Neutrino is any of three types of subatomic particles that have no electric charge. Experiments have shown that neutrinos have some mass (amount of matter). However, the masses of neutrinos are so small that scientists have not yet been able to measure them directly. A neutrino has no more than 1 millionth the mass of an electron, the lightest particle that has an electric charge.
Neutrinos travel near the speed of light. They are produced in reactions that occur in the decay (breaking apart) of unstable subatomic particles or nuclei. They are also created in reactions that produce energy in the sun and other stars. In addition, machines known as particle accelerators can produce neutrinos.
Neutrinos belong to the lepton family of particles. The other leptons carry an electric charge and have mass. They are, from the lightest to the heaviest, the electron, the muon, and the tau.
A neutrino can change into a charged lepton by interacting with an atomic nucleus. Each type of neutrino can change into only one kind of charged lepton. The names of the three types of neutrinos come from the names of the corresponding charged leptons. Thus, an electron-neutrino can change into an electron, a muon-neutrino into a muon, and a tau-neutrino into a tau.
The neutrinos also have antimatter counterparts called antineutrinos that differ from neutrinos in the direction of their spin. Spin is a measure of the internal rotation of a particle. There are electron-antineutrinos, muon-antineutrinos, and tau-antineutrinos.
The American physicists Frederick Reines and Clyde Cowan announced the discovery of the first neutrinos, electron-neutrinos, in 1956. In 1962, the American physicists Leon M. Lederman, Melvin Schwartz, and Jack Steinberger discovered the muon-neutrino. In 2000, an international team of scientists at Fermi National Accelerator Laboratory (Fermilab) in Batavia, Illinois, announced the first direct evidence for the tau-neutrino.
Neutrinos interact with other particles through a force called the weak interaction. This force is so feeble that neutrinos can pass through a large amount of matter with only a tiny chance of interacting with a particle in the matter. So, to detect enough neutrino interactions for study, researchers must build enormous detectors.
A detector senses a neutrino indirectly, after the neutrino interacts with a nucleus or collides with an electron. The interaction or collision releases charged particles that electronic devices can detect. But the devices can also detect other charged particles at Earth’s surface. Therefore, to shield detectors from the other particles, scientists build them deep underground.
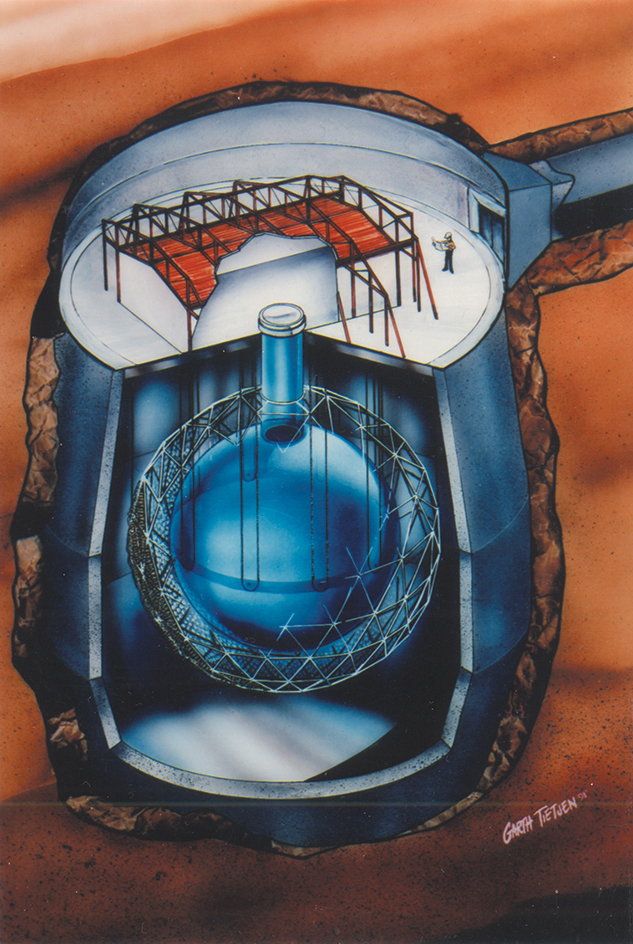
The most sensitive neutrino detector is the Sudbury Neutrino Observatory (SNO), which began operating in 1999 in a nickel mine in Ontario. The SNO detects several neutrino interactions per day. The Super-Kamiokande (Super-K) detector, in a zinc mine northwest of Tokyo, began operating in 1996 and has produced much valuable data. In 2005, scientists began building the IceCube Neutrino Detector near the South Pole. It began collecting data that same year but was not completed until 2010. IceCube is 1 cubic kilometer (0.24 cubic mile) in size and 1.4 kilometers (0.9 mile) below the ice.
Neutrino detectors have consistently observed fewer neutrinos from the sun than expected. Data from the SNO show that the shortage occurs because electron-neutrinos created in the sun change into other varieties as they travel through space. This effect is called oscillation. Data from the Super-K detector likewise show that neutrinos created in Earth’s upper atmosphere oscillate into other types. Oscillation can occur only if neutrinos have mass. In 2015, two neutrino physicists— Takaaki Kajita of Japan and Arthur Bruce McDonald of Canada—shared the Nobel Prize in physics for confirming that neutrinos oscillate.