Periodic table is a chart that lists the known chemical elements arranged according to their characteristics. The periodic table ranks as one of the most useful tools in chemistry and perhaps in all of science. No other scientific field has such a simple but descriptive means of representing its fundamental units. The version of the table commonly used today organizes the elements into rows and columns. Elements in the same column share certain chemical properties (characteristics). These properties repeat row by row in a regular or periodic fashion, giving the table its name.
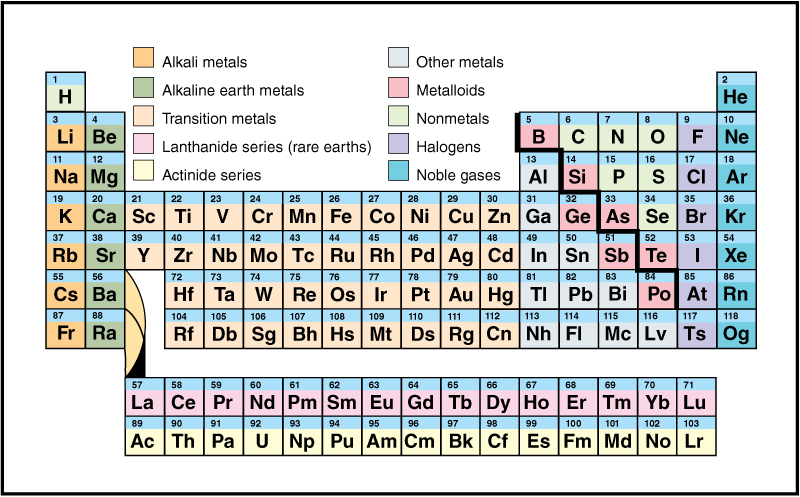
Each element’s entry in the periodic table lists some basic information about the element. Most versions of the table include the element’s name, chemical symbol, atomic number, and relative atomic mass. An element’s atomic number equals the number of protons in the nucleus of a single atom of the element. Its relative atomic mass represents the average mass (amount of matter) of all stable isotopes (forms) of the element, weighted according to each isotope’s abundance in nature. If an element has no stable isotopes, most periodic tables list the atomic mass number of the longest-lived isotope. An isotope’s atomic mass number equals the total number of protons and neutrons in a nucleus of that isotope. Some periodic tables also show the configuration of electrons in an atom of the element or whether the element occurs as a solid, liquid, or gas at room temperature.
Elements in the same region of the periodic table tend to have similar chemical and physical properties. For example, except for hydrogen, all of the elements on the left and center of the table are metals. With few exceptions, these elements have a shiny, silvery appearance and readily conduct heat and electric current. They are ductile (able to be drawn into wires) and malleable (able to be pounded into thin sheets). They can mix with other metals to make materials called alloys. Nonmetals, on the other hand, mostly occupy the upper right corner of the table. They vary widely in appearance, they do not conduct heat or electric current well, and many of them are brittle when solid. Although hydrogen appears on the top left side of the table, chemists typically group it with the nonmetals.
Structure of the periodic table
The periodic table orders the elements primarily by atomic number. The numbers begin with hydrogen (atomic number, 1) in the upper left corner. Proceeding left to right along each row, called a period, atomic number increases. When a period ends, the progression continues at the beginning of the next period down.
The division of the elements into periods reflects the basic structure of the atom. Electrons orbit the atomic nucleus in layers called shells. Each period on the table corresponds to one of these shells. The first period, for example, consists of just two elements, hydrogen and helium. Atoms of these elements have only a single shell of electrons.
Atoms tend to have the same number of electrons as protons. Therefore, as atomic number increases, more electrons are added to the shells. Hydrogen generally has one electron, which orbits in the first shell. Helium (atomic number, 2) has two electrons in the first shell. The first shell can hold only two electrons, so any additional electrons will form a second shell. Lithium (atomic number, 3) generally has three electrons. Two occupy the first shell, while the third forms a second shell. Lithium thus begins the second period, which runs through neon (atomic number, 10). Each element in the second period has an increasing number of electrons in the second shell. The elements of the third period—sodium through argon—have three shells, and so on.
Chemists refer to the columns of the periodic table as groups or families. Each group can be labeled in one of two ways. For simplicity, the International Union of Pure and Applied Chemistry (IUPAC), the recognized authority on chemical terminology, recommends that the 18 groups be numbered from left to right using the Arabic numerals 1 through 18. Many chemists, however, continue to use a more complex system based on Roman numerals. In a common version of this system, the group 1 elements—hydrogen, lithium, sodium, potassium, rubidium, cesium, and francium—are labeled group IA. The group 18 elements—helium, neon, argon, krypton, xenon, radon, and oganesson—are known as the group VIIIA elements. Under this system, from left to right, the 18 groups read: (1) IA, (2) IIA, (3) IIIB, (4) IVB, (5) VB, (6) VIB, (7) VIIB, (8) VIII, (9) VIII, (10) VIII, (11) IB, (12) IIB, (13) IIIA, (14) IVA, (15) VA, (16) VIA, (17) VIIA, and (18) VIIIA.
Like the periods, the groups also reflect the configuration of electrons in the atom. The electrons in each shell occupy smaller subshells, often labeled s, p, d, and f. Electrons tend to occupy the s subshell first, followed by p, d, and finally f. For example, each of the elements in Group 1 have one electron in their outermost s subshell. Moving one group to the right adds another electron to the outermost s subshell, filling it out. For this reason, chemists refer to Group 1 and Group 2 collectively as the s block.
Other regions of the periodic table correspond to other subshells. The rightmost six groups, known as the p block, represent the progressive addition of electrons to fill the outermost p subshell. Chemists often refer to the s and p blocks collectively as the main group or representative elements because these elements display a fairly predictable progression in chemical properties. The 10 columns between the s and p blocks are called the d block because they correspond to the filling of the outermost d subshell. The 14 columns that make up the f block are typically written separately from the rest of the table.
The periodic table and properties
The organization of the periodic table summarizes chemical properties that determine how an element will react to form compounds. The table also reveals important trends in the physical properties of the elements.
Chemical properties.
Atoms react with other atoms to form chemical bonds by gaining, losing, or sharing electrons in their outermost or valence shells. Because the elements in a group typically have the same number of valence electrons, they tend to bond in similar ways.
Ionic bonding
occurs when atoms gain or lose valence electrons to become charged particles called ions. An atom that loses one or more electrons becomes a positively charged ion called a cation. An atom that gains one or more electrons becomes a negatively charged ion called an anion. The positive charge of the cation and the negative charge of the anion can attract each other, forming an ionic bond. In general, metals react with nonmetals to form ionic bonds. For example, the metal sodium reacts with the nonmetal chlorine to form the ionic compound sodium chloride.
An element’s group on the periodic table helps determine what charge it will adopt in an ionic compound. Atoms from group 1 tend to lose their single valence electron, forming ions with a 1+ charge. Atoms from group 2 generally lose both valence electrons, acquiring a 2+ charge.
On the right side of the table, atoms tend to gain electrons, taking on a negative charge. Atoms in group 17, for example, tend to take on an additional electron, becoming ions with a 1– charge. Group 16 atoms take on a 2– charge, and group 15 atoms acquire a 3– charge.
Covalent bonding
occurs when atoms share one or more pairs of electrons. Each atom contributes one electron to the pair, forming a covalent bond. Nonmetals typically react with one another to form covalent compounds. For example, the nonmetals hydrogen and oxygen react to form water, a covalent compound.
An atom’s group on the periodic table determines the number of valence electrons it can share and thus the number of covalent bonds it can form. Group 17 atoms can share one pair of electrons, forming one covalent bond. Group 16 atoms normally make two covalent bonds, while group 15 atoms tend to make three bonds.
Physical properties.
With few deviations, the arrangement of elements on the periodic table also reveals clear trends in their physical properties. These properties include: (1) atomic radius, (2) ionization energy, (3) electron affinity, and (4) electronegativity.
Atomic radius
is the approximate size of an atom of a given element. Moving down a group, atomic radius tends to increase because each atom features a progressively higher number of electron shells. These extra shells lie farther and farther from the nucleus. Moreover, in atoms with more than one electron shell, the negatively charged electrons in the inner layers tend to repel the negatively charged electrons in the outer layer. This repulsion effectively shields outer electrons from the attractive pull of the positively charged nucleus.
Proceeding from left to right across a period, atomic radius tends to decrease. This trend occurs because elements in the same period have the same number of electron shells, minimizing the effect of the shielding. Within a period, an increase in the number of protons and electrons usually results in a greater attractive force between the nucleus and the electrons, decreasing the atomic radius. Thus, helium ranks as the smallest atom, and francium ranks as the largest atom yet known.
Ionization energy
is the energy required to remove a valence electron from an atom. Trends in ionization energy run opposite those seen in atomic radius. Proceeding across a period, ionization energy tends to increase. This trend occurs because the attractive force between the nucleus and the electrons generally increases, making the electrons more difficult to remove. Going down a group, ionization energy decreases because the nucleus usually exerts a weaker hold on the additional electrons. Helium, therefore, has the largest ionization energy of any element, and francium has the lowest ionization energy of any element yet known.
Electron affinity
represents the energy change that occurs when an electron is added to a neutrally charged atom. Not including the alkaline earth metals, which make up group 2, and the noble gases, which make up group 18, electron affinity increases from left to right across a period. This trend occurs because the attractive force between the positively charged nucleus and the negatively charged electrons tends to increase. Thus, the added electron is pulled more strongly toward the nucleus, causing it to give off more energy. Electron affinity generally decreases moving down a group because additional electrons usually orbit farther and farther from the nucleus, shielding them from its attractive force. The alkaline earth metals and the noble gases have unusually low electron affinities because their electron configurations are quite stable. Adding an electron to a noble gas actually requires energy.
Electronegativity
measures how strongly an atom attracts the shared pair of electrons in a covalent bond. In any such bond, the more electronegative atom will pull on the shared electrons more strongly than the less electronegative atom. Trends in electronegativity tend to reflect those seen in electron affinity and ionization energy. Except for the noble gases, electronegativity generally increases to the right of a period and tends to decrease moving down a group. The noble gases have extremely low electronegativities. Fluorine ranks as the most electronegative element.
Classes of the periodic table
Chemists often divide the elements into classes based on shared properties. Many of the classes correspond to a specific area of the periodic table. Publishers often mark these classes using color. Although the classes shown in this way can vary, they often include: (1) alkali metals, (2) alkaline earth metals, (3) transition metals, (4) the lanthanide series, (5) the actinide series, (6) other metals, (7) metalloids, (8) nonmetals, (9) halogens, and (10) noble gases. Some of these groups overlap one another.
Alkali metals
include all the group 1 elements except hydrogen. They are: lithium, sodium, potassium, rubidium, cesium, and francium. In their elemental (pure) form, these metals appear unusually soft and light. They are extremely chemically reactive, reacting spontaneously and vigorously with water. Chemical reactivity increases moving down the group. Because of their reactivity, alkali metals never appear in their elemental form in nature. They only occur in compounds.
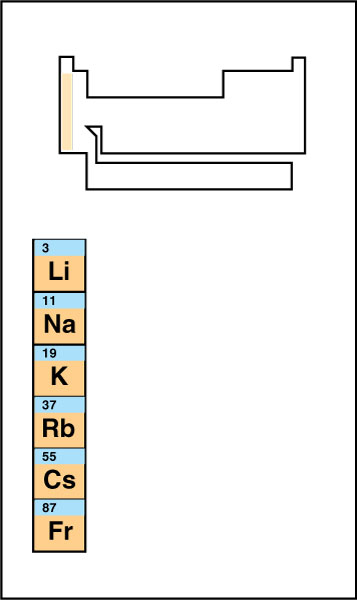
Alkali metals easily lose their single valence electron, becoming cations with a 1+ charge. Cations of two alkali metals, sodium and potassium, serve important functions in the human body. See Alkali metal.
Alkaline earth metals
make up group 2 of the periodic table. They include beryllium, magnesium, calcium, strontium, barium, and radium. They are harder than the alkali metals, with which they share the s block. Excluding beryllium, the alkaline earth metals also react with water, but less vigorously. They never occur in their elemental form in nature, appearing only in compounds.
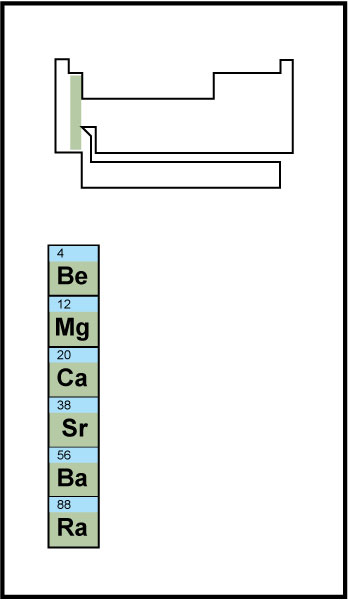
Atoms of the alkaline earth metals easily lose their two valence electrons. In compounds, they therefore tend to form cations with a 2+ charge. Manufacturers use magnesium, which is extremely light and strong, in many alloys. Calcium serves as an important component of bone tissue.
Transition metals
traditionally occupy the entire d block of the periodic table. Transition metal atoms can lose different numbers of valence electrons, forming cations that have different charges and thus different chemical properties. For example, iron atoms commonly lose either two or three electrons, adopting either a 2+ or a 3+ charge.
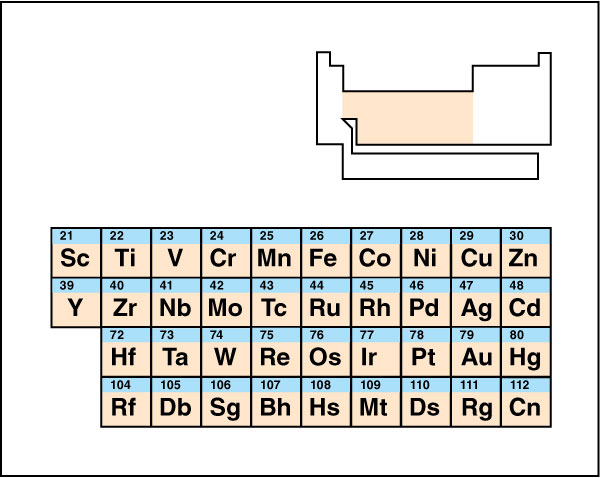
Because transition metals can form cations with different charges, their chemistry can be especially unpredictable. Compounds of transition metals feature a wide variety of colors.
The lanthanide series
consists of the top row of the f block. It begins with lanthanum, which gives the series its name, and continues through lutetium. Some scientists do not include lanthanum in the lanthanide series. To avoid excessive width in the periodic table, publishers usually display the lanthanides separately from the rest of the elements.
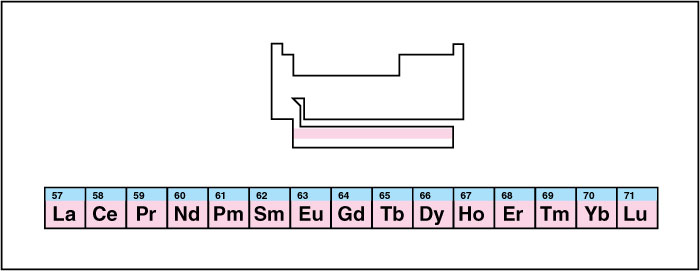
The lanthanide elements all have extremely similar chemical reactivities. This property makes it difficult to isolate individual lanthanide elements from ores that contain several of them. Lanthanides are used in many products, such as catalytic converters, lasers, lenses, lighters, magnets, metal alloys, nuclear reactors, phosphors, pigments, and X-ray intensifying screens. Chemists sometimes call the lanthanides the rare earth metals. See Rare earth.
The actinide series
occupies the bottom row of the f block. It begins with actinium, which gives the series its name, and continues through lawrencium. Some scientists do not include actinium in the actinide series. Along with the lanthanides, publishers usually list the actinides separately to prevent the periodic table from becoming too wide. See Actinide.
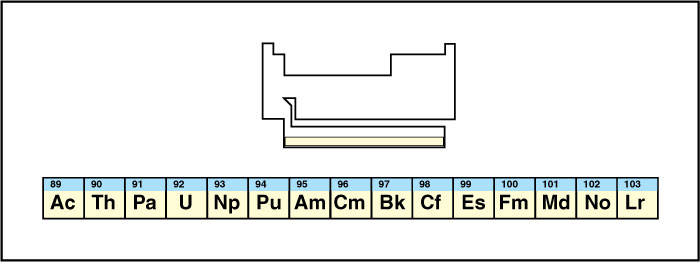
All the actinides are radioactive, and many of them are highly toxic. Some of them, especially uranium, serve as fuel in nuclear power plants. Scientists artificially produced all the elements beyond uranium, although tiny amounts of neptunium and plutonium exist in nature. One actinide, americium, serves as an important component in household smoke detectors.
Other metals
make up the lower left side of the p block. They include aluminum, gallium, indium, thallium, tin, lead, and bismuth. Some chemists include polonium in this class. Nihonium, flerovium, moscovium, and livermorium are presumed to be part of this group as well, but they are so unstable that little is known about their properties. The other metals are less chemically reactive than the alkali and alkaline earth metals. Unlike transition metals, they do not form ions with a variety of charges. As a result, their chemistry is more predictable. The other metals are also generally softer than the transition metals.
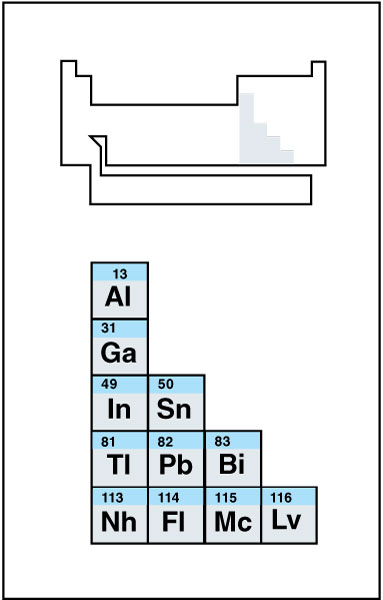
Metalloids
have some metallic and some nonmetallic properties. They appear along a staggered line in the p block that separates the metals from the nonmetals. Some periodic tables indicate the metalloids using a special color. Others use an especially heavy line to separate the metals and nonmetals. The metalloids lie along that line. People sometimes refer to the metalloids as the semimetals.
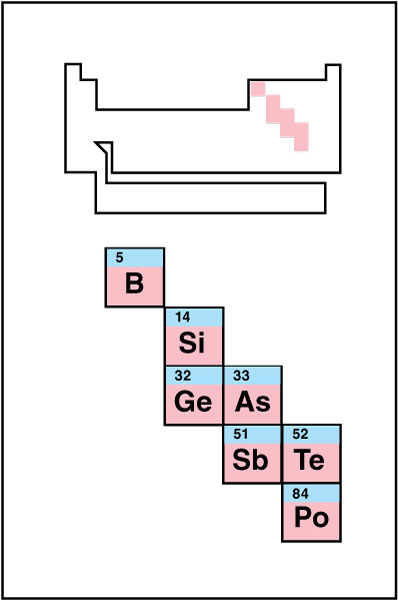
Silicon is an example of a metalloid. It has a silvery appearance like a metal but conducts heat and electric current poorly like a nonmetal.
Nonmetals
occupy the upper right corner of the p block. This small class of elements features an unusually wide variety of appearances. At room temperature, different nonmetals appear as solids, liquids, and gases. They take on a variety of colors from yellow sulfur to bluish-black iodine. The nonmetals do not conduct electric current or heat well and are brittle in solid form. Although the element hydrogen appears on the left side of the periodic table, chemists generally include it in this group.
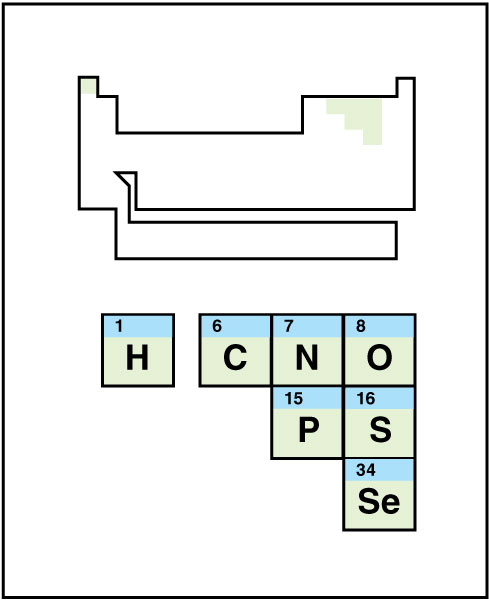
Nonmetals generally react with other nonmetals to form covalently bonded molecules. They react with metals by gaining electrons to become anions of various charges.
Halogens
make up group 17 of the nonmetals. Halogens << HAL uh juhnz >> include fluorine, chlorine, bromine, iodine, astatine, and tennessine. No other group features a wider range of physical states—at room temperature, fluorine and chlorine are gases, bromine is a dark red liquid, and iodine is a bluish-black solid. Astatine, which is radioactive, has never been prepared in sufficient quantities to be visible to the unaided eye. Only a handful of atoms of tennessine has ever been produced. Little is known of the properties of this element. The reactivity of halogens increases toward the top of the group. In fact, fluorine ranks as the most chemically reactive element.
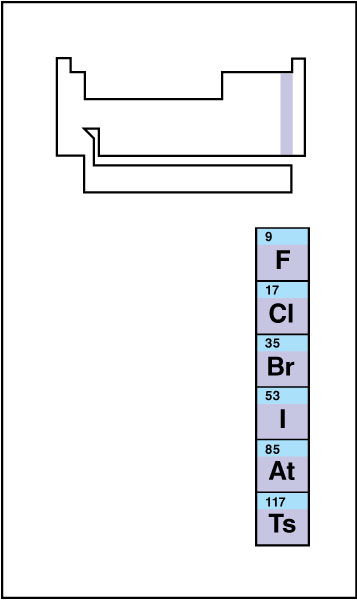
All of the halogens—probably even astatine and tennessine—exist in their elemental form as diatomic molecules (molecules made up of two atoms). For example, fluorine gas consists of pairs of fluorine atoms bound covalently to each other. In nature, however, halogens never occur in their elemental form. Instead, they exist largely in the form of ionic compounds known as salts. In ionic form, these elements gain an electron to adopt a 1– charge. Chlorine serves as an important ion in the human body. Iodine has a highly specialized function in the body, where it appears almost exclusively in several hormones produced by the thyroid gland. See Halogen.
Noble gases
occupy group 18. They include helium, neon, argon, krypton, xenon, radon, and oganesson. Chemists used to call them inert gases because it was thought that they did not form compounds. However, scientists now know that most of the noble gases will form compounds under specialized conditions. Because they do not react readily with other elements, noble gases were the last group of elements discovered. In fact, chemists added them as an extra column after the table had already been developed.
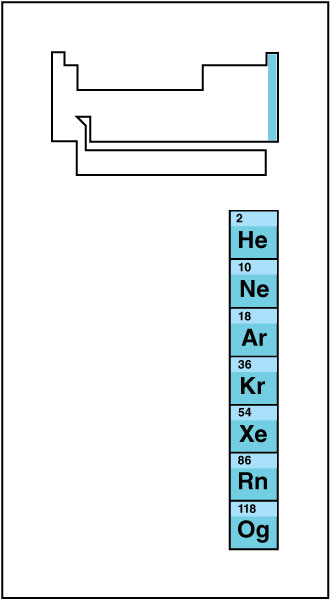
The noble gases’ lack of reactivity makes them useful in many applications. For example, manufacturers fill light bulbs with argon gas because it will not corrode the metal parts of the bulb. Because helium is lighter than air and does not burn, people can safely use helium in balloons, blimps, and other airships. People also use noble gases in devices called gaseous-discharge lamps. These lights use electric current to excite the gas, producing light of various colors. True “neon” lights—those filled with neon—glow reddish-orange. The other noble gases also give off their own characteristic colors.
Other classes.
Some less commonly used designations overlap one or more of the major classes. To avoid confusion, periodic tables typically do not set off these other classes using color.
Some chemists refer to the group 15 elements as the pnictogens << puh NIHK tuh juhnz or NIHK tuh juhnz >> or pnicogens. They include nitrogen, phosphorus, arsenic, antimony, and bismuth. They commonly form covalent compounds with either three or five bonds. When these elements do form ions, they typically take on a 3– charge.
Chemists refer to the group 16 elements as the chalcogens << KAL kuh juhnz >>. The chalcogens include oxygen, sulfur, selenium, tellurium, and polonium. The chalcogens can form ionic or covalent compounds. In ionic compounds, they take on a characteristic 2– charge, while in covalent compounds they typically form two chemical bonds.
Other collections of elements have special names, but none of these are in common use. Chemists sometimes refer to groups 8, 9, and 10 in the fifth and sixth periods as the platinum group. The platinum group includes ruthenium, osmium, rhodium, iridium, palladium, and platinum. All of these elements are chemically resistant and have high melting points. People sometimes refer to the group 11 elements—copper, silver, and gold—as the coinage metals because they are used in coins. However, coins are also made of other metals, such as zinc, lead, and steel. Because the coinage metals resist corrosion relatively well, they have also been called the noble metals.
History
The French chemist Antoine Lavoisier first established the modern concept of the element in the late 1700’s. As more and more elements were discovered, chemists noticed that the elements show certain chemical similarities and trends in properties. For example, the metals lithium, sodium, and potassium form compounds with oxygen in which there are always two units of metal for every one unit of oxygen. Similarly, the nonmetals chlorine, bromine, and iodine form compounds that have one unit of sodium for every unit of nonmetal.
In a paper published in 1829, the German chemist Johann Döbereiner grouped some elements into triads (groups of three) based on the similarities of their chemical properties. Döbereiner’s triads included lithium, sodium, and potassium; chlorine, bromine, and iodine; and calcium, strontium, and barium. After the discovery of more elements, the English chemist John Newlands noted around 1865 that if the elements are arranged according to their atomic masses, their chemical properties repeat after eight elements. Newlands called this principle the law of octaves.
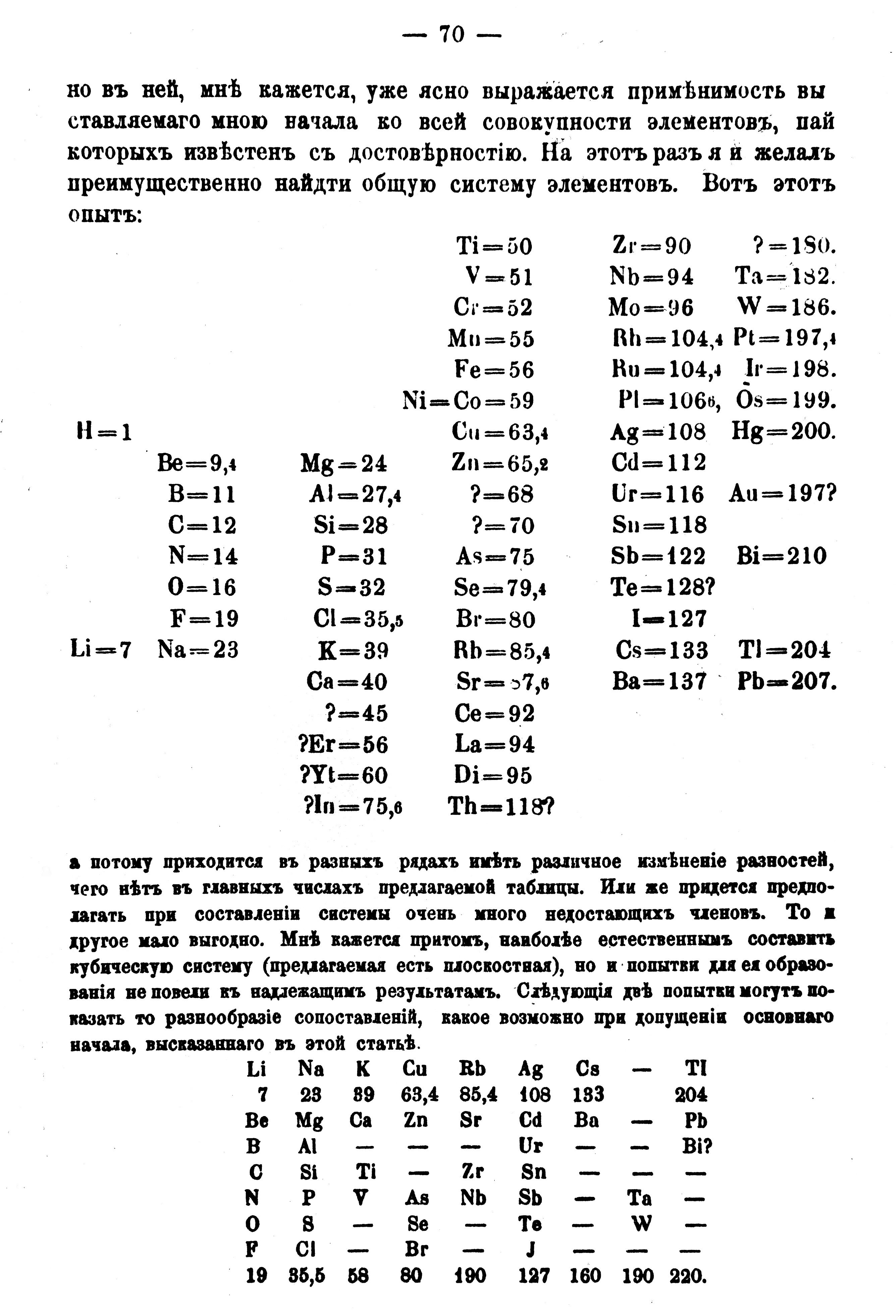
The Russian chemist Dmitri Mendeleev created what scholars widely consider to be the first modern periodic table, published in 1869. Two qualities of this table made it superior to previous attempts. First, Mendeleev did not organize the elements strictly by mass. Instead, he switched the order of some elements on the basis of their chemical properties. Second, he deliberately left gaps in his table where no known elements seemed to fit. Mendeleev correctly predicted that these gaps represented elements that had not yet been discovered. Around the same time, the German chemist Julius Lothar Meyer independently developed a similar periodic table. However, his table was not published until 1870.
In 1913, the English scientist Henry Moseley demonstrated conclusively that the elements in the table should be organized by atomic number rather than atomic mass. Atomic mass generally increases with atomic number. However, this trend does not hold true at several points on the table. Moseley’s work showed that ordering the elements strictly by atomic mass had hindered the development of a proper table. It also justified Mendeleev’s ignoring of mass where it contradicted chemical properties.
In the 1910’s and 1920’s, the development of quantum mechanics, which describes how electrons behave in atoms, provided a physical basis for the peculiar shape of the table. The last major change to the periodic table occurred in the 1940’s with the discovery of the transuranium elements. These elements have atomic numbers higher than that of uranium (atomic number, 92). The American chemist Glenn T. Seaborg proposed placing these elements in the f block rather than classifying them as transition metals. However, elements 104 (rutherfordium) through 112 (copernicium) are generally placed in the d block and classified as transition metals.
Other periodic tables
Many variations of the standard periodic table exist. Some versions list additional information on each element, such as melting point, boiling point, density, state at room temperature, crystal structure, atomic radius, electronegativity, common ionic charges, common uses, or other such properties. These tables serve as handy references for both scientists and students.
Another common version of the periodic table displays the f block between the s block and the d block, rather than removing it to a separate area. This version displays the elements in strict order of atomic number. However, publishers seldom use this version because it is difficult to print legibly on a single page. Other periodic tables list the elements lanthanum and actinium as the first elements in the f block, rather than in group 3 of the d block.
Chemists have also created periodic tables in spiral, triangular, and even three-dimensional shapes. These tables are often designed to show particular properties or relationships not obvious in the standard format of the table.
Expanding the periodic table
The periodic table expands as scientists create new elements in particle accelerators. After IUPAC confirms the discovery of a new element, the element’s discoverers propose a name. IUPAC approves the name along with a two-letter chemical symbol.
Before an element’s discovery can be confirmed and a formal name approved, IUPAC recommends using a provisional name and symbol based on Latin words for the numerals in the element’s atomic number. For example, the provisional name for tennessine (element 117) was ununseptium (chemical symbol, Uus). The letters un come from the Latin word for one, and sept comes from the Latin word for seven. Some periodic tables show such yet unnamed elements along with their predicted chemical and physical properties.
In 2010, IUPAC approved the name copernicium for element 112. The Heavy Ion Research Center in Darmstadt, Germany—which is credited with the discovery—gave it the name, in honor of the Polish astronomer Nicolaus Copernicus. In June 2011, the discoveries of elements 114 and 116 were officially recognized by IUPAC. In 2013, IUPAC approved the names flerovium for element 114 and livermorium for element 116.
In 2015, IUPAC recognized the discoveries of elements 113, 115, 117, and 118, completing the seventh row of the periodic table. In 2016, IUPAC approved the names nihonium for element 113, moscovium for element 115, tennessine for element 117, and oganesson for element 118. Based on position within the table, tennessine can be grouped with the halogens, and oganesson can be grouped with the noble gases. However, these heavy atoms are so unstable that scientists have not been able to determine their chemical properties.