Solar energy is the direct use of sunlight to produce heat or electric power. The sun’s energy is plentiful. But it is thinly distributed over a large area. The energy must be collected and concentrated to produce usable power. As a result, solar energy is a more expensive power source than fossil fuels for most applications. Solar technology is improving rapidly, though. Someday, it may provide a clean and abundant source of power.
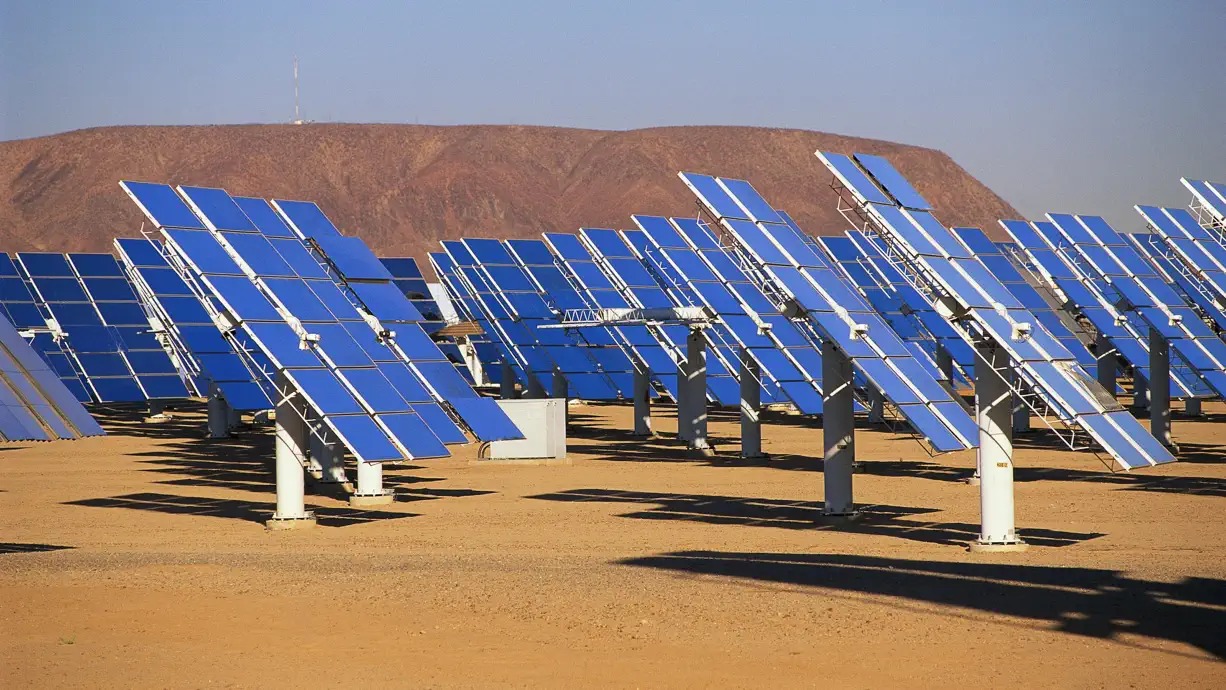
There are two chief ways that sunlight may be converted into electric power. It may be converted directly, in a process called photovoltaic conversion. Or it may be changed by solar thermal conversion, which converts light to heat and then to electric power. Most solar thermal devices heat water to produce steam, which drives a steam turbine.
Nearly all the energy that we use is actually solar energy—energy from the sun. For example, solar energy stored in plants millions of years ago makes up such fossil fuels as coal, petroleum, and natural gas. Hydroelectric power plants harness the energy of moving water. There would be no moving water without the sun. The sun’s heat evaporates moisture so that it falls back to Earth as rain and other forms of precipitation. The sun also powers the air currents that cause the wind to blow. This article, however, discusses only solar electric and solar heating technologies. For information on other forms of solar energy, see the articles on the fossil fuels, such as Coal and Petroleum. Also see the articles on other forms of power, such as Water power and Wind energy .
Photovoltaic conversion
Devices called photovoltaic cells or solar cells produce electric current directly from sunlight. This ability results from the photovoltaic effect. The photovoltaic effect is a phenomenon in which the energy in sunlight causes electric charges to flow through layers of a conductive material to produce a useful electric current. See Photoelectric effect (The photovoltaic effect).
The development of photovoltaic cells.
The French physicist Alexandre Edmond Becquerel discovered the photovoltaic effect in 1839. He immersed two metal plates in a solution and observed a small voltage when one plate was exposed to sunlight. The first photovoltaic cells were made of an element called selenium. Selenium cells could convert only 1 percent of sunlight to electric power. As a result, the cells remained just a curiosity for many years.
In 1954, scientists at Bell Telephone Laboratories (then the research department of the Bell Telephone Company) invented the first photovoltaic cell that could produce a useful amount of electric power. The Bell scientists were chemist Calvin S. Fuller and physicists Daryl M. Chapin and Gerald L. Pearson. They developed a solar cell with an efficiency of 6 percent, six times better than the best selenium cells. A solar cell’s efficiency measures the percentage of sunlight striking the cell that it turns into electric power. The Bell scientists made their cell from purified silicon, the material used to make computer chips. Silicon is a semiconductor. A semiconductor is a material that conducts electric current better than an insulator but not as well as a conductor.
How a photovoltaic cell is made.
The most common type of photovoltaic cells are crystalline silicon cells. They are so named because every atom in the cell is part of a single crystal structure. To make a crystalline silicon cell, a manufacturer begins with a thin wafer of silicon that has been doped (treated) with an impurity. The impurity, usually the element boron, is called a p-type dopant. The p stands for positive. The addition of the dopant causes local deficits (shortages) of electrons, called holes, to appear in the material. The doped material is called a p-type material. A hole can pass from one atom to another and migrate around the crystal.
An n-type dopant (n for negative), such as phosphorus, is then diffused (spread out) part way into the p-type material. The addition of this dopant produces local excesses of electrons. This layer is then called the n-type material. A potential (difference in electrical charge) is thus set up between the two layers. When sunlight strikes the photovoltaic cell, the light’s energy forces the negative and positive charges in the semiconductor to separate. The charges accumulate at electrodes joined to each of the two layers. An electric current will then flow through a wire connecting the two electrodes.
Using photovoltaic cells.
For most applications, engineers wire together many cells in a grouping called a module to produce a desired voltage. Multiple modules may be connected in an arrangement called an array to produce the required current for the application. Silicon cell efficiencies have reached 25 percent without special equipment to concentrate sunlight. Compound photovoltaic cells combined with solar concentrating systems have achieved 35 percent efficiency. Researchers have also made solar cells from a number of other semiconductor materials.
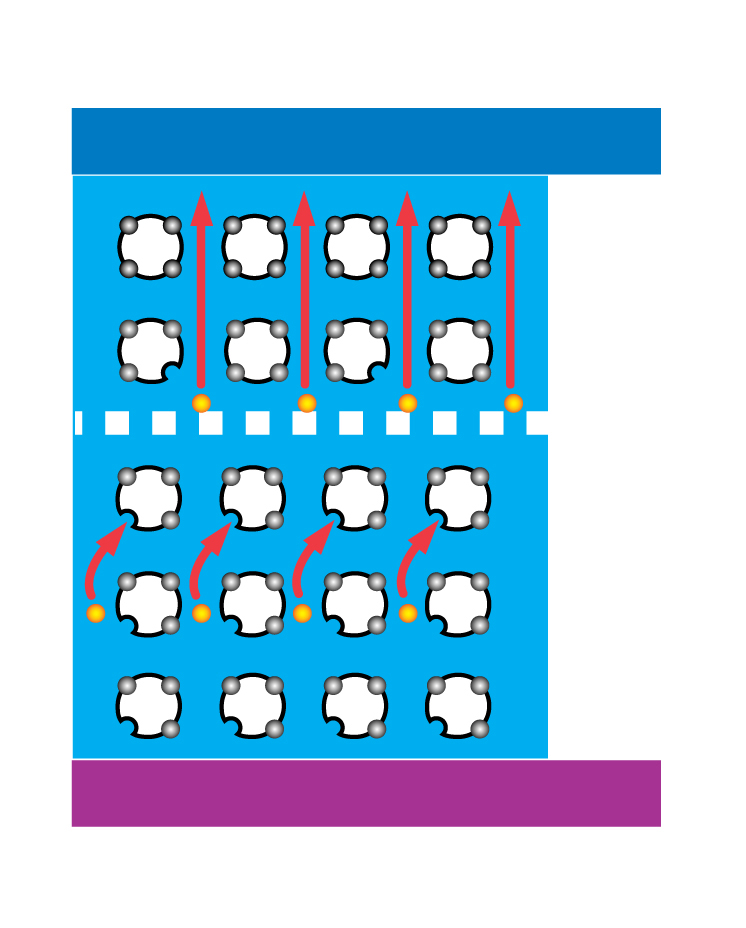
Today, solar cells provide power for spacecraft and artificial satellites, handheld calculators, and wristwatches. Solar cells are also used for electric power generation in remote areas, where extending power lines would be difficult or costly. Most photovoltaic systems require a storage facility. The storage facility normally consists of batteries. Excess energy is stored in the batteries during the day and extracted as needed during the night.
Other solar cells.
Researchers are also studying solar cells called photoelectrochemical cells. The simplest such cell is similar to the device made by Becquerel in 1839. However, today’s cells are much more stable and efficient. In addition to producing electric current, photoelectrochemical cells can be used to chemically split water directly into oxygen and hydrogen gas. The hydrogen can then be burned as fuel.
Solar thermal conversion
Solar thermal conversion systems, also called solar concentrators, use one or more reflectors to concentrate solar energy to high levels. There are three major kinds of systems—parabolic trough systems, parabolic dish systems, and central receivers.
Parabolic trough systems
are the simplest of the solar thermal systems. A parabola is a type of curve. In such a system, a parabolic (curved) trough covered with rows of reflectors moves to track the sun. The reflectors focus sunlight to a line. The line of sunlight strikes a fluid-filled pipe at the center of the trough. The fluid in the pipe is a heat-absorbing substance. It may reach temperatures above 750 °F (400 °C). The heat can be used to make steam to generate electric power or to merely heat water. Trough systems can concentrate solar radiation to 100 times the intensity of normal sunlight. Power plants using this technology in southern California provide about 350 megawatts of electric power per year. That is enough power for a community of about 350,000 people.
Parabolic dish systems
resemble parabolic trough systems except that they focus light to a point instead of a line. Parabolic dish systems have reflectors arrayed along the contour of a bowl-shaped structure called a dish. Such systems can achieve concentrations up to 10,000 times the intensity of normal sunlight.
Central receiver systems
use an array of sun-tracking mirrors, called heliostats. The mirrors reflect light onto a single central tower called a receiver. A central receiver system called Solar Two operates in the Mojave Desert near Barstow, California. It has 1,926 heliostats that focus sunlight on a single receiver. The receiver is filled with a mixture of molten sodium and potassium salts. This mixture holds heat longer than other fluids. After the molten salt is heated, the system pumps it into insulated tanks. When power is needed, the molten salt is pumped into a device called a heat exchanger. In the heat exchanger, it produces steam that turns a turbine. Solar Two’s storage capability enables it to operate even after dark or when the sun is covered by clouds. The plant supplies 10 megawatts of electrical power. Other central receiver test facilities operate in Almeria, Spain, and Rehovot, Israel.
Solar heating
Solar heating requires an efficient absorber to collect sunlight and convert it to heat. The absorber may be as simple as a coating of black paint. Or it may be a textured, heat-absorbing ceramic. A good absorber collects 95 percent or more of the solar radiation while emitting 20 percent or less of the heat energy an ordinary hot surface would.
There are several methods of solar heating. One common method uses windows as solar collectors, as in a greenhouse. The windows trap the sun’s heat. The heat passes back through the windows, roof, and walls slowly.
The simplest solar collectors are flat-plate collectors. The plates are fixed, and the sun shines on them at various angles as it moves. The sun heats fluid inside the plates to a temperature of up to 212 °F (100 °C). The hot fluid flows to a heat exchanger, a device like an automobile radiator through which water circulates. The hot fluid transfers its heat to the water. The hot water is used to warm buildings in a conventional hot-water heating system or to heat their hot-water supplies.
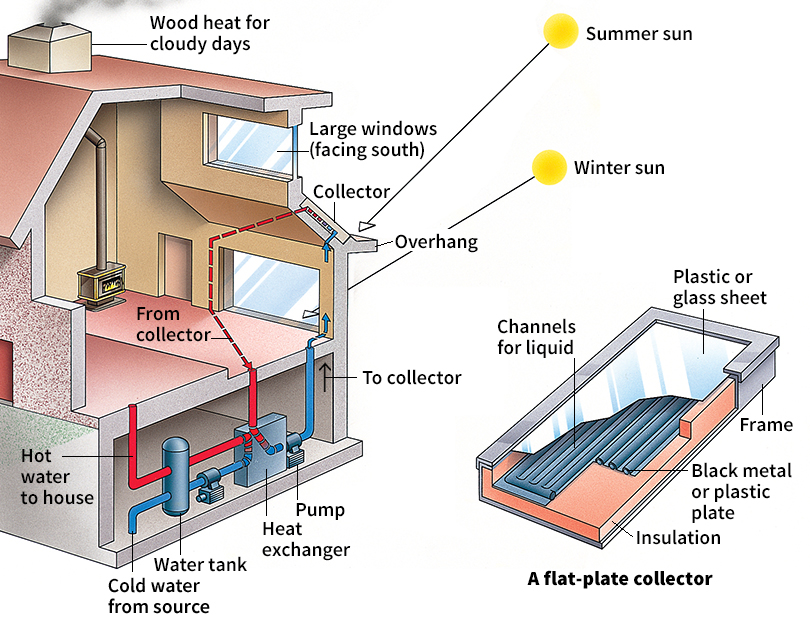
Another kind of collector, designed specifically for heating air, is the transpired solar collector. Such collectors consist of flat or ridged plates pierced by an array of small holes. Air is drawn through the holes and is heated by the sun-warmed plates. As much as 80 percent of the solar energy collected by the plates is transferred to the air stream.
A solar furnace is a type of solar collector that concentrates sunlight to produce temperatures high enough for use in industrial processes. Scientists use solar furnaces to process steel, ceramics, and other materials. Scientists also use solar furnaces to pump (provide energy for) solid-state lasers and to destroy hazardous wastes. One such furnace operates at the National Renewable Energy Laboratory in Golden, Colorado. In the Colorado installation, a single mirror tracks the sun. The tracking mirror directs solar radiation into a series of concentrating mirrors. The mirrors reflect the beam of light through a system of attenuators and shutters. These devices control the intensity of the beam and turn it on and off. This solar furnace has achieved concentrations of light 50,000 times greater than that of normal sunlight. Such highly focused energy can heat materials to temperatures as high as 6300 °F (3500 °C). Other solar furnaces operate in France, Germany, Israel, Spain, Switzerland, and Uzbekistan.