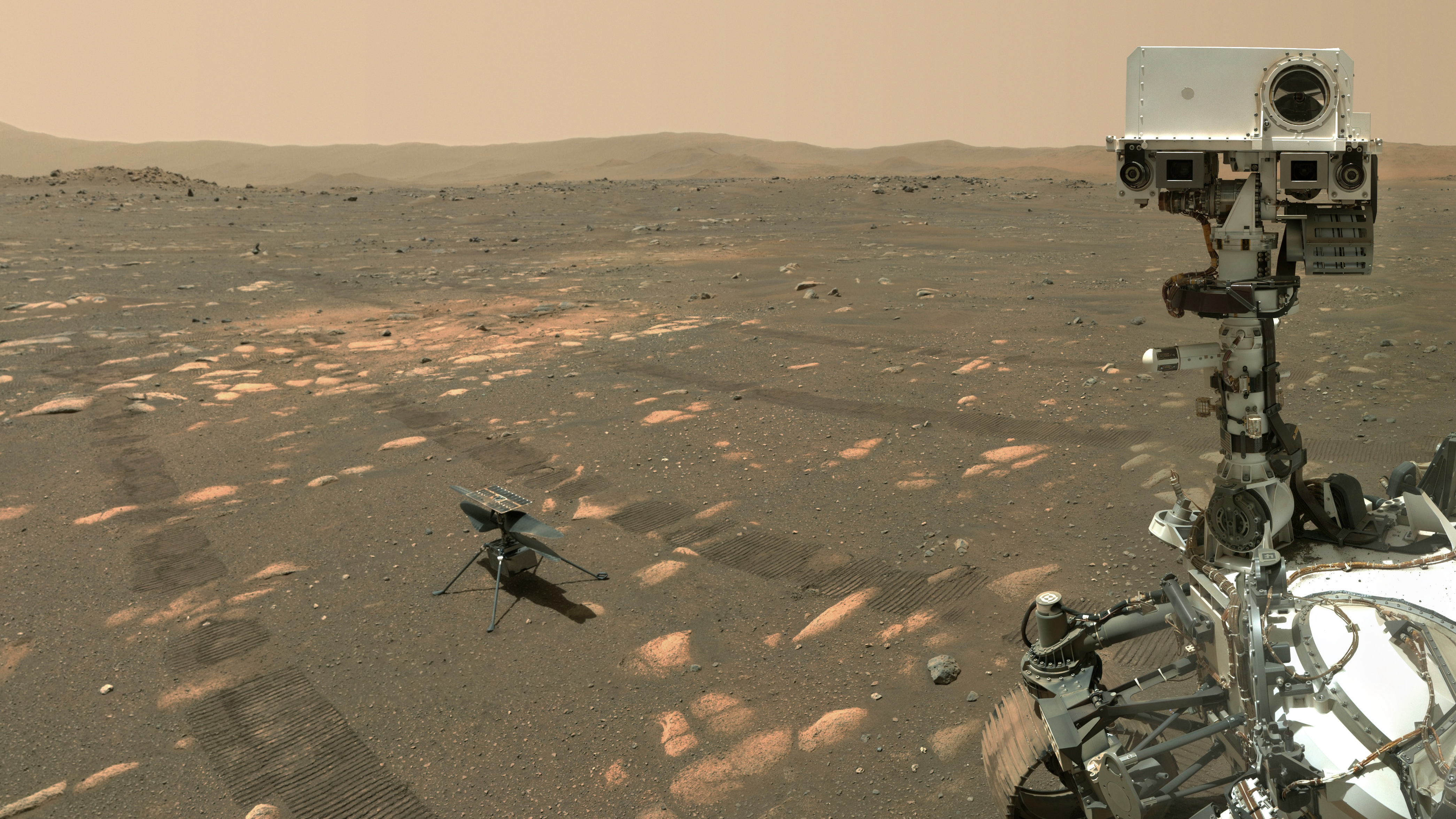
The essential chemical elements likely were present throughout the planet’s history. Sunlight could be the energy source, but a second source of energy could be the heat inside Mars. Earth’s internal heat, for example, supports life in the deep ocean and in cracks in the crust.
Liquid water apparently carved Mars’s large channels, its smaller valleys, and perhaps its young gullies. In addition, there are vast quantities of ice within about 3 feet (1 meter) of the surface near the poles. Thus, water apparently has existed near the surface over much of the planet’s history. And water is probably present beneath the surface today, kept liquid by Mars’s internal heat.
No direct evidence for life on Mars has yet been found. In 1996, some scientists reported that they had found evidence of microscopic Martian life inside a meteorite that had been blasted from the surface of Mars by an impact and made its way to Earth. They found complex organic molecules, grains of a mineral called magnetite that can form within some kinds of bacteria, and tiny structures that resemble fossilized microbes. But there are other, nonbiological explanations for this evidence, and most scientists are not convinced that these features were left behind by living things. Today, there is no general scientific agreement that Mars has ever harbored life.
History of Mars study
Observation from Earth.
Observing Mars through Earth-based telescopes, early astronomers discovered that the planet had polar caps that grow and shrink with the seasons. They also found light and dark markings that appeared to change shape and location.
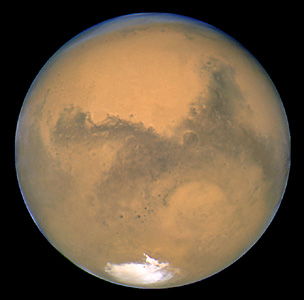
In the late 1800’s, the Italian astronomer Giovanni V. Schiaparelli reported that he saw a network of fine dark lines crisscrossing Mars. He called these lines canali, which is Italian for channels. But canali was generally mistranslated as canals. Many other astronomers also reported seeing such features. Among those observers was the American astronomer Percival Lowell, who referred to the features as canals. Lowell speculated that the “canals” had been built by a Martian civilization, and that the changing patterns might result from the growth and death of vegetation.
Later observers with more powerful telescopes failed to detect any canals. Schiaparelli, Lowell, and others seem to have misinterpreted the faint, blurry images of Mars that their telescopes produced, perhaps as a result of the mind’s natural tendency to search for patterns. However, the changing dark and light markings were real. Scientists now know that Martian winds blow light and dark dust across the surface, changing the planet’s appearance with the seasons. Scientists continue to use Earth-based telescopes to study Mars, particularly the planet’s atmospheric composition and global seasonal changes.
Observation by spacecraft.
Mars has been a target for robotic exploration since the beginning of the space age in the mid-1900’s, with dozens of spacecraft sent there by different countries. But exploring Mars with robotic spacecraft is extremely difficult. Over the years, about half of all missions sent to Mars have failed before completing most of their planned observations.
Early exploration.
Robotic spacecraft began detailed observation of Mars in the 1960’s. The United States sent the Mariner 4 probe to Mars in 1964 and Mariners 6 and 7 in 1969. The Soviet Union successfully sent the Mars 2 and 3 probes in 1971, and the Mars 5 probe in 1973. The pictures these spacecraft initially returned showed a barren surface covered with craters like those on the moon. There was no sign of liquid water or life. The spacecraft observed few of the planet’s most interesting features because they happened to fly by only heavily cratered regions. But when Mariner 9 went into orbit around Mars in 1971, people’s view of Mars completely changed. Mariner 9 mapped about 80 percent of Mars and made the first discoveries of the planet’s canyons and volcanoes. It also found the first evidence for water, taking photographs of the outflow channels and valley networks.
Viking, an ambitious mission with the goal of landing on Mars and searching for life, was launched by the United States in 1975. It consisted of two orbiters and two landers. The orbiters scouted out landing sites for the landers, which touched down in July and September 1976. The landers took the first close-up pictures of the Martian surface and analyzed the soil. They found no strong evidence for life.
Later exploration by orbiters.
Since the mid-1990’s, a number of orbiters have been sent to Mars. These probes have carried a wide range of sophisticated scientific instruments and have produced a detailed global understanding of the planet.
The U.S. Mars Global Surveyor operated from 1996 to 2006. Its high-resolution camera revealed layered sediments that may have been deposited in liquid water and small gullies that may have been carved by water. An altimeter (device for measuring elevation) used laser beams to map the entire surface. A magnetometer confirmed that Mars has no global magnetic field, but mapped magnetic variations in the crust.
NASA's Journey To Mars
The U.S. Mars Odyssey probe, launched in 2001, carries instruments to analyze the chemical composition of the Martian surface and the rocks just below the surface. It discovered vast amounts of water ice within a few feet (1 meter) of the surface.
The European Space Agency (ESA) probe Mars Express arrived at the planet in 2003. It has taken stunning three-dimensional pictures of the planet’s surface and mapped the composition of surface rocks using a device called an infrared spectrometer. It also carries a radar instrument designed to search for underground deposits of water. Its instruments detected traces of methane in the Martian atmosphere, a possible indicator of life, and confirmed the presence of large amounts of water ice in the planet’s southern region. Scientists estimate the total volume of water ice in the south polar region at about 0.4 million cubic miles (1.6 million cubic kilometers), enough to cover Mars with a layer of water about 36 feet (11 meters) deep.
The Mars Reconnaissance Orbiter, launched by the United States in 2005, carries the highest resolution camera ever sent to Mars, capable of resolving (distinguishing) objects on the planet’s surface less than a meter (3 feet) across. Its high-resolution images helped to identify safe landing sites for subsequent landers and discovered recurring slope lineae. Its payload also includes a high-resolution spectrometer that has mapped the occurrence of minerals that are formed in association with water; an instrument that measures temperatures, pressures, and dust concentrations throughout the atmosphere; and radar that can detect layered rocks and ice beneath the surface.
The MAVEN mission, launched in 2013, is dedicated to understanding the interactions between Mars’s upper atmosphere and the solar wind. This U.S. probe’s instruments have studied the ways that air molecules are lost from Mars. Knowing how the molecules are lost gives scientists strong clues about how an early thick atmosphere, which seems to have made liquid water possible on the surface, could have disappeared over time.
India’s Mars Orbiter Mission (also called Mangalyaan) is the first successful Mars mission by an Asian country. It overcame a minor engine failure to reach Martian orbit in 2014. It has returned many exciting color photographs of the Martian surface and carries instruments to observe Mars’s surface and to search for the presence of methane gas in the planet’s atmosphere.
The Trace Gas Orbiter mission is a collaboration between the ESA and Russia, launched in 2016. The main goal of this mission is to better understand methane and other gases in the Martian atmosphere that could be evidence of biological or geological activity.
In 2021, a probe called Hope (Amal in Arabic), developed by the United Arab Emirates (UAE), entered into orbit around Mars. The UAE became just the fifth country to reach the planet. The orbiter maps Martian weather on a planet-wide scale.
Later exploration by landers.
Twenty years after the launch of Viking, surface exploration resumed with the launch of Mars Pathfinder in 1995. Pathfinder consisted of a lander that carried a small roving vehicle called Sojourner. The rover rolled down a ramp to the surface and then moved from rock to rock. Pathfinder sent spectacular close-up photos back to Earth, and Sojourner analyzed rocks and soil.
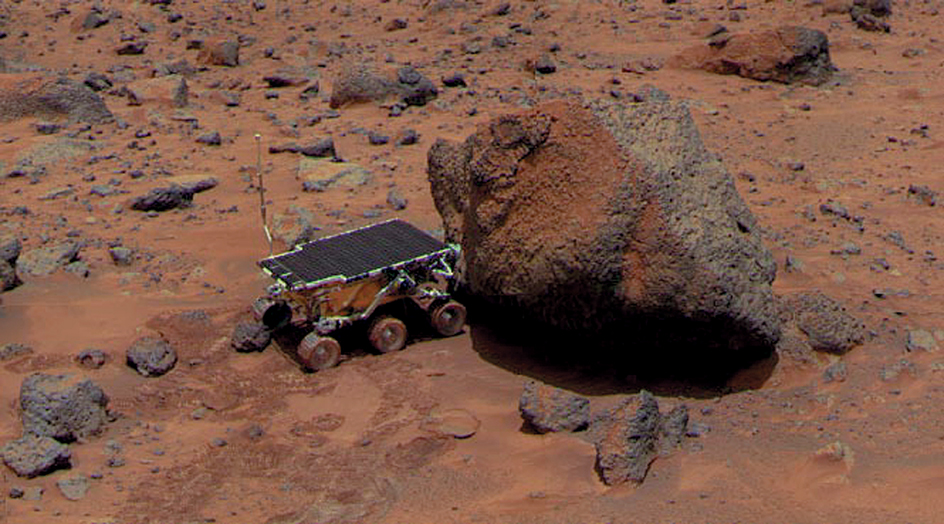
In 2004, two U.S. Mars Exploration Rovers landed safely on Mars. The rover Spirit set down in an area called Gusev Crater, and Opportunity landed in an area called Meridiani Planum. The rovers were originally designed for a 90-day mission. But Spirit continued to gather information on the surface of Mars for five years. Opportunity continued to operate until it was knocked out by a dust storm in 2018. The rovers transmitted detailed photographs of Martian ground features. They found the first conclusive evidence that large amounts of liquid water once existed on the planet’s surface.
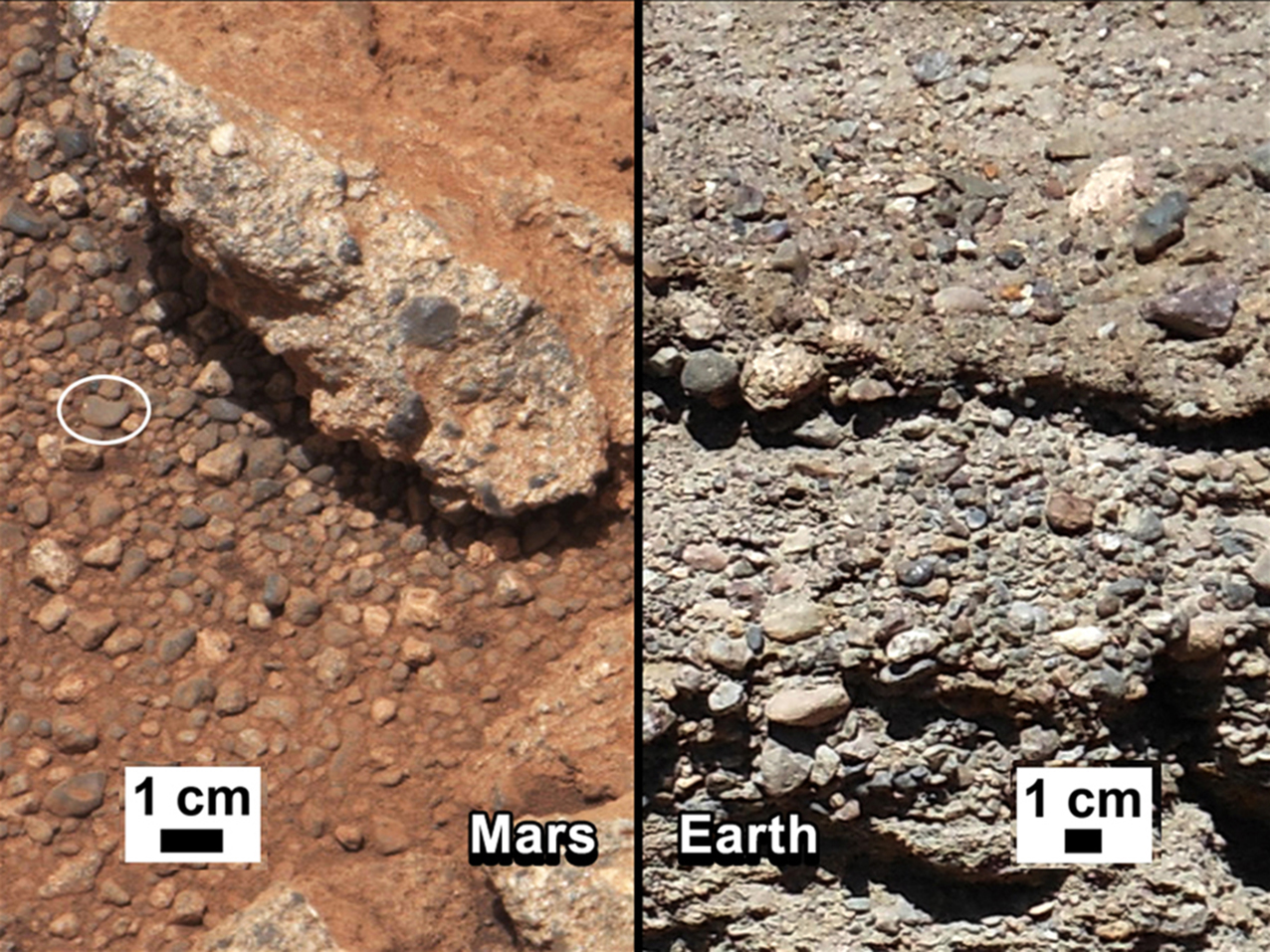
The United States Phoenix lander operated in the north polar region of Mars from May to November 2008. The lander revealed the presence of various minerals in the soil. Chief among its contributions was confirming the existence of water ice just below the surface of the Martian soil.
The U.S. Mars Science Laboratory (MSL) descended to the surface of Mars in 2012. The rover, nicknamed Curiosity, is roughly the size of an automobile and is one of the largest rovers to reach the Martian surface. The rover’s primary goal is to examine the rocks and soils around its landing site to determine if the conditions for supporting life ever existed on Mars. Curiosity found sulfur, nitrogen, oxygen, phosphorus, and organic carbon molecules—the key ingredients necessary for life—in powdered samples drilled from a rock. The samples also revealed clay minerals and not too much salt, suggesting that fresh, possibly drinkable water once flowed there. Curiosity also observed a tenfold increase in methane concentrations over a two-month period. Methane can be produced by living organisms or by chemical reactions between rock and water.
The U.S. InSight lander operated on Mars from 2018 to 2022. InSight stands for Interior Exploration Using Seismic Investigations, Geodesy, and Heat Transport. The lander’s instruments recorded data on Mars’s crust and interior to help answer questions about how the planet formed. InSight recorded more than 1,300 marsquakes over several years. It also took weather measurements. InSight ran out of power in 2022 due to an accumulation of dust on its solar panels.
Mars 2020, nicknamed Perseverance, is another robotic space mission based on the MSL rover design. It was launched in July 2020 and landed on Mars in 2021. Perseverance is the largest rover ever to land on Mars. It is the size of a small automobile and weighs over 2,200 pounds (1,000 kilograms) on Earth. Perseverance is exploring an ancient river delta in the Jezero Crater, where it searches for signs of past life. It also studies the geology and climate of the region and collects rock and soil samples. These samples may be brought back to Earth by a future NASA mission. In 2021, the Ingenuity Mars Helicopter, which Perseverance carried to Mars, became the first powered aircraft to fly on another planet.
In early 2021, the Chinese spacecraft Tianwen-1 entered orbit around Mars, making China the sixth country to reach the planet. The mission consists of an orbiter, a lander, and a rover. The Tianwen-1 rover—named Zhurong, after an ancient Chinese god of fire—landed on Mars in May 2021.
Future human exploration
Although astronauts have been to Earth’s moon, no humans have yet traveled to Mars. Mars is more than 500 times farther away than the moon, and astronauts would have to live in deep space for at least six months as they travel in each direction, all the time exposed to the danger of radiation from solar flares. To return from a surface visit, astronauts would also have to land with a rocket capable of lifting off again. Such a vehicle would be much larger than any that has yet been sent to the surface of Mars.
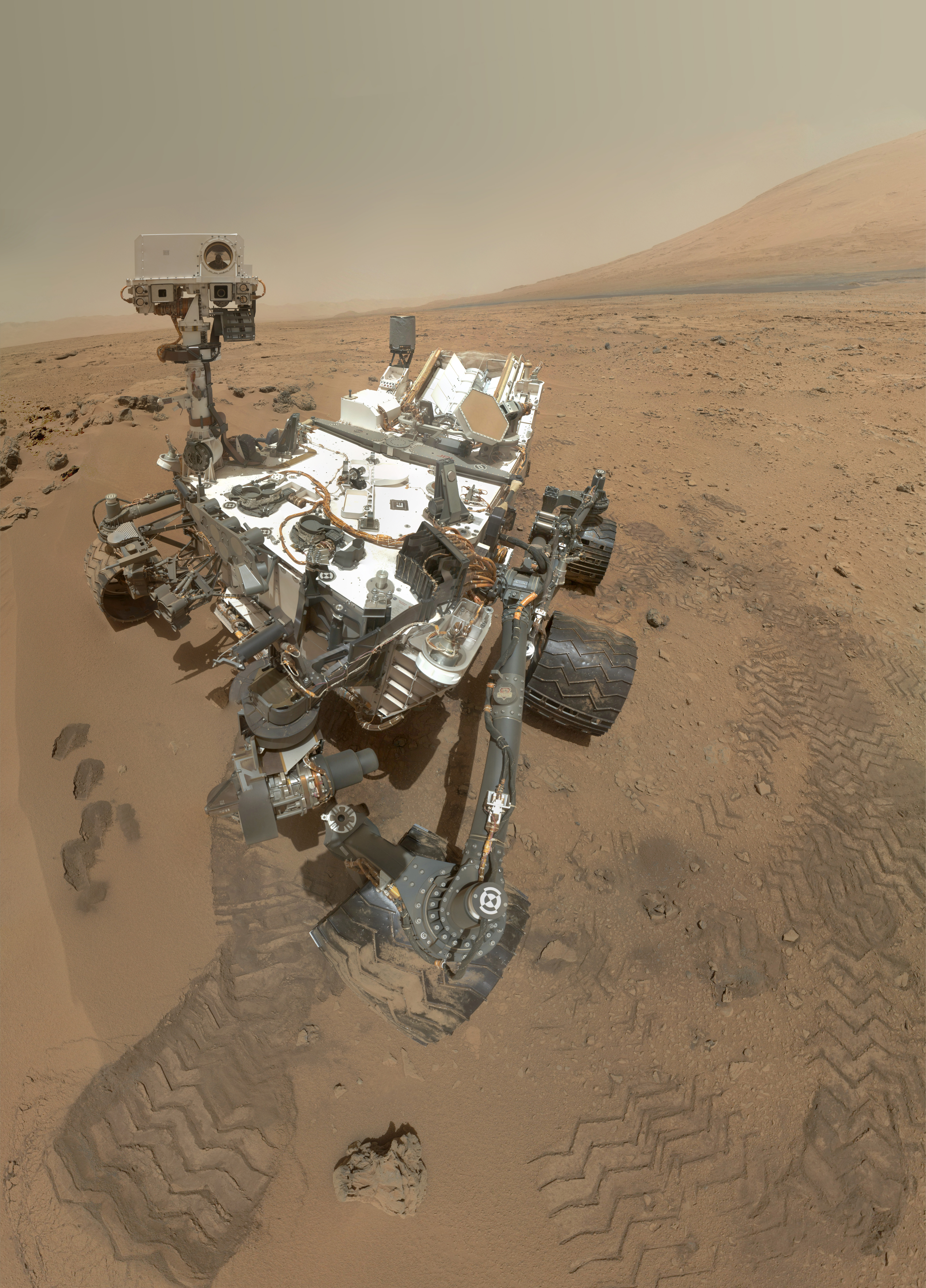
Robotic spacecraft are in many ways extremely effective in exploring a planet. They can be sent to places that would be too dangerous for humans. The cost of a robot is much less than that of the complex systems necessary to support and protect humans. But robotic explorers have limitations. They are generally best at doing predetermined activities and have limited capability to adapt to new situations. In addition, progress with remotely operated robots is slow. For comparison, the astronauts of the Apollo 17 mission drove almost 23 miles (37 kilometers) in their three-day stay on the moon. It took the Opportunity rover almost 10 years to drive that far.
The United States is developing the capabilities needed to support the human exploration of Mars and has a goal of sending people to Mars in the 2030’s. Engineers and scientists are working to develop the technologies astronauts can use to one day travel to Mars, live and work on its surface, and safely return home.