Semiconductor is a material that conducts electric current better than an insulator like glass, but not as well as a conductor like copper. A computer chip is a piece of a semiconductor, usually silicon, that contains an electronic circuit. Solar cells that convert light energy to electric energy are made of semiconductors, as are some lasers. Silicon (Si) is the most widely used semiconductor. Other major semiconductors include germanium (Ge) and gallium arsenide (GaAs).
Semiconductor crystals.
Unlike an insulator, a semiconductor will conduct a small electric current at room temperature. And unlike a metal, a semiconductor will conduct more current as its temperature is increased. These characteristics are a result of the semiconductor’s atomic and crystal structure.
For example, a normal silicon atom has a nucleus made up of 14 protons and 14 neutrons. Surrounding the nucleus are 14 electrons. The atom is electrically neutral: The neutrons have no net electric charge, and the positive charge of the 14 protons balances the negative charge of the 14 electrons.
The electrons are arranged in three shells that can be thought of as different-sized spheres with the nucleus in the center. The innermost shell has two electrons, the middle shell has eight, and the outermost shell has four.
The outermost four electrons are responsible for holding the crystal together. Each atom is bonded (joined) to four others, except for the atoms on the crystal’s surface. Each bond consists of a pair of electrons—one from the outermost shell of each of two atoms.
Electrons always vibrate, and the amount of the vibration depends on temperature—the higher the temperature, the greater the vibration. At room temperature, some of the outermost electrons vibrate so much that they leave their bonds. They become free electrons—electrons that can move about in or on the crystal.
Current is a flow of charge. If a battery is connected to the crystal, free electrons will flow as current. Because the number of free electrons depends on temperature, the amount of current will also depend on temperature.
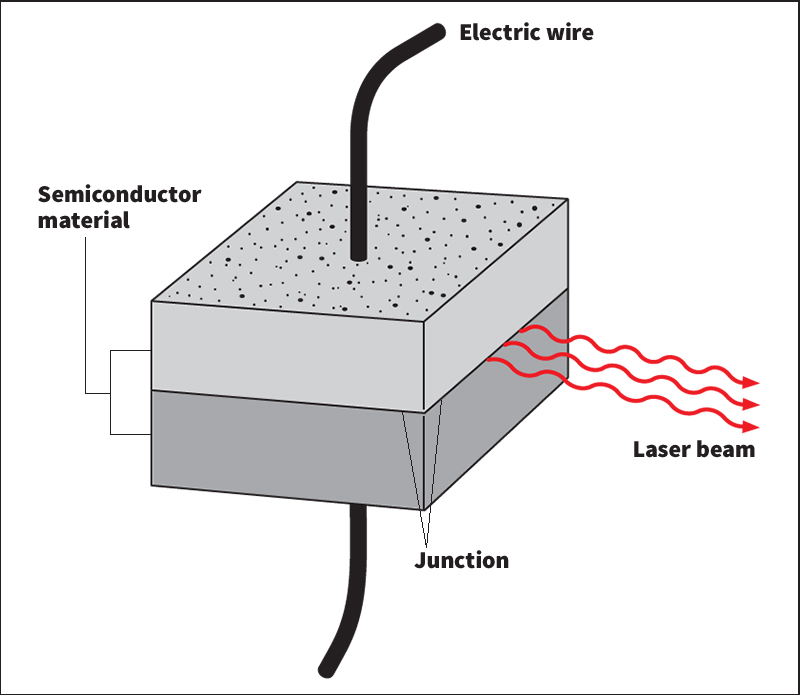
Doped crystals.
Semiconductor devices use crystals containing tiny amounts of dopants—atoms of other substances. A crystal can be n-doped or p-doped.
N-doped crystals
usually use phosphorus as the dopant. A normal phosphorus atom has 15 protons, 16 neutrons, and 15 electrons. Five of the electrons are in the outermost shell. Only four of them are needed for bonding with the four surrounding silicon atoms. As a result, the fifth one can easily become a free electron—an extra charge carrier. N-doped crystals get their name from the negative charge of their extra charge carriers.
P-doped crystals
usually use boron dopant. A normal boron atom has five protons, six neutrons, and five electrons. Three of the electrons are in the atom’s outermost shell. Each of these electrons is in a bond with a surrounding silicon atom. As a result, the fourth silicon atom has an unpaired electron in its outermost shell. That is, there is a hole in the crystal.
A nearby bonding electron can easily gain enough energy to leave its bond and move into the hole. The electron leaves behind a hole that can be occupied by yet another electron. This process can continue as long as the crystal is warm enough. For convenience, designers of electronic devices refer to the process as a flow of holes, rather than a succession of movements of individual electrons. Because electrons are negatively charged, the holes are positive. P-doped crystals get their name from this positive charge.
Using doped crystals.
A solar cell is one of the simplest electronic devices. It consists of an n-type layer of silicon next to a p-type layer of silicon. The boundary between the layers is known as the junction.
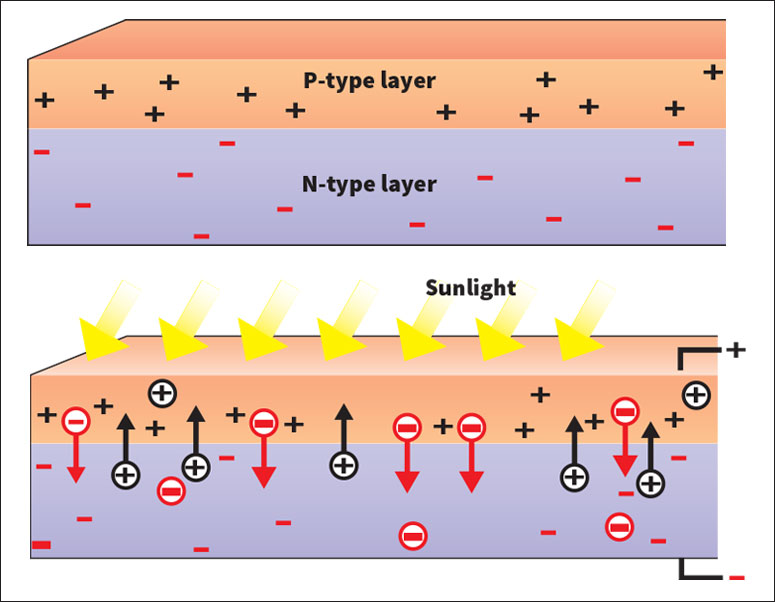
When the cell is produced, electrons cross the junction. They leave the n-type layer near the junction and fill holes in the p-type layer near the junction. A boron atom that gains an electron in this way becomes negatively charged-it has six electrons but only five protons. Thus, the movement of electrons creates a negatively charged region next to the junction in the p-type layer.
In addition, holes leave the p-type layer near the junction and replace electrons in the n-type layer. Phosphorus atoms that gain a hole become positively charged. Thus, the movement of holes creates a positively charged region next to the junction in the n-type layer.
Because negatively charged objects repel each other, it eventually becomes much more difficult for any more electrons to flow from the n-type layer to the p-type layer. And because positively charged objects repel each other, it becomes much more difficult for any more holes to flow from the p-type layer to the n-type layer. As a result, a balanced condition occurs, with regions of opposite charge near the junction.
However, because of the attraction between oppositely charged objects, free electrons created in the p-type side of the junction flow to the n-type layer, and free holes created in the n-type side of the junction flow to the p-type layer. Sunlight striking the cell creates free electron-and-hole pairs by giving up its energy to them. Electrons build up in the n-type layer, and holes, in the p-type layer. If a wire is connected to both layers opposite the junction, current will flow through the wire. The current will consist of the separated free electrons and holes returning to their original layers.