Quantum << KWON tuhm >> mechanics is a branch of physics that describes the structure and behavior of matter. It has replaced classical mechanics, a group of older theories, as a description of the smallest known units of matter and their activity. The older theories are still adequate for describing and predicting the behavior of objects that we ordinarily encounter in our daily lives.
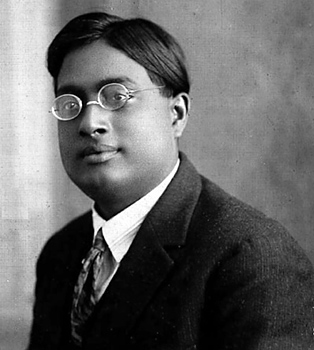
Classical mechanics is based on physical laws described by two physicists and mathematicians, Isaac Newton of England and James Clerk Maxwell of Scotland. Newton explained his laws of motion and gravitation in Philosophiae naturalis principia mathematica (Mathematical Principles of Natural Philosophy), published in 1687. In 1864, Maxwell described electric and magnetic phenomena in a series of equations. Several scientists helped establish quantum mechanics from 1900 to the late 1920’s.
Quantum mechanics describes a number of objects and phenomena that seem strange and are difficult to understand. Among these are quanta, or “chunks” of energy; the wave-particle duality of matter; and the uncertainty principle, which limits what we can know about objects.
Quanta of energy.
Before quantum mechanics was developed, physicists considered atoms to be made up of solid particles. In addition, scientists had found that light has properties of waves. For example, they had observed that light can spread around an obstacle.
Scientists knew that visible light is a form of electromagnetic radiation, and they understood its relationship to the other forms of such radiation. From the least energetic to the most energetic form, electromagnetic waves consist of radio waves, microwaves, infrared rays, visible light, ultraviolet rays, X rays, and gamma rays. Physicists also understood the relationship between the energy of electromagnetic waves and their wavelength (distance between successive wave crests). The greater the energy of a wave, the shorter its wavelength.
Physicists commonly refer to all kinds of electromagnetic radiation as light, except when it is important to mention a specific kind of radiation. The remainder of this article follows that practice.
Emission of quanta.
The German physicist Max Planck found the first failure of classical mechanics in 1900. Scientists had measured the amounts of energy in different wavelengths of light emitted (sent out) from the surfaces of certain hot objects. According to classical mechanics, the tiny vibrating objects that emit light from the surfaces, now known as molecules and atoms, can emit continuous amounts of energy. But Planck showed that the measured emissions cannot be explained if the energy is emitted continuously. He said that, instead, the energy is emitted in “chunks,” which came to be called quanta. Quantum is the singular of quanta.
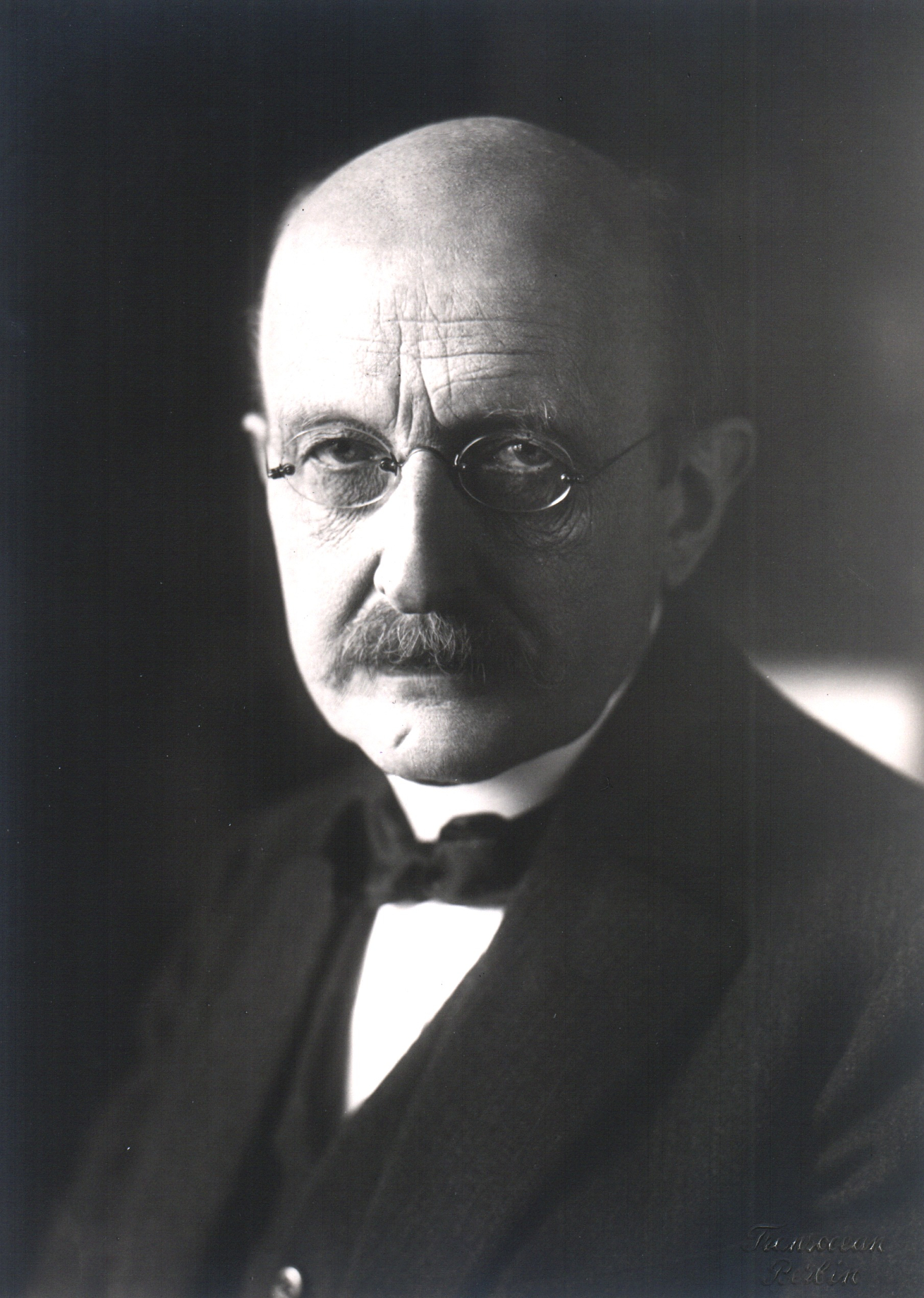
Quantum nature of light.
The German-born physicist Albert Einstein extended Planck’s ideas in a series of scientific papers beginning in 1905. One of Einstein’s proposals was that all light consists of quanta—wave “packets” that were later called photons.
Quantized orbits.
By 1911, scientists knew that an atom is made up of a positively charged nucleus surrounded by negatively charged electrons. But positively charged objects attract negatively charged objects. Scientists could not explain why electrical attraction does not make the electrons spiral into the nucleus.
In 1913, the Danish physicist Niels Bohr offered an explanation. He said that electrons in atoms can change their energy only by absorbing or emitting quanta. According to Bohr’s explanation, electrons can occupy only certain orbits around the nucleus. When an electron absorbs a quantum, the electron moves quickly to an orbit farther from the nucleus. When an electron emits a quantum, the electron jumps to a closer orbit. But when an electron reaches its closest possible orbit, it cannot plunge into the nucleus.
Wave-particle duality
refers to the fact that light and parts of atoms have properties of both particles and waves. Experiments conducted in 1923 by American physicist Arthur H. Compton showed that photons have a property of particles called momentum—that is, a total amount of motion. For slowly moving particles, momentum equals velocity (speed in a given direction) times mass (amount of matter). Photons have zero mass, but still possess momentum.
In 1924, Louis de Broglie, a French physicist, proposed that subatomic particles, such as electrons, are associated with waves. Experiments conducted later showed that he was correct. As he suggested, electrons can bend around obstacles, and they can display wave shapes known as interference patterns and diffraction patterns.
Quantum mechanics now describes an electron as a wave—but a wave with an unusual interpretation. The wave serves to locate the electron, but the electron must always be measured as a whole particle. Specifically, the square of the height of the wave at a given point in space corresponds to the probability that the electron is at that point. (The square of the height equals the height multiplied by itself.)
The uncertainty principle.
In 1927, the German physicist Werner Heisenberg discovered a general characteristic of quantum mechanics, the uncertainty principle. According to this principle, it is impossible to precisely describe both the location and the momentum of a particle at the same time. For example, if you describe a particle’s location with much precision, you must give its momentum in terms of a broad range of numbers.
The uncertainty principle affects attempts to measure a particle’s location and momentum. Suppose you want to measure the position of an electron precisely. To do so, you force the electron to absorb and then re-emit a photon so that a light detector can “see” the electron. You know the precise location of both the photon source and the light detector. But even so, the photon’s momentum spoils your attempt: The absorption of a photon by the electron changes the electron’s momentum. That is, the photon “bumps the electron off course.” The electron is therefore proceeding in a new direction when it re-emits the photon. Thus, your detection of the re-emitted photon cannot enable you to determine where the electron was when it absorbed the initial photon.