Radar is an electronic instrument used to detect and locate moving or fixed objects. Radar can determine the direction, distance, height, and speed of objects that are hidden from view by clouds or are much too far away for the human eye to see. It can find objects as small as insects or as large as mountains. Radar can even operate effectively at night and in heavy fog, rain, or snow.
Radar serves a wide variety of purposes. In bad weather, ship navigators use it to steer their ships clear of nearby vessels and dangerous objects. Many countries use radar to guard against surprise attacks from enemy aircraft or missiles. Radar enables weather forecasters to track approaching storms. Scientists use radar to investigate the atmosphere and surface of Earth and also to study the other planets and their moons.
The word radar comes from ra_dio _d_etection _a_nd _r_anging. Almost every radar set works by sending radio waves toward an object and receiving the waves that are reflected from the object. The time it takes for the reflected waves to return indicates the object’s _range—how far away it is. The direction from which the reflected waves return tells the object’s location.
Radar sets vary greatly in size and shape, but they all have the same basic parts. Every set has a transmitter to produce radar waves and an antenna to focus them and send them out. In most types of radar, the same antenna collects the waves bounced back from an object. The reflected waves, commonly called echoes, are strengthened by a receiver so they can be seen on a display. The typical radar display resembles the picture tube of a television set. It shows the echoes as spots of light or as a color image of the object observed.
The uses of radar
In aviation.
Radar contributed greatly to aviation safety both at airports and in airplanes. Air traffic near large airports is extremely heavy. Air traffic controllers at major airports used radar to direct the continuous flow of incoming and outgoing planes. Radar enabled them to prevent collisions by selecting the safest routes for pilots to follow. Radar also enabled controllers to direct landings from the ground when bad weather made approach lights and runways difficult for pilots to see.
Most modern aircraft now have technology called Automatic Dependent Surveillance-Broadcast (ADS-B). ADS-B uses signals from Global Positioning System (GPS) satellites to safely communicate with air traffic controllers and other airplanes.
In ship navigation.
Radar is widely used as a navigation aid on all kinds of boats and ships, from small pleasure craft to huge oil tankers. When visibility is poor, a ship’s radar can spot other vessels, reefs, and icebergs in time to prevent an accident. It is especially useful in fog. When a ship is near shore, the navigator can determine the vessel’s position by the radar echoes from special reflector buoys, islands, and other landmarks.
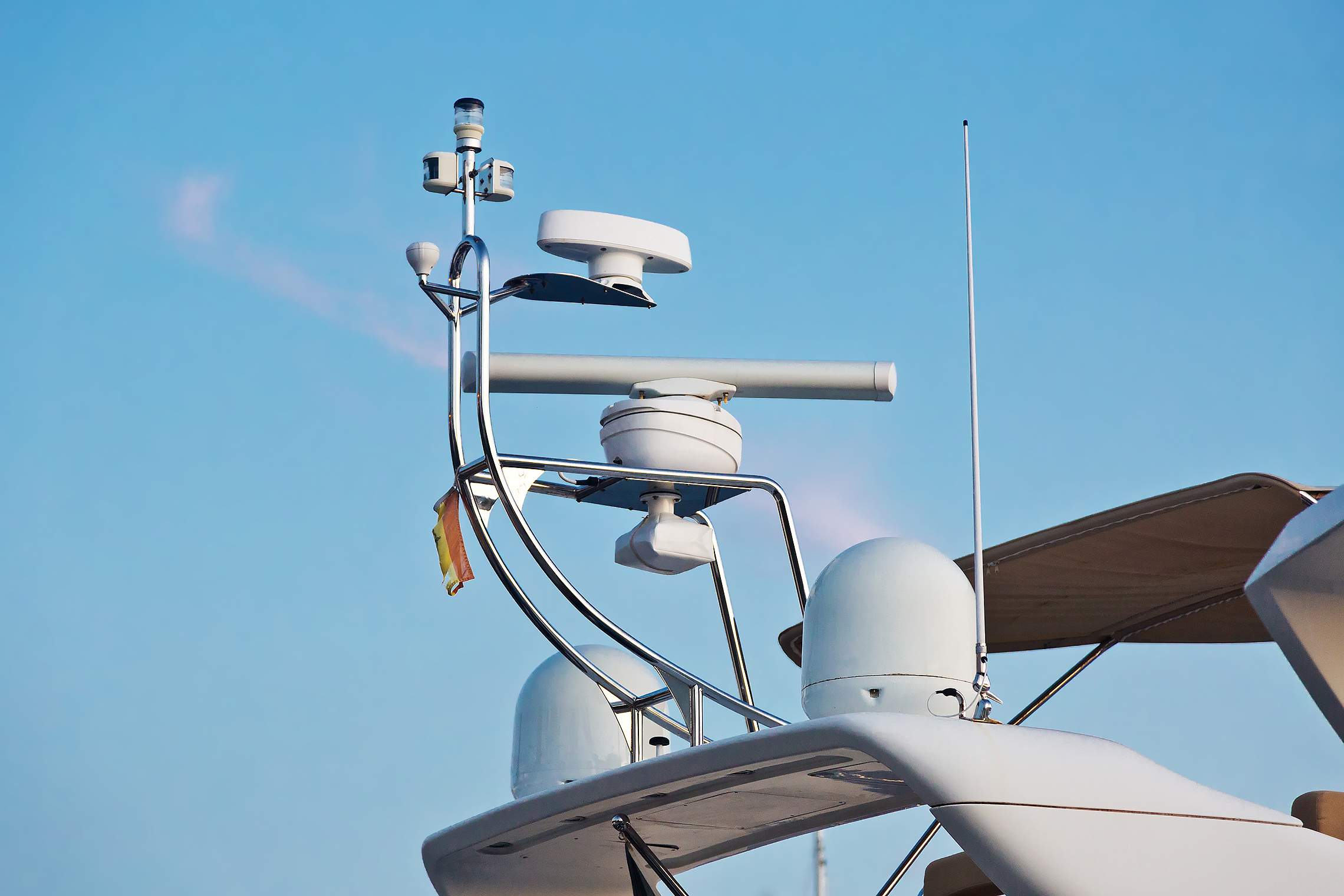
Harbor masters use radar to control ship traffic in crowded seaports. They follow the movements of all ships in a harbor on a radar display that provides a maplike picture of the harbor. By means of radio communication, harbor masters can guide ships into and out of a port safely in any weather.
Coast guard stations keep track of vessels in their vicinity through radar observations. Coast guards also use radar to search for ships that are reported missing.
In the military.
Radar has a variety of military uses. The major uses include (1) air defense, (2) missile defense, (3) space surveillance, (4) intelligence gathering, (5) range instrumentation, and (6) weapon fire control.
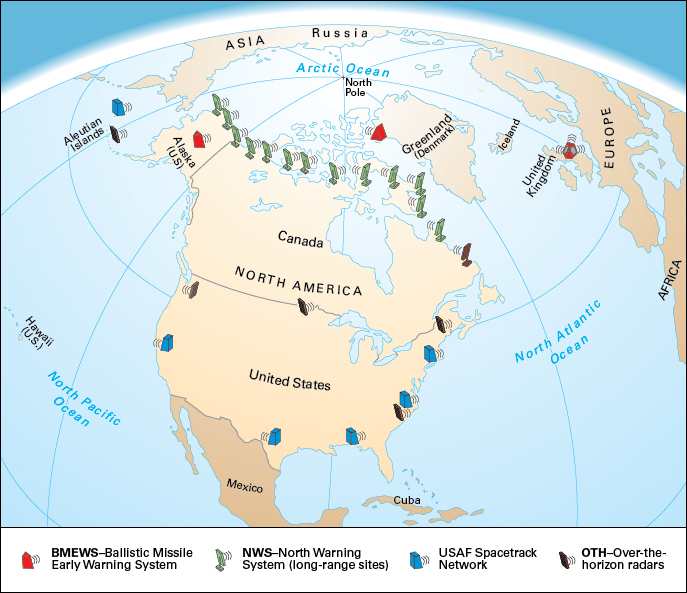
Air defense
requires long-range radar that can detect and track approaching enemy aircraft at great distances and so give the earliest possible warning. Vast networks of radar stations form the heart of most nations’ air defense systems. The North Warning System, a network of radar stations across northern North America, is designed to alert the United States and Canada to an airborne attack from the north. Australia, China, Russia, and the United States also have built huge over-the-horizon radar stations to detect attacks from any direction.
In addition to land radar stations, Israel, the United Kingdom, the United States, and several other countries use aircraft equipped with radar for protection against surprise air attacks. Airborne radar can spot low-flying enemy bombers that may escape detection by ground-based radar.
Missile defense
consists of radar networks like those used for early warning of hostile aircraft. But more powerful radar is needed to detect guided missiles because they fly faster and much higher than planes. For example, the main radar network developed by the United States for missile defense is the Ballistic Missile Early Warning System (BMEWS). This system has installations in Alaska, Greenland, and England. Its radar units can spot long-range missiles up to 3,000 miles (4,800 kilometers) away. Another major U.S. radar network operates under the Sea-Launched Missile Defense (SMD) program. Its radar stations guard the east and west coasts of the United States against missiles launched from enemy submarines and surface vessels.
Space surveillance
involves the use of extremely powerful radars to detect and track artificial satellites and other objects put into orbit around Earth. For this purpose, the United States operates the Space Surveillance Network (SSN). The U.S. Air Force coordinates the network, which includes about 20 sites around the world that use radars and satellites to track other satellites and space debris. Each day, the SSN observes the locations of thousands of orbiting objects. Data from these observations can help identify reconnaissance satellites, which are used for spying.
Intelligence gathering.
Government agencies and the military use radar to collect information about preparations other countries might be making for war. A mapping radar in a plane can produce detailed maps of the ground and show military installations and equipment. Other types of radar provide important information about another country’s missile systems by monitoring its missiles during test firings.
Range instrumentation.
Radar is often used at rocket test ranges to check the performance of military equipment. For example, range instrumentation radars can accurately track the flight of a new missile. If the missile does not perform as well as expected, the tracking data might help the designer determine what went wrong.
Weapon fire control.
Radar can locate objects so precisely that it is used to aim and fire many kinds of weapons. Radar controls the firing of antiaircraft guns on tanks and warships. It directs guided missiles from jet fighters and from land-based launching sites. In addition, planes with radar bombsights can drop bombs accurately on targets at night or in bad weather.
In controlling automobile speed and traffic.
Police in many countries use radar to enforce speed laws by checking the speed of motor vehicles on streets and highways. Their portable radar sets can detect speeding vehicles up to 1/2 mile (0.8 kilometers) away.
In weather observation and forecasting.
Radar has an important role in short-term forecasting of local weather conditions. Radar echoes can be detected from raindrops and ice particles in clouds up to 250 miles (400 kilometers) away. Meteorologists use storm surveillance radars to monitor developing weather conditions and to map regions of heavy precipitation. They can estimate precipitation rates and accumulations by measuring the strength of reflected radar signals. Storm echoes from weather radars are displayed on weather segments of television news broadcasts and on Internet websites that feature weather reports. The weather services of many countries operate national radar networks to monitor precipitation.
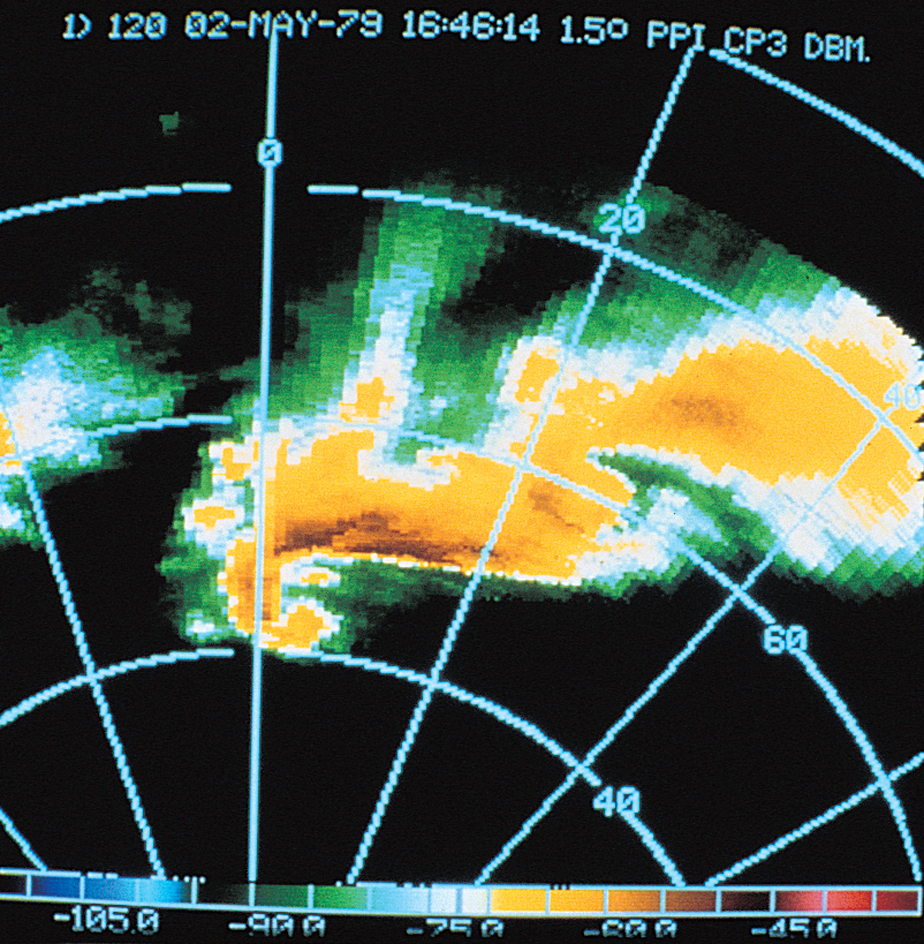
Most weather surveillance radars can measure the wind speeds and airflow patterns inside storms. Radar data help save lives by providing early warnings of weather dangers. These dangers include heavy rain; hail; wind shear, a sudden change in wind direction or speed; and mesocyclones, rotating columns of air that usually produce tornadoes. Weather services often issue tornado warnings on the basis of radar data alone, before anyone has actually sighted a tornado.
Data from short-range weather radar systems at airports provide immediate warnings to help pilots avoid aviation weather hazards. Even shorter-range weather radars on board many airplanes give pilots a maplike view of thunderstorms as they fly between airports.
Meteorologists use wind-profiling radars to gather information on wind speed and direction at various altitudes up to about 59,000 feet (18,000 meters). Computers can use this information to generate forecasts covering several days.
In space travel.
The first step in a mission into space is to launch a spacecraft into orbit around Earth. During the launch, mission controllers use a system of ground-based radars and other radio equipment to track the vehicle. As soon as the spacecraft enters orbit, the radars measure the orbit’s size and shape. Using the measurements, computers calculate when the craft’s remaining rocket engines should be fired and for how long to send the vehicle from Earth orbit into outer space.
Spacecraft designed to land on the moon or on another planet carry landing radar. This instrument measures the altitude of the spacecraft above the landing site and the rate of descent. Such information is used to regulate the engines of the craft so that it lands at the correct speed. If the vehicle descends too fast, it will crash. If it lands too slowly, it will burn too much fuel. Radar is also used to select stable, level landing sites for spacecraft.
A mission may call for a spacecraft to dock with another space vehicle. The astronauts in the spacecraft locate the other vehicle with radar. They then use the radar data to adjust the direction and speed of their own craft to perform the docking maneuver.
In scientific research.
Scientists rely on radar in conducting various kinds of studies. Meteorologists employ radars to study how clouds affect climate and climate change. Special cloud radars detect the tiny water droplets and ice crystals that make up clouds, as well as the precipitation they form.
Scientists use high-powered radars to investigate Earth’s upper atmosphere. At altitudes of 60 miles (100 kilometers) and higher, the air reflects radio waves. This air is in a part of the upper atmosphere known as the ionosphere. In this region, the sun’s radiation is so strong that it breaks air molecules into electrically charged particles called electrons and ions. As a result, the ionosphere can be studied by radar from Earth’s surface. Radar observations help scientists determine the temperature of the upper atmosphere and the kinds of gases in the air. Radar observations also indicate how fast winds blow at such high altitudes during different periods of the day.
Radar equipment and techniques contribute much to the study of the solar system. Astronomers have made radar observations of the moon, the sun, and the planets closest to Earth. They have even collected radar echoes from several of Jupiter’s largest satellites. Such radar observations provide extremely accurate measurements of the distances to these objects. They also show how rapidly the objects rotate. Astronomers have obtained detailed radar maps of the moon and Mars by recording radio waves bounced off their surfaces. By using the same technique, astronomers have succeeded in penetrating the thick clouds that surround Venus and discovered enormous mountains and valleylike features on its surface. See Telescope (Radio and microwave telescopes).
Zoologists depend on radar to trace the flight patterns of birds that migrate at night or that are too small to be seen from the ground. Radar can also be used to measure and map currents on the ocean surface. Such information is useful for research in marine biology and for planning offshore oil-drilling projects.
Geologists and engineers use ground-penetrating radar to detect underground features and objects within about 50 feet (15 meters) of the surface. They pull the small, boxlike antenna of such a radar across the terrain. The radar can detect soil layers and such buried objects as pipelines, archaeological artifacts, and land mines.
How radar works
Radar sets differ in design and purpose, but they all operate on the same general principles. All radars produce and transmit signals in the form of electromagnetic waves—that is, related patterns of electric and magnetic energy. These electromagnetic waves include radio waves and light waves. A true radar transmits radio waves. But similar instruments commonly called optical radars or laser radars send out light waves. These instruments are also known as lidars, from _li_ght _d_etection _a_nd _r_anging.
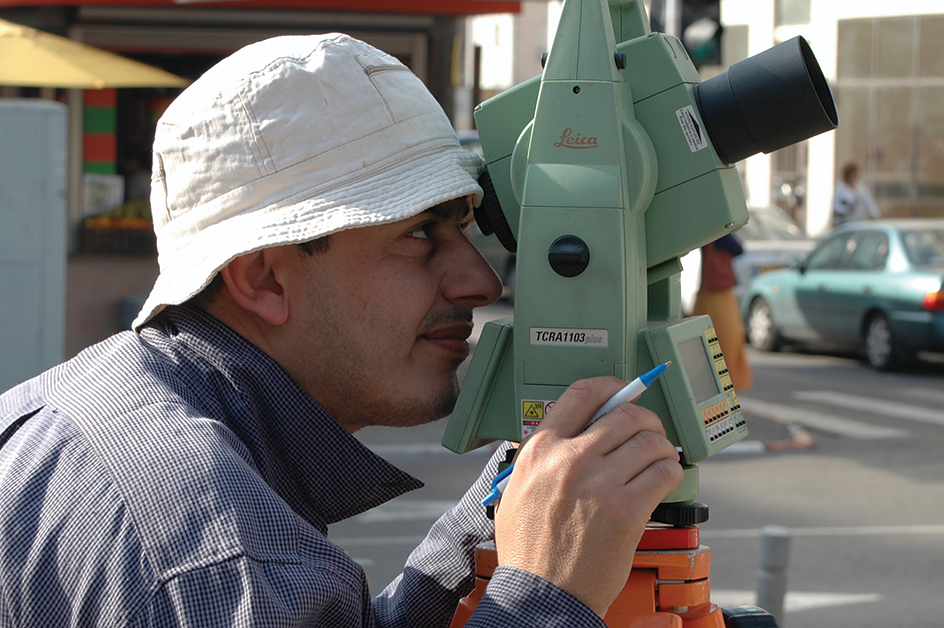
When the transmitted waves strike an object, they are reflected. Some of the reflected waves return to the set along the same path on which they were sent. This reflection closely resembles what happens when a person shouts in a mountain valley and hears an echo from a nearby cliff. In this case, however, sound waves are reflected instead of radio waves or light waves.
The waves transmitted by radar have a definite frequency. The frequency of such a wave is measured in units called megahertz (MHz). One megahertz equals 1 million hertz (cycles per second). Radio waves have lower frequencies than light waves have. Most radars that transmit radio waves operate at frequencies of about 3 to 300,000 MHz. Optical radars operate at much higher frequencies. Some generate light waves with frequencies up to 1 billion MHz.
In many cases, radar sets designed for different purposes operate at different frequencies. Radars that transmit at lower frequencies are more effective than high-frequency radars in penetrating clouds, fog, and rain and so are widely used on planes and ships. On the other hand, high-frequency radars provide precise direction measurements with much smaller antennas than those used by lower frequency radars. Optical radars are especially useful for surveying rough terrain where distant points have to be measured between such objects as large rocks and trees. Over-the-horizon radars use relatively low-frequency radio waves between 3 and 30 MHz. These waves reflect from the ionosphere and can reach beyond the horizon to detect ships, planes, and ocean surface currents at great distances. Ground-penetrating radars also use low frequencies. Radars of the North Warning System use relatively high-frequency radio waves called microwaves, which pass through the ionosphere.
Radar sets also differ in how they transmit signals. On this basis, they are usually classified into two general types: (1) pulse radar and (2) continuous-wave radar. Pulse radar is the more common type.
Pulse radar
sends out signals in powerful bursts, or pulses, of waves. These pulses last only a few millionths of a second. A pulse radar set usually has only one antenna, which alternately transmits the pulses and receives their echoes.
The distance to an object is found by measuring the time it takes a radar wave to reach the object and return. Radar waves, like all other electromagnetic waves, travel at the speed of light—186,282 miles (299,792 kilometers) per second. Therefore, a radar wave that returns after two seconds would have traveled 372,564 miles (599,584 kilometers)—186,282 miles to the object and 186,282 miles back. Using a measurement of the time required for the round trip, a pulse radar automatically calculates the distance to the object.
The antenna focuses the transmitted energy and sends out the pulses of waves in a narrow beam, enabling the set to determine an object’s direction. Only an object within the area of the beam can reflect the waves. Thus the direction from which the waves are reflected to the antenna indicates the location of the object.
Pulse radar can track an object by transmitting signal pulses and measuring the object’s distance and direction at regular intervals. It also can be used to make radar maps. Radar maps are produced by scanning a beam of pulses over an area and plotting the strength of the echoes from each direction. The echoes appear on the radar display screen as spots or as images of various colors and brightnesses and are stored as digital (numeric) data by a computer. Such objects as buildings, bridges, and mountains produce especially bright images because they produce strong echoes.
If an object in the radar beam is moving, the frequency of reflected waves differs from that of the transmitted waves. An object moving away from the antenna will return waves with a lower frequency, and an approaching object will cause a shift to a higher frequency. This phenomenon is called the Doppler effect, and the frequency change is known as a Doppler shift. The faster a tracked object is moving, the greater the Doppler shift. Special electronics in some pulse radar systems can indirectly measure the Doppler shift to determine the speed of a moving object. Modern storm surveillance and wind-profiling radars are pulse Doppler systems. Loading the player...
Doppler effect
Continuous-wave radar,
or CW radar, sends out its energy continuously rather than in short bursts. It uses one antenna for transmitting and another for receiving the reflected waves. CW radar directly measures Doppler shift, but not the distance to the moving object. The military uses CW radar to check the speed of incoming aircraft, and the police use it to watch for speeding automobiles on the highway. It is also used in baseball stadiums to clock the speed of pitches.
A more complex form of CW radar is frequency-modulated continuous-wave radar, or FM-CW radar. In addition to transmitting continuously, FM-CW radar rapidly increases or decreases the transmitted frequency of its signal at regular intervals. This feature enables FM-CW radar to determine the distance to a moving or stationary object. By the time the signal reaches an object and returns, the transmitter’s frequency will have changed. The difference between the frequency of the echo and that of the transmitter is measured, and the measurement is used to calculate the distance from the antenna to the object. The farther away an object is, the greater the difference in frequency.
Like pulse radar, FM-CW radar is used for mapping and tracking. It also serves as an altimeter for airplanes.
The parts of a radar set
Radar sets vary widely in size. A set’s size depends mainly on its use. For example, the sets used by motorcycle police to detect speeding cars can be held in the hand and may weigh less than 2 pounds (0.9 kilogram). Many of the huge radar units used to study planets and other distant objects occupy large buildings. One radar unit is built into a valley and has an antenna that measures 1,000 feet (305 meters) in diameter.
Although radar sets differ in size, most have similar parts. These parts include (1) the oscillator, (2) the modulator, (3) the transmitter, (4) the duplexer, (5) the antenna, (6) the receiver, (7) the signal processor, (8) the display, and (9) the timer.
The oscillator
is a device that generates a low-power electric signal of a constant frequency. This frequency determines the operating frequency of a radar set.
The modulator.
In pulse radar, the modulator is an electronic switch that rapidly turns the transmitter on and off. It causes the transmitter to produce short pulses of waves. In FM-CW radar, the modulator varies the frequency of the continuously transmitted wave.
The transmitter
serves as an amplifier. It takes the low-power electric signal generated by the oscillator and produces a high-power electromagnetic wave. For example, the transmitter of a pulse radar used in air traffic control may produce wave pulses with a peak power of several million watts.
The duplexer
makes it possible to transmit and receive using the same antenna. It routes the electromagnetic waves from the transmitter to the antenna and prevents them from flowing into the receiver. The powerful waves from the transmitter would damage the sensitive receiver if they flowed into it. After the waves have been released through the antenna, the duplexer connects the receiver to the antenna. This switching action enables the receiver to pick up incoming echoes.
The antenna
sends out radar waves in a narrow beam. It also collects the reflected echoes. Most modern radar units have a duplexer, and so they use the same antenna for transmitting and receiving.
The most common type of antenna consists of a horn attached to the front of a large reflecting dish called a reflector. The horn sends out the radar waves, and the reflector focuses them into a narrow beam. The antenna rotates so that the beam sweeps around the radar station, scanning for objects in all directions.
Other types of antennas are used in radar sets that operate either at extremely low frequencies or at extremely high frequencies. Radars that transmit low-frequency radio waves have an antenna made of metal tubes or rods. Such antennas resemble the outdoor aerials of TV sets. A device called a laser generates the intense beam of light used by an optical radar. In such a radar, lenses control the size of the transmitted beam and capture the light waves returning from the target.
An antenna generally transmits wave pulses for much briefer periods than those during which it receives reflected waves. In typical weather radars, for example, the antenna transmits pulses for only about one-millionth of a second and then receives waves for about one-thousandth of a second.
The receiver
takes the weak echoes collected by the antenna and greatly amplifies them. It is so sensitive that it can easily detect echoes of less power than a millionth of a millionth of a watt. The receiver also filters out much of the noise and other interference picked up by the antenna.
The signal processor.
In most radar sets, the incoming waves from the receiver pass through a signal processor before going to the display. The signal processor performs different tasks in radars used for different purposes. In many radar units, it blocks out echoes from large, fixed objects and allows only echoes from small, moving targets to reach the display. By doing so, the signal processor enables the operator of a radar set to see an airplane, for example, even though the echoes from the plane arrive at the same time as much stronger echoes from a mountain. A computer serves as the signal processor in most modern radars.
The display
presents radar operators with information obtained about an object. Some sets have a simple display. The portable Doppler radars used by the police, for example, have a meter that indicates the speed of a vehicle. But most radar sets have a more complex display that consists of a computer screen or some other type of display screen. Such a display can present radar data in several forms. The most common form is the Plan Position Indicator, generally called the PPI.
The PPI provides a circular, maplike picture of the area scanned by the radar beam. The center of the picture corresponds to the location of the radar set. The screen has a compass scale around its edge for direction readings. The screen might also have geographical landmarks and rings spreading out from the center of the picture to mark distance in miles or kilometers. Radar echoes appear as bright or colored spots. The position of a spot with respect to the compass scale shows the direction of the object. The distance of the spot from the center indicates how far away the object is. The speed of a moving object can be determined by noting the time it takes a spot to travel a certain distance on the radar screen, or it can be measured using its Doppler shift and displayed instantaneously, without waiting for the spot to travel.
Other display presentations show the elevation of an object. Such a presentation is used with radar sets designed to help direct aircraft landings.
The timer
ensures the smooth, efficient operation of a radar set. This device automatically turns other major parts of the radar set on and off at precisely the right time. The timer does so by sending control signals to the various parts of the system in the proper sequence.
The development of radar
The theories and experiments of many scientists led to the development of radar. James Clerk Maxwell, a Scottish mathematician and physicist, made the first major contribution. During the 1860’s, Maxwell said that there existed then-undiscovered kinds of electromagnetic waves that travel at the speed of light. He also proposed that devices might be developed to generate such waves. In the late 1880’s, Heinrich R. Hertz, a German physicist, proved Maxwell’s ideas correct by producing radio waves. In addition, Hertz demonstrated that such waves could be reflected from solid objects.
Hertz’s discovery promoted widespread efforts to find ways of using radio waves for communication. Some scientists realized that radio waves might also be used for detecting distant objects. However, little research could be done until basic radio equipment was developed. Devices for sending and receiving radio signals over long distances became available by the early 1900’s.
The first uses of radar.
In 1925, two American physicists, Gregory Breit and Merle A. Tuve, bounced short radio pulses off the ionosphere. They determined the height of the ionosphere by measuring the time taken by the reflected signals to return. Many scientists consider this experiment to have been the first practical use of radar. The success of the experiment encouraged researchers in many countries to conduct further scientific studies of the ionosphere with similar equipment and techniques.
Scientists also began experimenting with radio echoes to detect airplanes and ships. Much of the early work in this area was done by Robert A. Watson-Watt, a Scottish engineer and physicist. In 1935, he and a team of British scientists refined the pulse techniques used in ionospheric studies to locate aircraft at distances up to about 17 miles (27 kilometers).
The growing threat of a world war stimulated efforts to improve radar technology during the late 1930’s. Before World War II began in 1939, the British had built a chain of radar stations along the east and south coasts of England for defense against air and sea attacks. By 1940, the United States was producing pulse-type radar for tracking planes and for controlling antiaircraft guns. Germany also had similar kinds of radar by about the same time. Japan and the Soviet Union developed radar-warning systems a few years later.
Advances during World War II.
The radar sets available at the beginning of the war proved extremely valuable for military operations. As a result, scientists were urged to develop even better equipment.
American and British radar experts cooperated closely during the war and produced important advances. The British were working to improve a special kind of vacuum tube called the magnetron. By late 1939, their version of the magnetron could generate pulses of microwave energy at high enough power levels to be used in radar systems. In 1940, the British turned it over to the Americans for further development and manufacturing.
The magnetron contributed greatly to the development of modern radar. This vacuum tube generates microwaves—that is, short radio waves with frequencies of more than 1,000 MHz. These high-frequency waves can be concentrated into narrow beams without the use of a huge radar antenna. Microwaves thus made it possible to design radar units small enough for aircraft, patrol boats, and mobile ground stations. In one of the earliest applications of airborne radar, Allied forces located German submarines from a distance as they surfaced.
Before the war ended in 1945, both the Allies and the opposing Axis forces had developed methods of making enemy radar less effective. In one widely used method, planes on bombing missions dropped countless numbers of metal foil strips called chaff. Each strip reflected radio signals like a radar target. The bombers filled the air with so many strips that enemy radar operators had difficulty recognizing echoes from the planes.
In another countermeasure, planes and ships carried high-powered radio transmitters. These transmitters produced enough interference to prevent enemy radar from receiving echoes from the planes and ships. Engineers also designed equipment that received pulses from enemy radar and sent them back at an increased power level after a short pause. As a result, false targets appeared on the display of the enemy radar and drew attention from the real targets.
Continued progress.
During the early 1950’s, American scientists worked on a type of vacuum tube called the klystron. They succeeded in developing a high-powered klystron, which is well-suited for radars that require a constant microwave frequency from one pulse to the next. This development helped increase the accuracy of radar. Scientists also worked to improve radar sensitivity. By the late 1960’s, they had designed receivers that produced little internal noise, which interferes with the reception of faint echoes.
The rapid development of the computer after World War II contributed much to radar technology. Computers make effective signal processors. They can analyze echoes efficiently at high speeds and present the information obtained in a form useful to radar operators.
Radar also benefited from the invention of the transistor in 1947 and of related solid-state electronic devices during the 1950’s and 1960’s. These devices enabled engineers to build lighter and more reliable radar sets. In addition, engineers used a solid-state device called a phase shifter to develop a new kind of radar. This radar, known as a phased array radar, moves its signal beam electronically rather than by rotating an antenna. Phased array radars are especially useful in situations where the signal beam must be moved rapidly from one target to the next.
In the late 1960’s, physicists perfected the laser. Their work led to the development of optical radars, also called lidars, which operate at the high frequencies of laser light. Such instruments require only a small antenna to send out an extremely narrow signal beam.
Radar in the future.
Researchers today are seeking ways to reduce the size and production costs of microwave radars. Pocket-sized radar units could be widely used as aids for blind people and as collision-warning devices in cars. Radars on orbiting satellites could provide worldwide measurements of precipitation and cloud conditions. Special polarimetric radars will be able to identify objects by shape, enabling scientists to distinguish between rain, snow, and swarms of insects, for example. Microwave navigation radars built into a single artificial satellite might one day track ship and aircraft traffic over most of Earth.