Physics is the science devoted to the study of matter and energy. Physicists try to understand what matter is and why it behaves the way it does. They seek to learn how energy is produced, how it travels from place to place, and how it can be controlled. Physicists are also interested in how matter and energy are related to each other and how they affect each other over time and through space.
The word physics comes from a Greek word meaning natural things. Knowledge obtained from the study of physics is important in other sciences, including astronomy, biology, chemistry, and geology. There is also a close connection between physics and practical developments in engineering, medicine, and technology. For example, engineers design automobiles and airplanes according to certain principles of physics. Laws and theories of physics have enabled engineers and scientists to put satellites into orbit and to receive information from space probes that travel to distant regions of the solar system. Research in physics has led to the use of radioactive materials in the study, diagnosis, and treatment of certain diseases. In addition, theories and principles of physics explain the operation of many modern home conveniences, from vacuum cleaners to videotape recorders.
What physicists study
Physicists try to answer basic questions about the world, how it is put together, and how it changes. Some physicists, called experimental physicists, perform carefully designed experiments and then compare their results to what was predicted to happen. Such predictions come from laws and theories developed by another group of physicists, called theoretical physicists. These laws and theories are almost always expressed in the language of mathematics, a basic tool of physics.
The subjects studied by physicists consist of two broad categories, classical physics and modern physics. These two categories differ primarily in emphasis. Classical physics deals with questions regarding motion and energy. It includes five important areas: (1) mechanics, (2) heat, (3) sound, (4) electricity and magnetism, and (5) light. Modern physics concentrates on scientific beliefs about the basic structure of the material world. Its major fields include (1) atomic, molecular, and electron physics; (2) nuclear physics; (3) particle physics; (4) solid-state physics; and (5) fluid and plasma physics.
Mechanics
is the study of the effects of forces on bodies at rest or in motion. For example, it describes how force acts upon an object to produce acceleration. The mechanics of bodies that undergo a change in motion because of forces acting upon them is called dynamics. The mechanics of bodies at rest or in motion at a constant speed and in a constant direction is called statics. One branch of mechanics, known as fluid mechanics, deals with the behavior of liquids and gases. Principles of mechanics are used in describing such types of motion as planetary orbits and the paths of other moving objects. They are also important to designers of large structures, containers, roads, and various kinds of vehicles. See Dynamics; Mechanics; Statics.
Heat.
The study of heat is called thermodynamics. It involves investigating how heat is produced, how it is transmitted from one place to another, how it changes matter, and how it is stored. Heat energy can be transformed into other kinds of energy, and other kinds of energy can be transformed into heat energy. For example, when coal is burned, the chemical energy that binds its molecules is partially transformed into heat. Thermodynamics also includes cryogenics, the study of material at very low temperatures. Principles of thermodynamics are essential for understanding all types of heat engines, as well as refrigerators and freezers. See Heat; Thermodynamics.
Sound.
The study of sound is called acoustics. Sound consists of vibrations that are produced by an object and which travel through a medium, such as air, water, or the walls of a building. Understanding sound is important for designing auditoriums, hearing aids, tape recorders, phonographs, and speakers. The study of sound also includes ultrasonics, which deals with vibrations that have frequencies too high for human beings to hear. See Acoustics; Sound.
Electricity and magnetism
are so closely related that scientists often refer to the two of them together as electromagnetism. The motion of electric charges can produce magnetic effects, and magnetic forces can produce electrical effects. A combination of these effects produces electromagnetic radiation, which includes visible light, radio waves, and X rays. Knowledge of this relationship has led to the development of huge electric generators and many electronic devices. See Electricity ; Electromagnetism; Electronics; Magnetism.
Light.
The study of light is called optics. Optics has two major branches, physical optics and geometrical optics. In physical optics, physicists study the nature of light and the physical processes by which it is emitted (given off) from bodies and transmitted from place to place. Geometrical optics is the study of how light travels, and how the direction of travel is affected by different materials. It is important in understanding the lenses and mirrors which are used in telescopes, microscopes, and eyeglasses. See Light ; Optics .
Atomic, molecular, and electron physics
are concerned with understanding the structures of molecules and atoms. In particular, these fields of physics concentrate on the behavior, arrangement, motion, and energy states of the electrons that orbit atomic nuclei. Studies in atomic, molecular, and electron physics have revealed much about the structure of matter. For example, scientists have determined that substances differ from one another in the arrangement of the atoms of their molecules. Because of this difference, the way that each substance absorbs and emits electromagnetic energy is unique. As a result, scientists are able to identify a substance on the basis of its electromagnetic activity alone. This method of identification has important applications in medicine and in certain industrial situations where minute amounts of a material are involved. See Atom ; Electron ; Molecule .
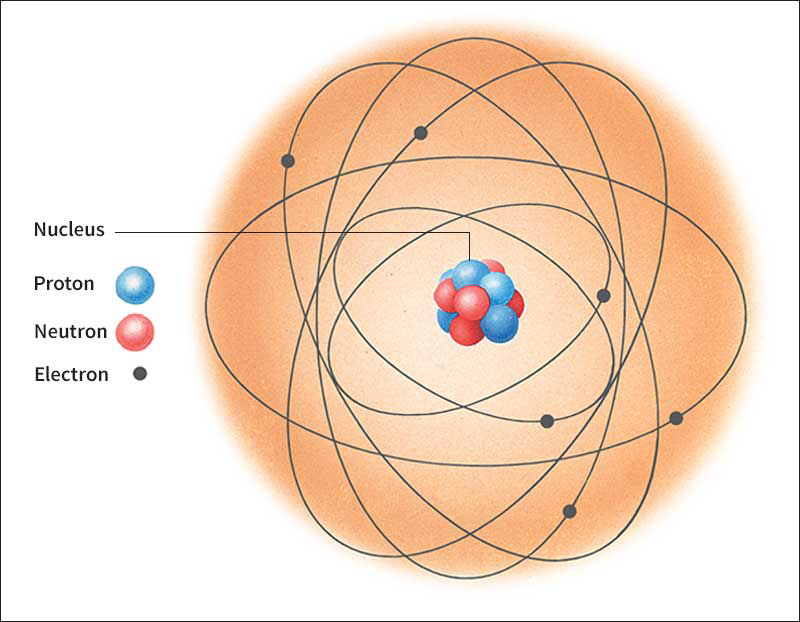
Nuclear physics
involves the study of the structure and properties of the atomic nucleus. It focuses on radioactivity, fission, and fusion. Radioactivity is the process by which certain nuclei spontaneously give off high-energy particles or rays. Radioactive materials are used to treat cancer and diagnose illnesses, and to trace chemical and physical processes. Fission is the process in which an atomic nucleus splits into two nearly equal parts, releasing a huge amount of energy. It provides the energy for atomic bombs and nuclear reactors. Fusion is the process in which the nuclei of two atoms join together to form the nucleus of a heavier element. It occurs primarily with hydrogen and other light elements. Fusion, which releases even more energy than does fission, produces the energy of the sun and other stars. Fusion also powers the hydrogen bomb. See Nuclear physics .
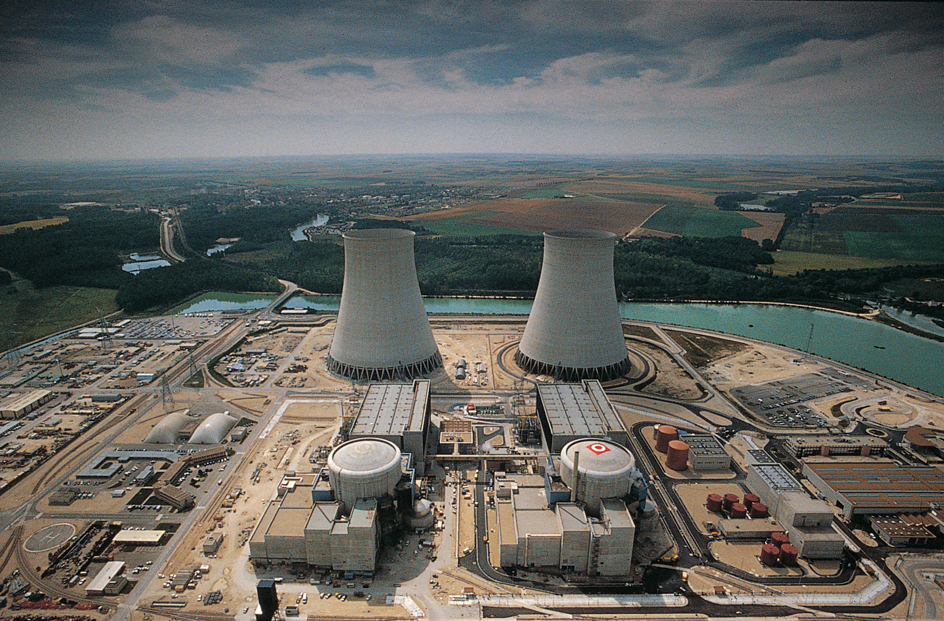
Particle physics.
Physicists have discovered that the protons, neutrons, and other particles within atomic nuclei are formed of still more elementary particles called quarks. Particle physicists conduct research by using particle accelerators. These devices can raise subatomic particles to high speeds. When these particles have reached speeds close to the speed of light, they are allowed to collide with other particles. Physicists study the fragments that result from the collisions and measure their energy. In this way, they hope to understand how protons, neutrons, and other subatomic particles behave. See Particle accelerator .
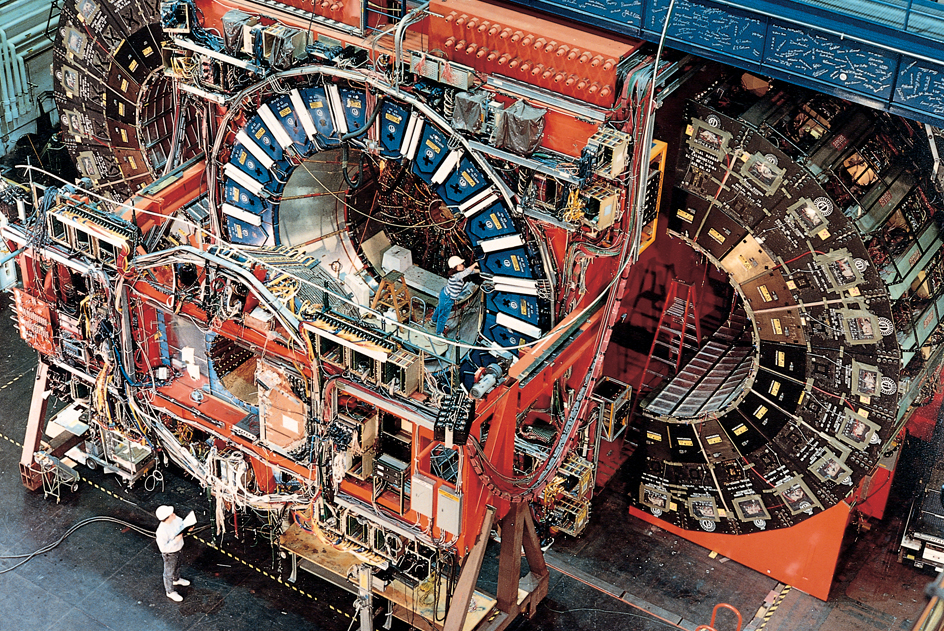
Solid-state physics,
also called condensed-matter physics. Solids may be classified according to how the electrons and nuclei of the different atoms that make them up interact with each other. Physicists who study solids are interested in how the properties of these materials are affected by such factors as temperature and pressure. For example, at extremely low temperatures, some solids lose all electrical resistance, thereby becoming superconductors. Research on the electronic structure of solids is especially important in understanding the behavior of semiconductors, which serve as the basis of modern electronic devices. See Semiconductor ; Solid-state physics ; Superconductivity .
Fluid and plasma physics.
The modern physics of fluids is built on the principles of classical fluid mechanics. Understanding the behavior and movement of fluids is important for the design and construction of automobiles, ships, airplanes, and rockets, as well as for the study of weather. Plasma physics concerns the study of substances called plasmas. When enough energy is introduced into a gas, the gas becomes ionized (separated into positively and negatively charged particles). The result is a plasma. Plasmas are used in neon lights and fluorescent lamps. Physicists are studying how plasmas might be controlled and used to produce fusion energy to generate electric power. See Fluid mechanics ; Hydraulics ; Plasma .
History
Through the centuries, physics has been closely linked to developments in technology and to advances in mathematics, astronomy, and other sciences. The use of the word physics in its current sense was first recorded in the 1700’s.
The beginnings
of physics date back to prehistoric times. Stonehenge and the other huge rock structures prehistoric people built indicate that they had some knowledge of mechanics. Such knowledge would have been necessary for them to transport these rocks and to place them on top of one another. In addition, there is strong evidence that prehistoric people may have used these rock structures to mark significant moments in the seasonal cycle of the sun and moon.
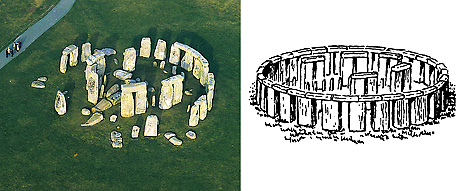
The first people to leave written records of their discoveries and inventions were the ancient Egyptians and Mesopotamians. Mesopotamia is a region in what is now Iraq. By around 3000 B.C., the Mesopotamians had developed a number system, and they used formulas for following and predicting movements of the stars, sun, moon, and planets. Similar developments occurred in Egypt. The Egyptians also developed practical geometric techniques for use in construction and land surveying.
The Greeks
appear to have been the first people to develop general theoretical systems of mathematics and natural science. Beginning about 600 B.C., they developed a general understanding of the principles of geometry. The Greek mathematician Euclid organized these principles into a unified system about 300 B.C.
The Greeks were keen observers of the physical world. In the 300’s B.C., the philosopher Aristotle provided proofs, based on physical evidence, of the spherical shape of Earth. In the 200’s B.C., the astronomer Eratosthenes calculated the circumference of Earth, and Aristarchus, another astronomer, estimated the relative distances to the moon and sun. Also during the 200’s B.C., the mathematician and inventor Archimedes discovered several basic scientific principles and developed ways of calculating areas and volumes.
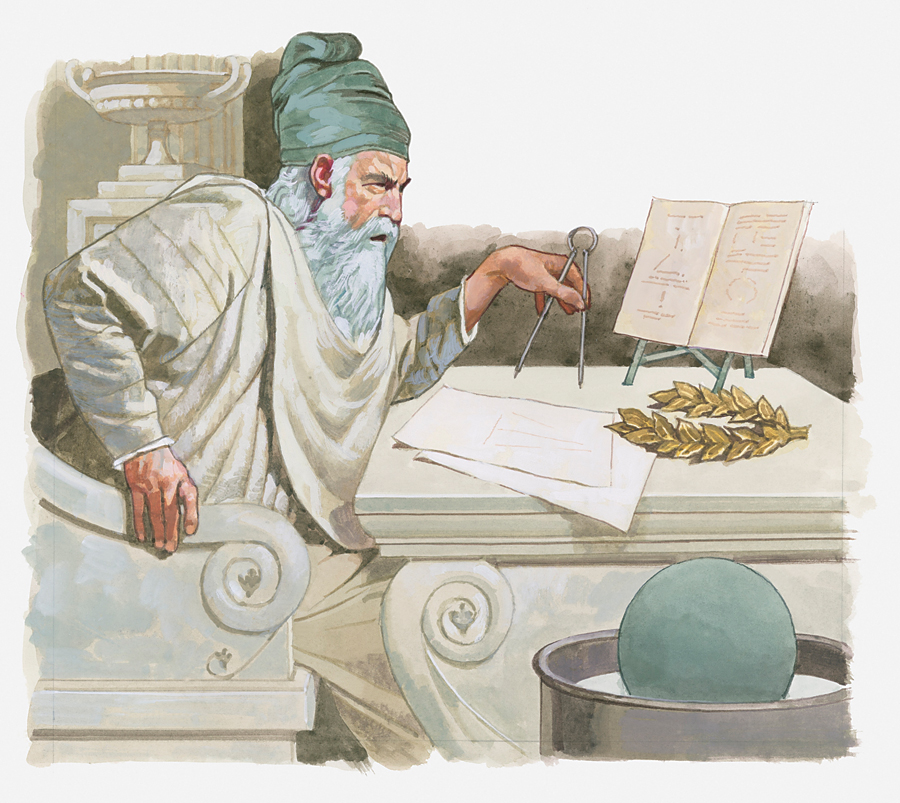
In the A.D. 100’s, Ptolemy, an astronomer in Alexandria, Egypt, developed a model for predicting the positions of the sun, moon, stars, and planets. Like Aristotle and other Greek philosophers, Ptolemy viewed Earth as the center of the universe. Ptolemy’s system served as a guide for predicting the motion of the heavenly bodies for nearly 1,500 years.
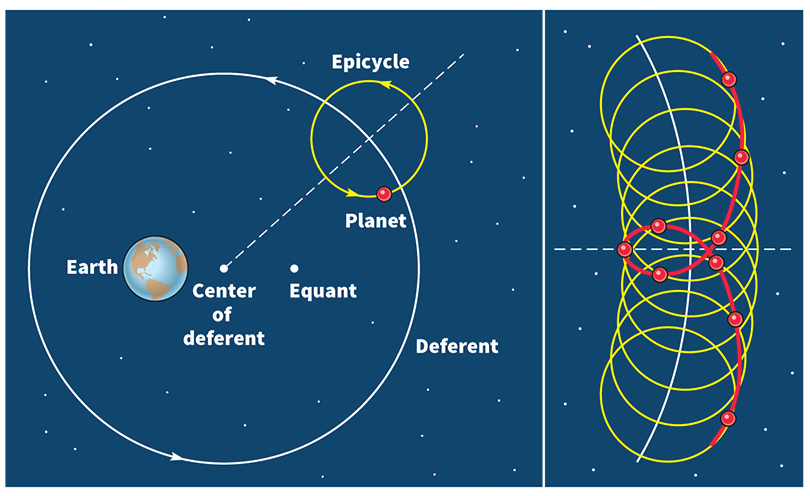
The Middle Ages
began in about the 400’s with the collapse of the Roman Empire in western Europe. At that time, records of Greek scientific discoveries were lost to western Europe. From about 400 to 1000, most educated people in Western Europe felt that religion, rather than scientific investigation, should provide the answers to questions about the universe.
Much of the Greek written tradition in science was preserved during the early Middle Ages by people in the Middle East. These people translated many of the Greek works into Arabic. Arabic scholars wrote commentaries on these texts, made astronomical observations, and performed experiments in optics and mechanics.
Trade between the Arab cultures of the East and the Christian cultures of the West increased during the 1000’s. As a result, Greek scientific documents were reintroduced into the West, this time as translations from Arabic to Latin. At first, the church rejected the science of Aristotle and other Greeks. But during the 1200’s, Saint Albertus Magnus, Saint Thomas Aquinas, and other religious scholars successfully reconciled Aristotelian physical science with church principles.
During the 1100’s and 1200’s, there was also increasing interest in scientific observation and experiments. For example, various writings, including those of the English scholars Robert Grosseteste and Roger Bacon, proposed effective methods for scientific research.
Practical inventions in agriculture and other fields also sparked scientific inquiry in Europe during the Middle Ages. In China and other Asian countries, scientific activity and invention flourished during this period. However, unlike in the West, science and technology had little influence on each other.
The Renaissance
is the name given to the period in Europe that extended from about the early 1300’s to about 1600. It was a time of social, economic, political, and intellectual excitement that produced many new approaches in both the arts and the sciences.
In the 1300’s, at Oxford University and the University of Paris, such scholars as Richard Swineshead and Nicole Oresme investigated the problem of the description of motion. During the 1400’s and 1500’s, the famous Italian painter and inventor Leonardo da Vinci also conducted studies of motion and hydraulics.
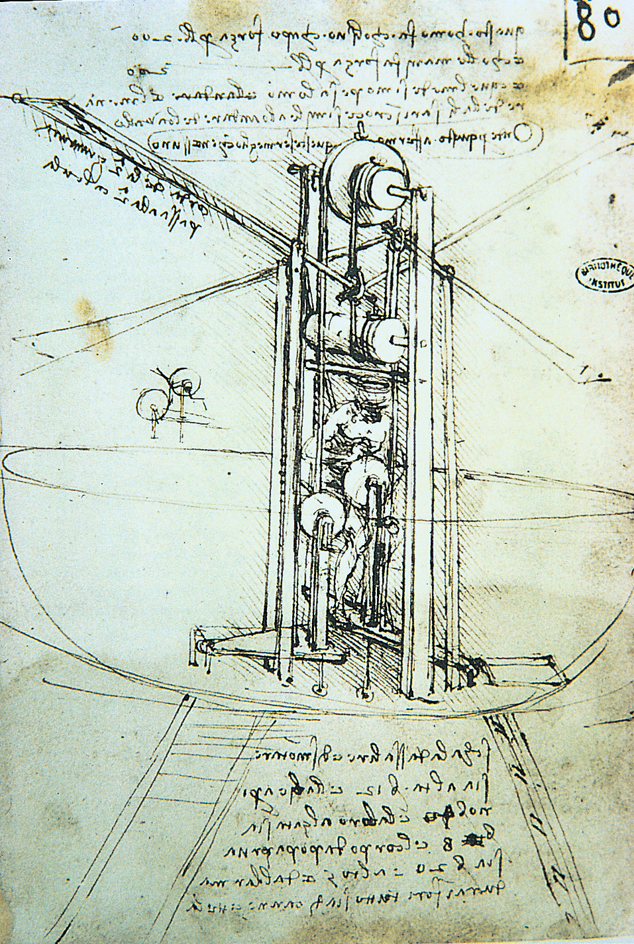
In 1543, the Polish astronomer Nicolaus Copernicus published a revolutionary astronomical system in which he placed the sun—instead of Earth—at the center of the universe. Copernicus proposed that Earth was one of the planets, all of which orbited the sun. At the time, almost no one accepted his point of view. Many Catholic and Protestant leaders felt that his system was in conflict with their religious beliefs. There were also serious scientific objections to the system. Acceptance of the Copernican system required a complete rethinking of the whole basis of physical science. Such a rethinking did in fact occur over the next 150 years, primarily through the work of such major figures as Galileo, Johannes Kepler, and Rene Descartes.
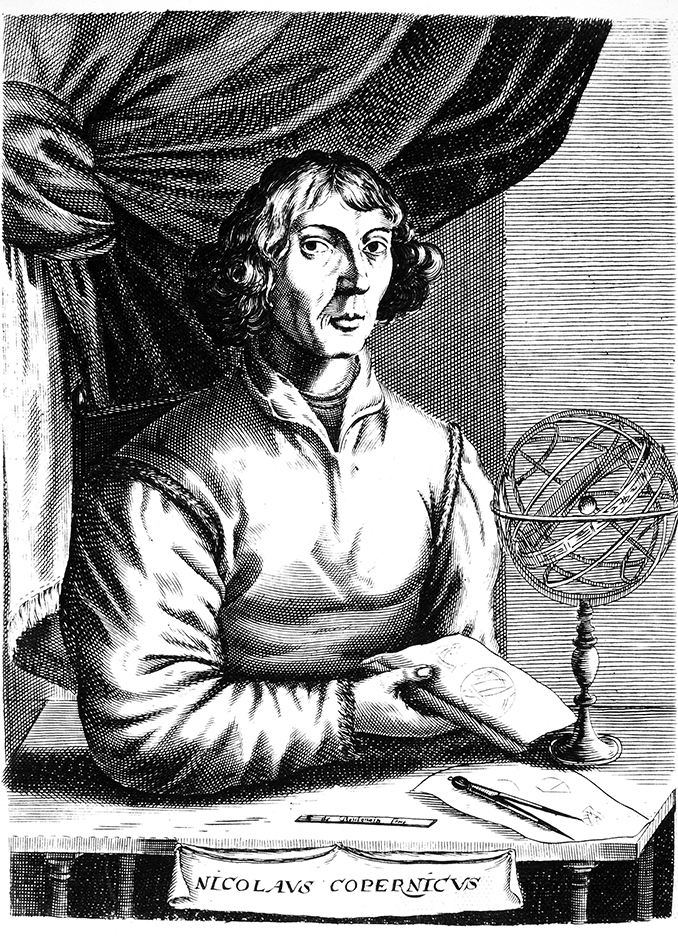
Beginning in 1609, the Italian astronomer and physicist Galileo built a number of telescopes for observing the heavens. While none of Galileo’s observations with his telescopes proved the Copernican system, they did call traditional views into question. Galileo also perfected the idea of the laboratory experiment in his study of the motion of falling bodies. He showed that a person could gain an understanding of the way objects fall by assuming that, in the absence of disturbing influences, all the objects accelerate at the same constant rate.
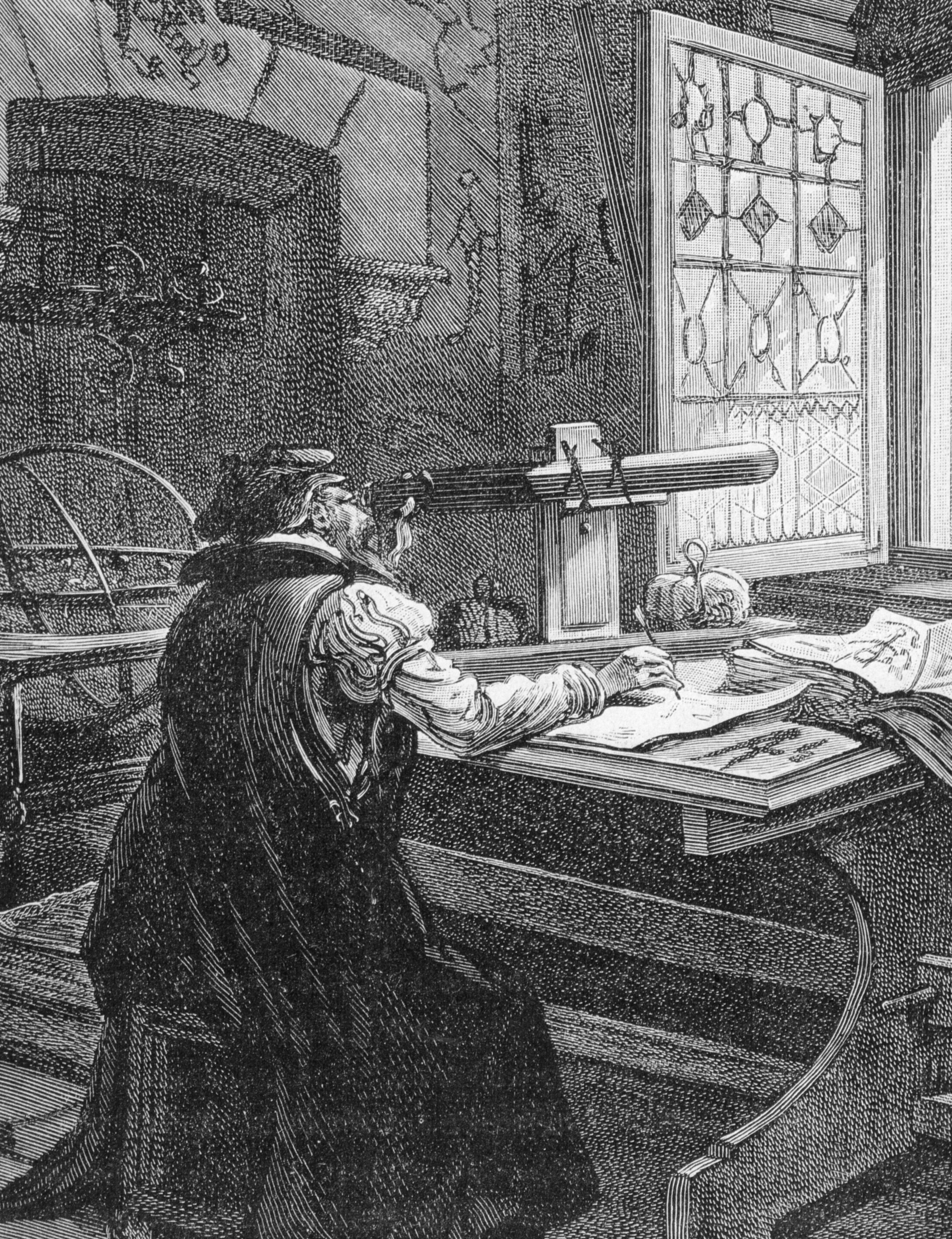
In the early 1600’s, the German astronomer and mathematician Johannes Kepler used the observations of others to construct a new and accurate model of the solar system. In the mid-1600’s, Rene Descartes, a French philosopher and mathematician, challenged the long-standing assumption that an absence of motion was the natural state of all objects. Instead, he proposed that objects have inertia—that is, they maintain whatever their state of motion unless otherwise disturbed.
The work of Galileo, Kepler, and Descartes reflects a change in attitude that occurred during the Renaissance. People had begun to believe that the physical world was governed by natural laws, and that it was possible to discover those laws. The path to such discovery was now seen to begin with careful measurements carried out, if possible, under controlled laboratory conditions.
Newton.
By the 1600’s, a great deal of scientific activity was underway. The climax of this increased activity was the publication, in 1687, of Mathematical Principles of Natural Philosophy, by the brilliant English scientist Sir Isaac Newton. In this work, Newton showed how both the motions of heavenly bodies and the motions of objects on or near the surface of Earth could be explained by four simple laws. These laws were Newton’s three laws of motion and his law of universal gravitation.
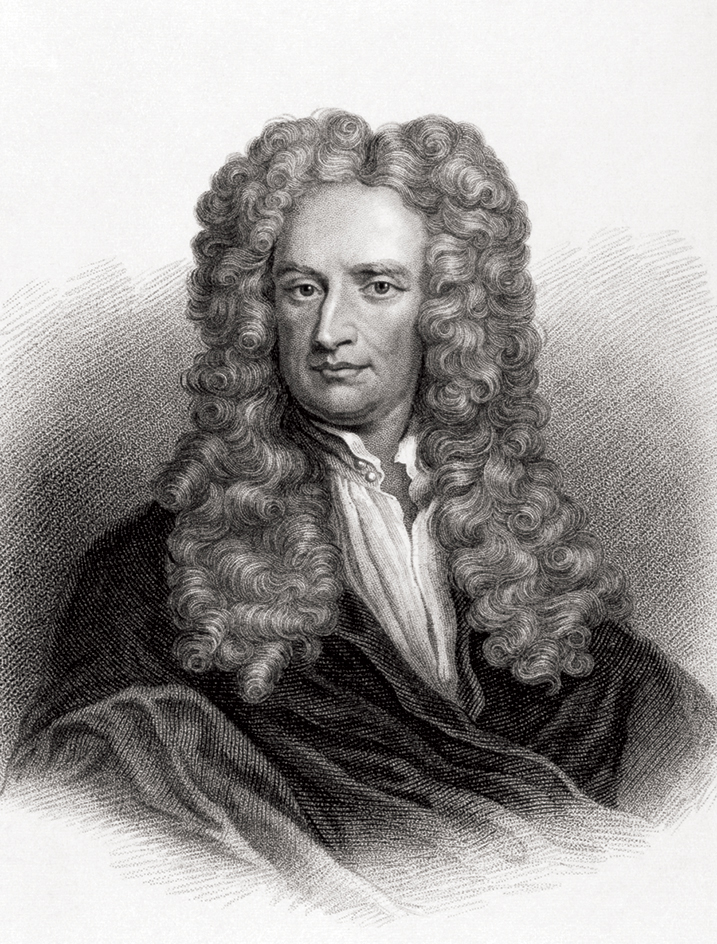
Newton’s laws of motion summarized and extended the work of Galileo and Descartes. His law of universal gravitation explained both Galileo’s law of falling bodies and Kepler’s laws of planetary motion. To perform some of his calculations, Newton invented a new form of mathematics, called calculus. Another scholar, Gottfried Wilhelm Leibniz of Germany, independently developed calculus about the same time. See Calculus .
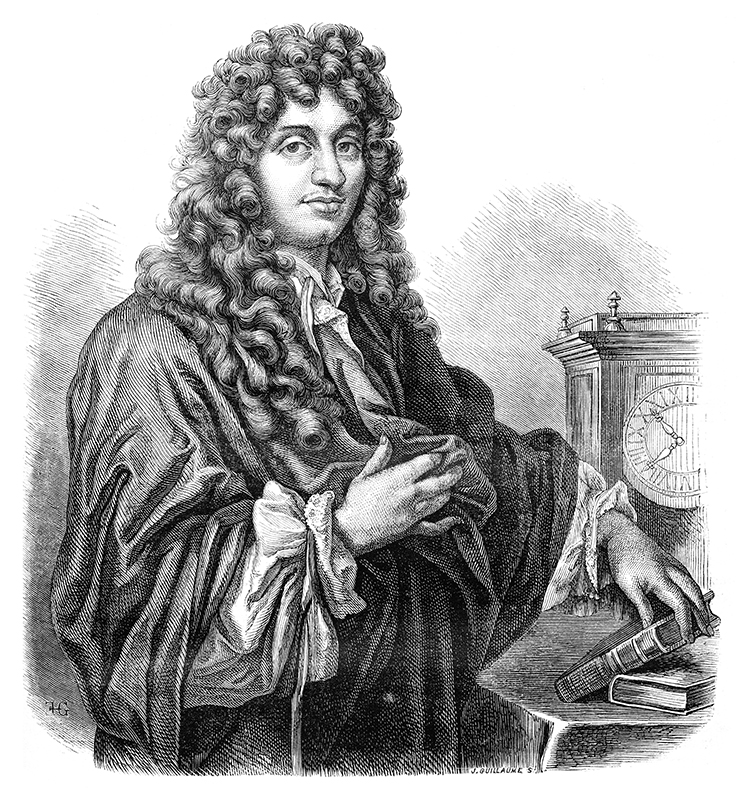
In addition to his theoretical discoveries, Newton constructed the first reflecting telescope. Using prisms, he performed ingenious experiments on light that led him to propose that white light was a mixture of all colors. In 1704, he published a particle theory of light. This theory competed with another theory of light that had been proposed by the Dutch physicist Christiaan Huygens in 1678, but not published until 1690. Huygens had argued that light traveled in the form of waves, rather than particles. But during the 1700’s, most scientists accepted Newton’s particle theory.
Developments in the 1800’s.
The Industrial Revolution, which had begun in the United Kingdom in the 1700’s, led to the production of scientific instruments that were extremely accurate for their time and which enabled scientists to perform more complicated experiments. As scientific research grew more complex, people began specializing in more narrowly defined areas of study. Three areas of particular interest in the 1800’s were heat and energy, light, and electricity and magnetism.
Developments in the study of heat and energy.
At the beginning of the 1800’s, it was widely believed that heat existed in the form of a fluid, called caloric. But by the middle of the century, scientists had come to view heat not as a fluid, but as a form of energy. That is, they had learned that heat is able to do work. In the 1840’s, James Joule, an English physicist, showed how to calculate how much work a given quantity of heat could do. About the same time, a number of physicists, including Lord Kelvin of the United Kingdom and Hermann von Helmholtz of Germany, independently proposed the law of conservation of energy. This law states that energy cannot be created or destroyed, only transformed from one kind to another. See Energy (Conservation of energy) .
By the mid-1800’s, heat energy also came to be interpreted as the mechanical movement of the atoms of which everything was made. This interpretation was based on the atomic theory proposed in 1803 by John Dalton, an English chemist.
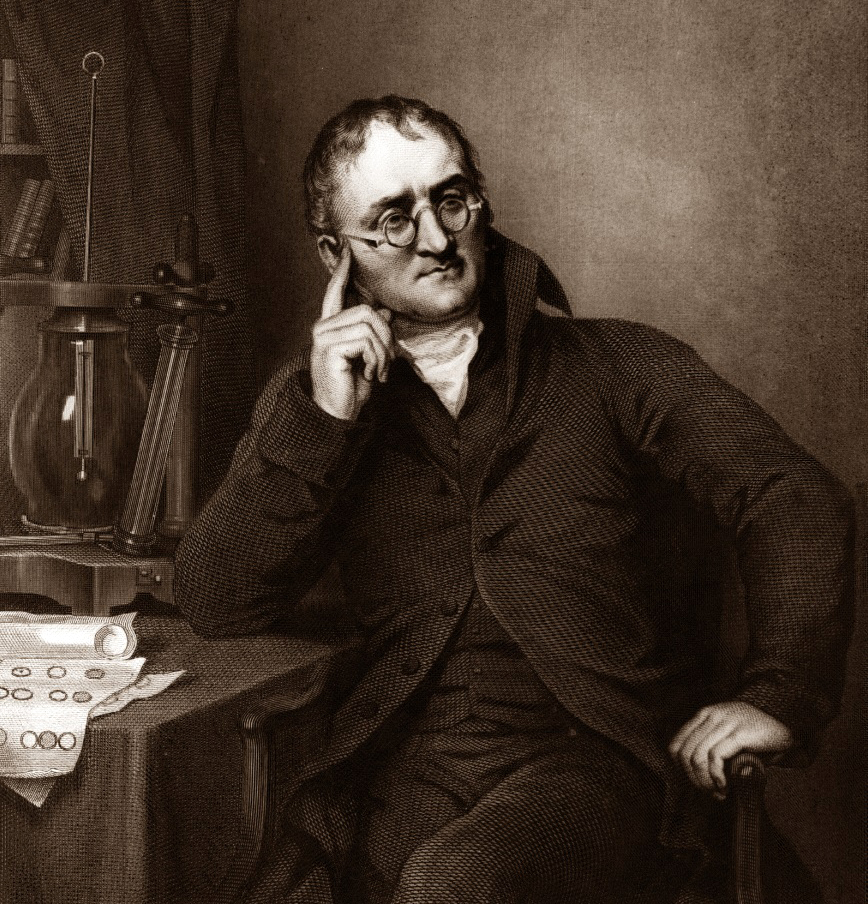
Developments in the study of light.
From 1800 to 1803, the English physicist Thomas Young published a series of papers, based on experiments he had done, that revived the theory that light existed in the form of waves. From about 1815 to 1819, Augustin Fresnel, a French physicist, provided still more evidence. By 1850, the wave theory of light was almost universally accepted, replacing Newton’s particle theory.
The wave theory of light led physicists to propose the existence of a material called the ether. They reasoned that if light traveled in waves and could travel through a vacuum, there had to be some medium present to support the waves. They concluded that all space, including vacuums, was filled with the ether. They interpreted light energy as simply the vibration of the ether, in the form of waves. See Ether .
Developments in the study of electricity and magnetism.
In 1800, Alessandro Volta of Italy announced his invention of the first electric battery. This invention opened the way for new methods of studying electrical effects. About 1820, two physicists, André-Marie Ampère of France and Hans Christian Oersted of Denmark, showed that electricity and magnetism were related. In the early 1830’s, the English physicist Michael Faraday and the American physicist Joseph Henry independently demonstrated how to produce electric current in a changing magnetic field. Their demonstrations showed that mechanical energy could be converted into electrical energy and suggested the principles behind the generator and the motor.
In the 1860’s, the Scottish physicist and mathematician James Clerk Maxwell developed a theory that interpreted visible light as the movement of electromagnetic waves. Maxwell predicted the possible existence of similar electromagnetic waves that were invisible. Heinrich Hertz, a German physicist, detected such invisible radio waves in the late 1880’s. Hertz’s discovery eventually led to the development of radio, radar, and television. But it also suggested that light, electricity, and magnetism were related. All three were viewed as resulting from waves in the ether. Such waves are sometimes referred to as electromagnetic radiation.
The beginning of modern physics.
Near the end of the 1800’s, many physicists believed that the work of physics was nearly over. They believed that science had discovered almost all the laws governing the physical universe. Some of them believed that all physical laws would one day be expressed in a few simple equations.
A few problems remained to be solved, however. One such problem involved determining the source of electromagnetic radiation. Scientists knew that under the right conditions, each chemical element radiates a unique combination of visible, infrared, and ultraviolet light, called line spectra. At the time, the atom was considered to be the most fundamental unit of matter in the universe. But to some physicists, the line spectra phenomenon suggested that the atom might itself be composed of still more fundamental units.
The dream of explaining all physical phenomena with one small set of basic laws was not realized. Instead, various discoveries began to reveal that such phenomena were more complex than scientists had thought. In 1895, for example, Wilhelm Roentgen of Germany discovered X rays. In 1896, the French physicist Antoine Henri Becquerel discovered natural radioactivity, the spontaneous emission of radiation from atoms. In 1897, the English physicist Joseph John Thomson discovered the first subatomic particle, later called the electron. In 1898, the French physicists Marie Curie and her husband, Pierre, discovered the radioactive element radium. Such developments signaled that, rather than being nearly over, the work of physics had only begun.
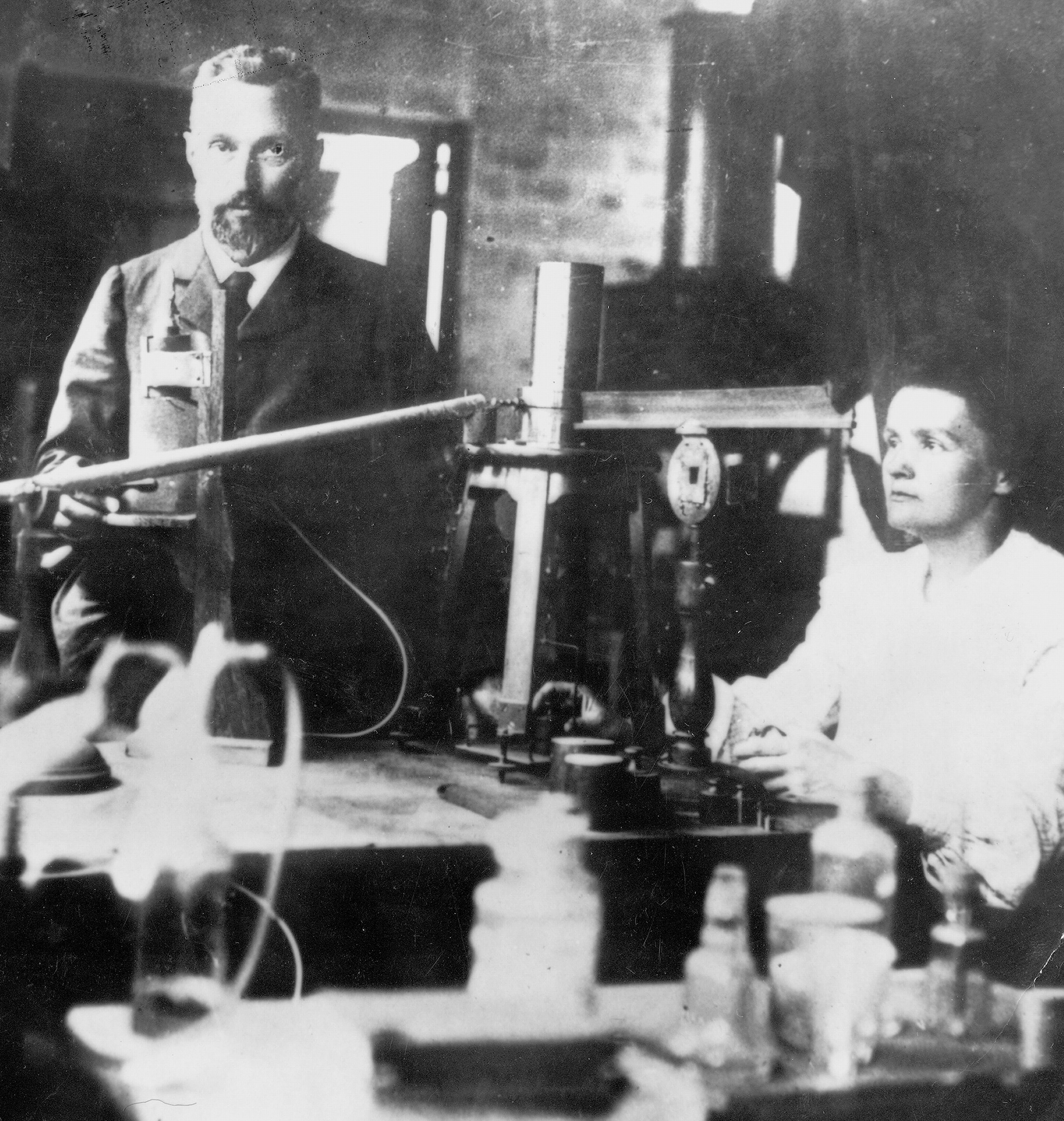
Quantum theory.
The 1900’s brought revolutionary developments in physics. Scientists looked for errors in the classical physics of Newton and others, and discovered new interpretations of observed events.
In 1900, the German physicist Max Planck published his quantum theory of energy transfer to explain the spectrum of light emitted by certain heated objects. He stated that energy is not given off continuously, but in the form of individual units called quanta. In 1905, Albert Einstein, the German-born American physicist, proposed a new particle, later called the photon, as the carrier of electromagnetic energy. Einstein said that light, in spite of its wave nature, must be composed of these energy particles.
In 1913, the Danish physicist Niels Bohr explained in terms of quanta how atoms absorb and radiate energy. In 1924, Louis de Broglie, a French physicist, proposed that electrons could also exhibit wave properties. In the mid-1920’s, two physicists, Erwin Schrödinger of Austria and Werner Heisenberg of Germany, produced separate, but equivalent, systems for organizing all earlier quantum physics. The combined ideas of Schrödinger and Heisenberg have since been developed as the field of quantum mechanics. See Quantum mechanics .
Einstein and relativity.
During the 1800’s, physicists tried unsuccessfully to measure the speed of Earth relative to the ether. According to classical physics, the ether was motionless. In the early 1880’s, Hendrik A. Lorentz, a Dutch physicist, explained the failure of these experiments by assuming that the ether was partially dragged along as Earth moved through it. Two American physicists, Albert A. Michelson and Edward W. Morley, developed an instrument that made far more precise measurements than earlier devices. Their experiments helped destroy the ether theory. In 1887, Michelson and Morley demonstrated that Earth’s movement around the sun had no effect on the speed of light. Their finding could be understood only by assuming that the ether near the surface of Earth moved at the same speed as Earth. However, this assumption contradicted the results of many other experiments.
The contradiction was not resolved until 1905. That year, Albert Einstein analyzed the measuring process itself and, as a result, proposed his special theory of relativity. The theory begins with two postulates (fundamental principles). The first postulate states that for all observers moving uniformly relative to each other, the laws of physics have the same form. The second postulate states that for all observers, the speed of light is invariant (has the same value). One conclusion from these postulates is that mass and energy are related. Einstein expressed this relationship in his famous equation E = mc-squared (also written E= mc 2). In this equation, E stands for energy, m for mass, and c-squared for the speed of light multiplied by itself.
Einstein also attempted to replace classical gravitational theories with a more exact statement of the laws of gravitation. In 1915, he announced his general theory of relativity, which assumes that the effects of gravity on objects are identical to the effects of nongravitational forces acting on objects. Gravity is no longer viewed as a property of objects interacting with each other, but of objects interacting with space itself. The theory predicted that the path of a light beam will be affected by nearby massive objects. This prediction was confirmed in 1919. The theory also predicted the existence of gravitational waves that travel at the speed of light. This prediction was confirmed in 2016, when researchers announced that the Laser Interferometer Gravitational-Wave Observatory (LIGO) had detected gravitational waves created by the collision of two black holes.
In 2011, scientists announced that data gathered by the National Aeronautics and Space Administration’s Gravity Probe-B confirmed two effects predicted by the general theory: These effects were the geodetic effect, the warping of space and time around a gravitational body, and frame-dragging, the amount a spinning object pulls space and time with it as it rotates. See Relativity .
Uncovering the secrets of the atom.
The discovery that atoms have an internal structure prompted physicists to probe further into these tiny units of matter. In England, Ernest Rutherford developed a model of the atom in 1911. In this model, the dense positive charge resided in a small, spherical core called the nucleus, and the electrons traveled around this nucleus. Bohr proposed modifications of the model in 1913. That year, an American, Robert Millikan, reported an accurate measurement of the electron’s charge. See Atom .
Loading the player...Atomic transmutation
The discovery of other subatomic particles continued after this early work. In 1932, James Chadwick, an English physicist, conducted experiments that suggested that the atomic nucleus was composed of two kinds of particles: positively charged protons and neutral neutrons. In 1935, Hideki Yukawa, a Japanese physicist, proposed that other particles, which he called mesons, exist in the atomic nucleus (see Meson ).
In 1938, two German physicists, Otto Hahn and Fritz Strassmann, discovered nuclear fission by splitting uranium atoms. Scientists quickly realized that, as Einstein’s E = mc2 formula indicated, fission could liberate huge quantities of energy. In 1942, during World War II, the Italian-born physicist Enrico Fermi and his co-workers at the University of Chicago achieved the first controlled fission chain reaction. In 1945, near the end of the war, American scientists and engineers produced the first bombs that relied on nuclear fission for their explosive power. Two such atomic bombs were dropped on Japan in August 1945. See Nuclear energy (The development of nuclear energy) ; Nuclear weapon .
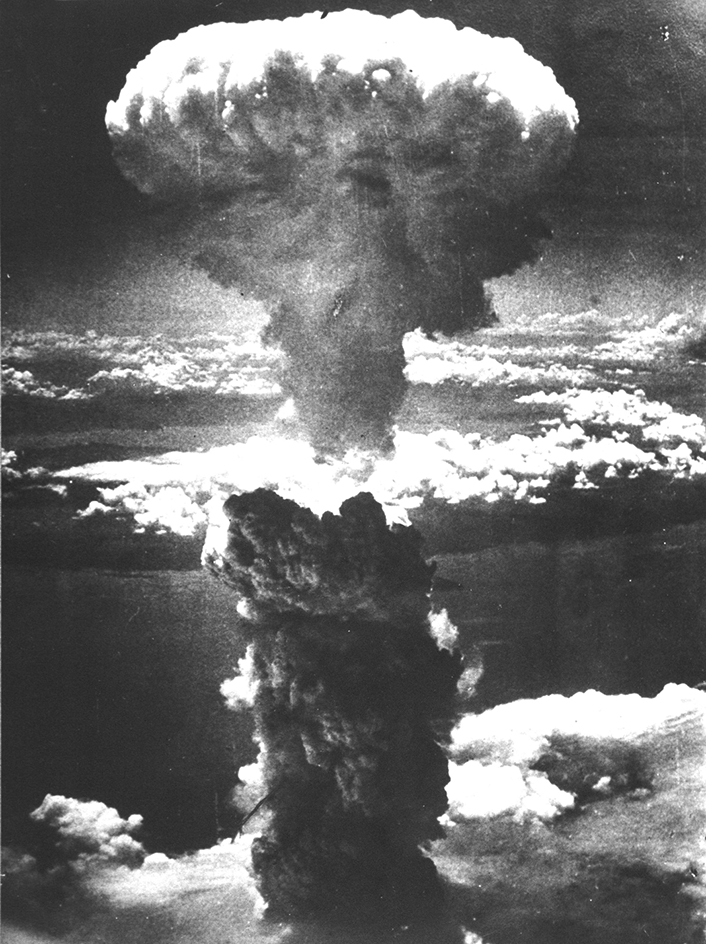
Advances in the mid-1900’s.
After 1945, the picture of the atom grew more complicated as physicists discovered more and more subatomic particles. In 1955, the American physicists Owen Chamberlain and Emilio Segre discovered the antiproton (a negatively charged proton) In 1964, two California Institute of Technology physicists, the American Murray Gell-Mann and Russian-born George Zweig, proposed the existence of quarks as fundamental particles. Protons and neutrons are composed of different combinations of quarks. Strong evidence for the existence of the quark resulted from the discovery of the psi particle, a type of subatomic particle also called the J particle, by the Americans Burton Richter and Samuel C. C. Ting. By 1995, scientists had discovered six types of quarks. Physicists are almost certain that there are no more quarks to discover. See Antimatter ; Psi particle ; Quark .
Research in the mid-1900’s also led to important advances in technology. In 1947, American physicists invented the transistor. This tiny device revolutionized the electronics industry. In the early 1960’s, researchers in atomic and optical physics produced light-amplifying devices called lasers. Lasers have become valuable tools in such areas as communications, industry, and nuclear energy research. See Laser ; Transistor .
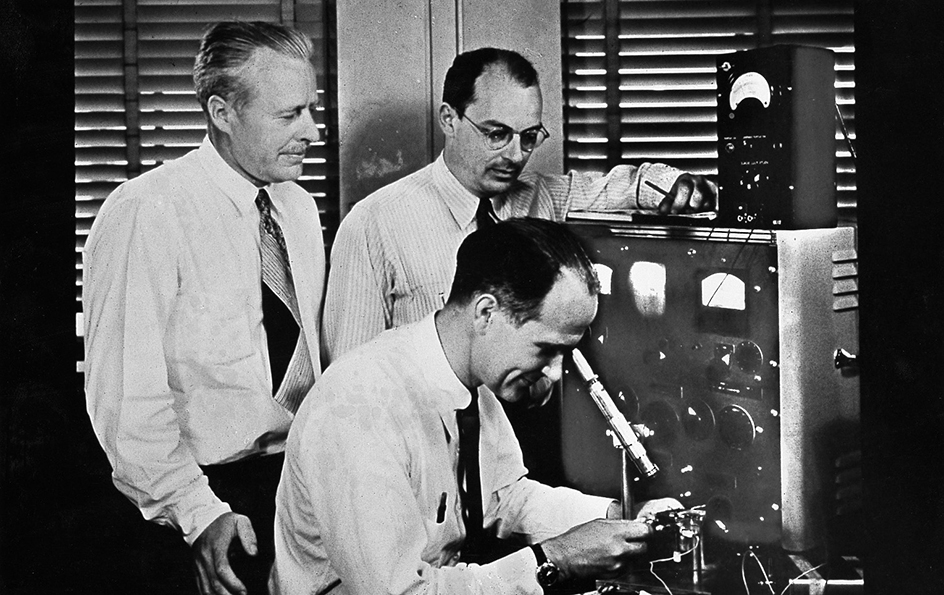
Physics today.
Physics continues to be one of the most active and important sciences. Ongoing research into the nature of matter has led to important discoveries. For example, researchers in West Germany discovered an important elementary particle, the gluon, in 1979. Gluons carry the strong nuclear force. This force, also called the strong interaction, holds quarks together in protons, neutrons, and other particles; and holds protons and neutrons together in atomic nuclei. In 1983, a research team led by Carlo Rubbia of Italy discovered two more subatomic particles—the W particle and the Z particle. Physicists predict that these particles are a source of the weak nuclear force, or the weak interaction. This force controls the disintegration of atomic nuclei—the process at work in radioactivity.
Physicists believe that six kinds of quarks exist. The last of these to be discovered was the top quark. Scientists at Fermi National Accelerator Laboratory (Fermilab) in Batavia, Illinois, announced the discovery of this particle in 1995 Physicists also believe that there are three types of neutrinos, particles that interact with other particles by means of the weak nuclear interaction. The last kind of neutrino to be directly detected is known as the tau-neutrino. In 2000, researchers at Fermilab announced that they had made the first direct detection of tau-neutrinos.
There may be an underlying unity among three of the basic forces of the universe: the strong force, the weak force, and the electromagnetic force that holds electrons to the nucleus. Theories that attempt to establish this underlying unity are referred to as grand unified theories (GUT’s). Researchers are also testing supergravity theories, which would include the fourth fundamental force, gravitation. Such theories illustrate how physicists have again begun to express the hope that a few basic laws will unify all our knowledge about how the world works.
Physics also continues to make important contributions to technology. For example, advances in electronics resulted in the development of extremely sophisticated computers. Lasers and optical fibers (glass or plastic filaments that carry light) led to improvements in communication systems and medical technology (see Fiber optics ). Physicists began developing ceramiclike materials that can act as superconductors at much higher temperatures than the superconductors of the past.
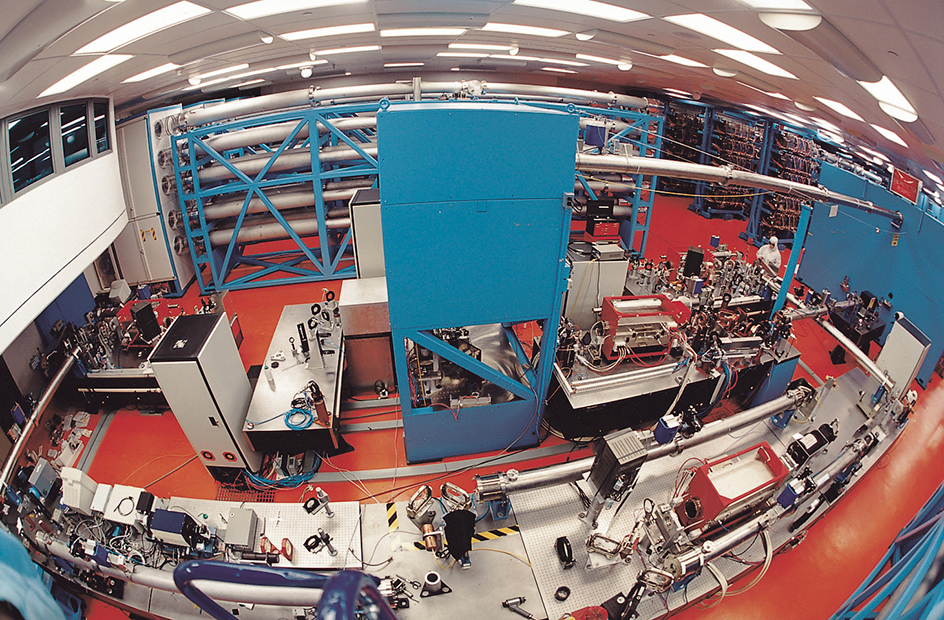
Careers in physics
Education and training.
A person who plans to pursue physics as a career should take science and mathematics courses in high school. The formal training of physicists begins in college. Physics students must learn calculus, modern algebra, and other forms of higher mathematics. They also take basic courses in chemistry. After a year or two of introductory courses, physics majors begin to specialize, taking more advanced courses in the various subfields of physics. Many of these advanced courses include intensive laboratory work.
Most physics majors continue their education beyond the bachelor’s degree. A majority pursue doctor’s degrees.
Employment.
More than half the physicists in the United States are engaged in research and development activities. Many industries employ physicists in their research departments. These people often work in applied physics, which generally involves improving manufacturing processes or products. Many physicists are employed by colleges and universities. They generally divide their time between teaching classes and performing research.
Today, physics research often requires the use of highly specialized and expensive instruments and equipment, such as high-energy particle accelerators. In the United States, special laboratories with this type of equipment are funded by government agencies (see National laboratory ). An association of European nations now known as the European Organization for Nuclear Research (CERN), established the world’s largest particle physics research center, near Geneva, Switzerland. Although the U.S. national laboratories and the CERN laboratories have their own staffs, physicists throughout the world may apply to use the facilities in their research.