Space exploration is the act of traveling to places beyond Earth to enrich humanity’s understanding of the universe. Space exploration is hazardous and costly. But many important questions cannot be answered without leaving Earth: What was our solar system like when it was young? How do planets form and evolve? Has life developed on other worlds?
Since the invention of spaceflight, robotic space probes have explored destinations throughout our solar system. Probes have visited all eight planets, dozens of moons, two dwarf planets, various asteroids and comets, and the sun. People have recorded firsthand observations from Earth orbit, lunar orbit, and the surface of Earth’s moon. On Earth, scientists, engineers, and technicians from many countries work together to design spacecraft, supervise missions, and make tough decisions in emergencies.
Someone who pilots a spacecraft or works in space is called an astronaut. Astronauts from former republics of the Soviet Union are called cosmonauts. Astronauts from other countries are sometimes given special titles, such as taikonaut for Chinese astronauts and spationaut for French astronauts. Although their titles differ, their duties are much the same. This article will use astronaut when referring generally to people from any country who work in space.
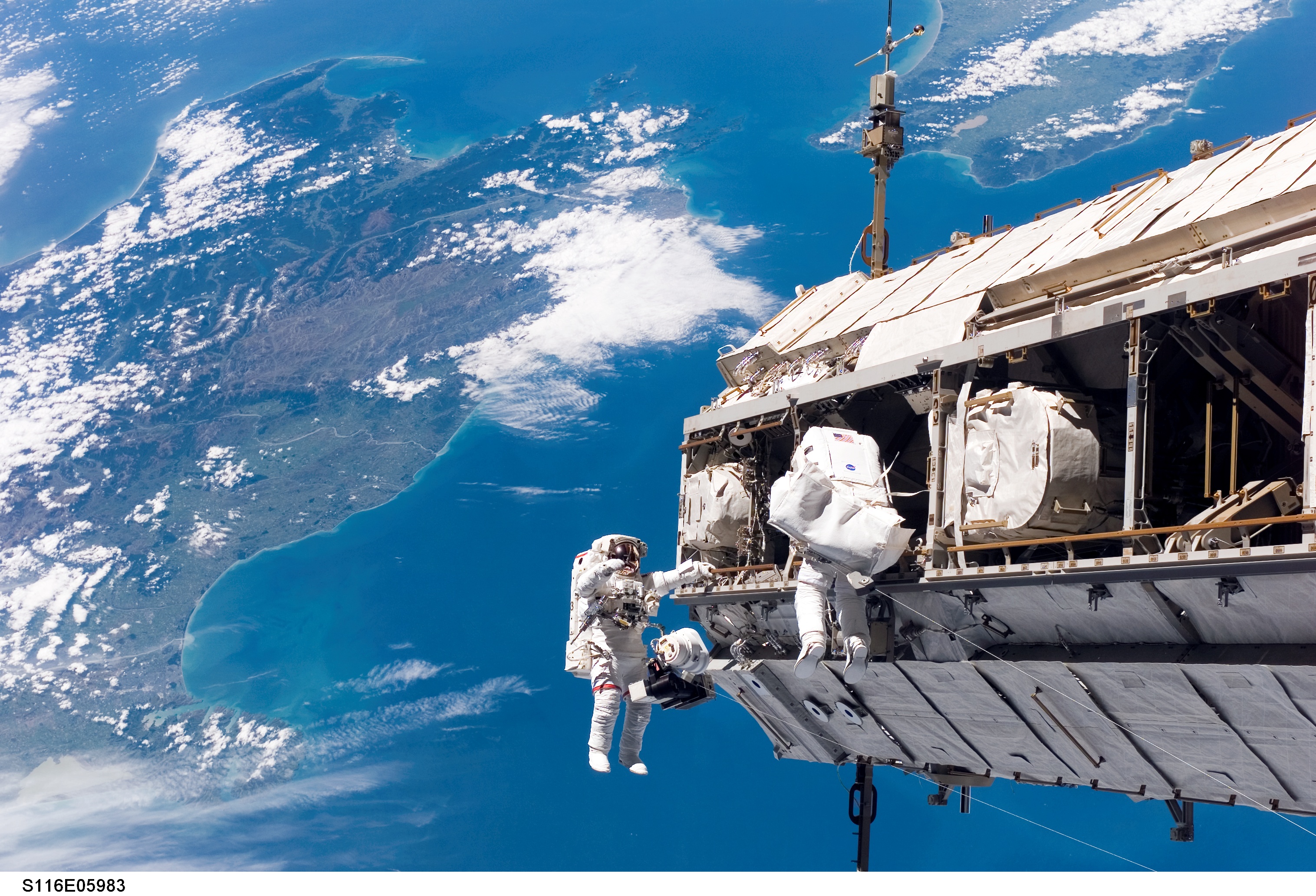
Success in space exploration requires great investment in science and engineering. Since the earliest spaceflights, nations have mounted space missions to demonstrate their technological capabilities to the world. When space travel first became possible, the United States and the Soviet Union were engaged in an intense rivalry called the Cold War. The two nations competed to develop impressive spaceflight technology and mount ambitious space missions.
In 1958, the U.S. government created the National Aeronautics and Space Administration (NASA), the first space agency. Today, numerous national space agencies exist around the world, including NASA, the Canadian Space Agency (CSA), the China National Space Administration (CNSA), the Indian Space Research Organisation (ISRO), the Japan Aerospace Exploration Agency (JAXA), and the Russian space agency Roscosmos. Some space agencies are international. For example, the European Space Agency (ESA) is the space program of the European Union. Private corporations, universities, and nonprofit organizations also research space exploration.
What is space?
Space is the nearly empty expanse that contains all objects in the universe. Planets, stars, and even galaxies are tiny specks compared with the vastness of space.
The beginning of space.
There is no distinct physical boundary between Earth’s atmosphere and outer space. Earth is surrounded by air, which makes up its atmosphere. As the distance from Earth increases, the air becomes thinner. To entirely leave the effects of Earth’s atmosphere, a spacecraft would need to travel over 600 miles (965 kilometers) away from Earth’s surface. But many spacecraft, including the International Space Station (ISS), operate much closer to Earth.
Space is governed by international treaties, but authorities disagree on the legal boundary between Earth’s atmosphere and space. Most authorities affirm that space begins somewhere beyond 60 miles (95 kilometers) above Earth’s surface. Many consider the Kármán line, which lies 62 miles (100 kilometers) above sea level, to be the official boundary of space. Some institutions, such as the U.S. military, claim that space begins just 50 miles (80 kilometers) above Earth’s surface.
The space just beyond Earth’s atmosphere contains particles of air and dust. There are also chunks of metal or rock called meteoroids. Various kinds of radiation flow freely. Thousands of spacecraft have been launched into this region of space.
More than 12,000 human-made objects can be found orbiting Earth, including rocket boosters, old satellites, bolts, garbage bags, and broken hardware. Most such objects eventually reenter the atmosphere. However, when satellites break apart, potentially dangerous debris is left behind.
Earth’s magnetic field, the space around the planet in which its magnetism can be observed, extends far out beyond the atmosphere. The magnetic field traps electrically charged particles from outer space, forming zones of radiation called the Van Allen belts.
The region of space affected by Earth’s magnetic field is called the magnetosphere. It is shaped like a teardrop, with the point extending away from the sun. Beyond this region, Earth’s magnetic field is overpowered by the sun’s magnetic field.
The region of space in which the moon orbits Earth is called translunar space. Space beyond the moon’s orbit is known as deep space.
Interplanetary space
is the space between the planets. In our solar system, the sun’s gravity affects the motion of all objects in interplanetary space.
Objects move through interplanetary space at great distances from each other. Venus is the planet that comes nearest to Earth, but when the two planets are closest, they are still 25 million miles (40 million kilometers) apart.
Astronomers measure distances within the solar system in astronomical units (AU). Earth’s average distance from the sun, 93 million miles (150 million kilometers), is equal to 1 AU.
Interstellar space
is the space between star systems. Distances in this region are so immense that scientists do not describe them in miles or kilometers. Astronomers measure these distances in units called light-years. For example, the nearest star to the sun is Proxima Centauri, 4.2 light-years away. A light-year equals 5.88 trillion miles (9.46 trillion kilometers). This is the distance light travels in one year at its speed of 186,282 miles (299,792 kilometers) per second.
Various gases, thin clouds of extremely cold dust, and a few escaped comets float through interstellar space. Interstellar space also contains many objects not yet discovered.
Traveling in space
The force of gravity,
also called gravitation, is the force of attraction that acts between all objects because of their mass. Because of gravitation, an object that is near Earth falls toward the planet. An object on Earth’s surface experiences a downward force. We experience this force on our bodies as our weight. At the surface of Earth, acceleration due to gravity, called g, is about 32 feet (9.8 meters) per second each second.
The force of gravity affects everything that moves through space. Gravity keeps the planets in their orbits around the sun, and moons in their orbits around planets. A spacecraft may use rockets to counteract the pull of gravity. Or it may allow the pull of gravity to carry it toward a destination. To leave Earth’s surface, a spacecraft must overcome the downward force of the planet’s gravity.
Launching into space
Months or years before a launch, project managers designate a time period in which conditions are best for a spacecraft’s mission and planned trajectory. This period is called the launch window. As the launch window approaches, launch directors select a date and time. Weather conditions, malfunctioning equipment, problems with the payload (cargo), or an astronaut feeling sick may delay a launch for several days. If an issue is dangerous or difficult to fix, the launch window may end before the spacecraft launches. Serious equipment problems can delay a launch for years. Loading the player...
Launch of a Soyuz rocket in 2009
To prepare for launch, a truck, tractor, or train transports the rocket and its payload very slowly to the launch pad. At the launch pad, the rocket is moved into position over a flame pit. Technicians load propellants (fuel and oxidizer) into the rocket through pipes.
The powerful rockets that launch spacecraft are called boosters. These rockets counteract the downward force of Earth’s gravity by creating upward thrust.
At launch time, the rocket engines ignite and begin to burn the propellant. This reaction forcefully expels gases, creating thrust. When the thrust exceeds the vehicle’s weight, the rocket lifts off the launch pad. Launch vehicles commonly have multiple stages (sections). The first stage, which is lit at launch, falls off after its propellant has been used up. The second stage then ignites. Without the discarded empty components, the vehicle is lighter, so the second stage rockets can propel it more easily.
A launch vehicle is enormous compared with its payload. NASA’s Curiosity rover weighs about 1,980 pounds (900 kilograms), but the vehicle that launched it weighed 1.17 million pounds (531,000 kilograms). This is because rockets need propellant to create thrust. The heavier the vehicle, the more thrust that is needed to lift it up, and the more propellant that is needed to create the thrust. But the propellant also adds to the vehicle’s weight. Propellant may make up 90 to 95 percent of a launch vehicle’s weight.
For more information about rocket launches, see Rocket.
Spacecraft navigation
Staying on course
is an essential part of space missions. Spacecraft must control their attitude (orientation). A spacecraft oriented in the wrong direction might not be able to communicate with Earth. A spacecraft that drifts from its planned trajectory may never reach its target.
Early probes were often equipped with star trackers to maintain attitude and keep antennas pointed at Earth. Star trackers are small optical devices that maintain a lock on a bright star. The most common star used for this purpose was Canopus, visible in the Southern Hemisphere. The star tracker would signal if a spacecraft drifted away from its correct alignment, and thrusters would be fired to correct the position.
As a spacecraft approaches its intended destination, other techniques are used to refine its position. Cameras on the spacecraft photograph nearby planetary bodies with stars in the background. Because the positions of the stars are well known, these images can be used to determine the position of the spacecraft relative to the planetary body.
One of the first tasks required in space navigation is determining velocity. Radio transmissions from a moving object are slightly distorted. This effect is called the Doppler shift. A spacecraft’s speed can be found by measuring the Doppler shift of its radio transmissions. Measuring the amount of Doppler shift enables engineers to determine the rate at which the spacecraft is traveling away from Earth.
Engineers also determine a spacecraft’s range (distance from Earth) using radio transmissions. The amount of time for a transmission to travel between a tracking station on Earth, the spacecraft, and back can be used to calculate the distance because the transmission travels at the speed of light, which is precisely known.
The angular position of the spacecraft must also be determined. The angular position is a measure of the probe’s location as seen from Earth. If engineers know a spacecraft’s angular position, range, and velocity, they can calculate its movement in three dimensions. Angular position can be crudely measured using an Earth-based antenna. More precise measurements are possible by using transmissions received simultaneously at two different Earth-based tracking stations.
All the space navigation techniques have one important aspect in common: they rely on accurate timekeeping. Atomic clocks are used at each tracking station. These clocks provide a stable timing reference to maintain constant transmission frequencies and allow data to be combined from more than one station.
A reaction control system keeps spacecraft correctly oriented. The reaction control system often includes thrusters, sets of small rockets that can respond to disturbances in the spacecraft’s trajectory. It may also include reaction wheels. A reaction wheel is a spinning device similar to a flywheel. Reaction wheels are important for spacecraft that need to remain extremely stable, such as a telescope that must remain oriented toward a fixed point.
Connecting multiple spacecraft.
A mission may need two spacecraft to rendezvous (approach each other closely). A rendezvous requires fine control of both spacecraft. They must travel very slowly relative to each other. At high speeds, an accidental collision can destroy spacecraft.
Docking procedures physically connect two spacecraft and their mechanisms. As the incoming vehicle approaches its target, precise sensors monitor the relative position of the two spacecraft. Once the incoming vehicle reaches the correct position beside the target, hardware on each spacecraft connects and locks together. When it is time for the spacecraft to undock, the mechanism releases.
Two spacecraft cannot dock if their docking hardware is not compatible. Soyuz spacecraft and other older vehicles use a probe-and-drogue docking mechanism. In this arrangement, the actively docking vehicle has an outward-pointing mechanism (probe), and the receiving vehicle has an inward-pointing mechanism (drogue). Other spacecraft use symmetrical docking systems in which each spacecraft has the same hardware. The recently developed International Docking System Standard (IDSS) describes technical specifications for docking systems. All spacecraft built with these specifications are able to dock with each other. Compatible spacecraft make international collaboration projects cheaper and more efficient because different vehicles do not need to be modified to fit together.
Today, docking is used most frequently to connect supply vehicles with space stations. Cargo vehicles often dock autonomously—that is, without being steered by a human. Vehicles carrying a crew may be piloted. Astronauts on the ISS can use the space station’s robotic arm to grab a resupply craft and pull it into place. This procedure is sometimes called berthing.
A small satellite in Earth orbit may rendezvous with another satellite to repair or refuel it. Such procedures help equipment last longer.
Interplanetary travel
Transfer orbits.
Spacecraft sometimes change their orbit to follow a different path around the object they are orbiting. For example, a satellite orbiting Earth may move from a close orbit to a more distant one. While the satellite follows a path from its original orbit to its new orbit, it is still traveling in orbit around Earth. It is following a temporary orbit whose path intersects with its original orbit and with its new orbit. A spacecraft’s path from one orbit to another is called a transfer orbit.
Transfer orbits take spacecraft from one planet to another. A spacecraft that travels from Earth to Mars moves from Earth’s orbit around the sun to Mars’s orbit around the sun. When the spacecraft is sitting on the launch pad, it is following Earth’s orbit. At launch, its path around the sun diverges from Earth’s. When the spacecraft’s new orbit intersects with Mars’s orbit, it uses rockets to match the velocity of Mars and join the planet on its orbit.
Interplanetary missions often follow a Hohmann transfer orbit. In this kind of orbit, the orbit’s perihelion (closest point to the sun) lies along the path of one planet, and its aphelion (farthest point from the sun) lies along the path of the other planet. To use the least amount of energy, a spacecraft must launch when the planets are correctly arranged. Mission planners may have to wait months or years for the best launch window for each planet. For this reason, missions with the same target often launch around the same time.
Flybys.
Flyby is a general term for when a spacecraft passes by a planet, moon, or other object. A flyby may be a spacecraft’s primary mission, or it may be part of a longer journey. Mission planners often time flybys for favorable times for gathering data. A flyby may also be planned to accelerate or decelerate a spacecraft. Such a maneuver is called a gravity assist.
Gravity assists.
A gravity assist is a method to alter a spacecraft’s trajectory without needing to consume significant amounts of fuel. Gravity assists depend on the laws of momentum to increase or decrease a spacecraft’s speed, or change its path. Momentum is a measure of the motion of an object. The momentum of a moving object equals its mass (quantity of matter) multiplied by its velocity (speed in a given direction).
To accelerate, a spacecraft approaches a planet in the same direction as the planet’s orbit around the sun. It approaches closely enough for the planet’s gravity to alter its trajectory. As the planet travels in its orbit, the spacecraft “slingshots” around it. The spacecraft leaves the encounter with a greater velocity. (To decelerate, the spacecraft approaches the planet from the opposite direction.)
Gravity assists work because as the planet’s gravity pulls on the spacecraft, the spacecraft’s gravity also pulls on the planet. This “steals” a tiny bit of the planet’s momentum. The loss of momentum is too tiny to have an effect on the planet. But because the planet is enormous and the spacecraft is small, this little bit of momentum can significantly boost (or decrease) the spacecraft’s velocity relative to the sun.
Slowing down.
Usually, spacecraft slow down by firing a rocket backward. But a spacecraft on an interplanetary trajectory may be able to decelerate by using a gravity assist.
Spacecraft can also slow down by aerobraking. In this maneuver, a spacecraft steers into a planet’s atmosphere. The increased air resistance slows down the spacecraft. Aerobraking is usually the first step in descending to the surface. It can also be used to adjust a satellite’s orbit.
Entry, descent, and landing
Landing safely requires a spacecraft to significantly decrease its speed. Careful deceleration is especially important for spacecraft returning humans to Earth. Unlike robotic probes, spacecraft crew and passengers cannot endure extreme acceleration or smash into the ground at great speed.
To leave orbit, a spacecraft uses small rockets to redirect its flight path into the upper atmosphere. This action is called de-orbiting. When the spacecraft enters the atmosphere, air resistance slows its descent. Thicker atmospheres provide more air resistance. Spacecraft landing on a body with little or no atmosphere cannot rely on air resistance. They brake using rockets.
A spacecraft descending through an atmosphere needs protection from heat. At the high speeds associated with reentry, air molecules pile up in front of the spacecraft and become tightly compressed. This compression heats the air to extreme temperatures. In Earth’s atmosphere, reentry temperatures exceed 10,000 °F (5,500 °C). Spacecraft are often protected by ablative shields that absorb heat by burning off, layer by layer. Reusable launch vehicles may be protected by a thermal protection system (TPS). A TPS includes heat-resistant ceramic tiles, insulated thermal blankets, and a composite material called reinforced carbon-carbon.
After air resistance slows a spacecraft, it falls freely through the air. Parachutes usually deploy to slow the spacecraft further. During descent, astronauts feel forces that are much higher than what most people experience in everyday life. Astronauts returning to Earth from the ISS feel around 4 g’s (four times the acceleration due to gravity felt on Earth).
A hard, fast landing can seriously injure astronauts. Crewed spacecraft use a long controlled descent to slow the spacecraft before landing. The earliest human space capsules returned to Earth in a ballistic reentry. This kind of descent is much steeper and shorter. Astronauts have compared ballistic reentry to being in a car crash. Today, astronauts use ballistic reentry only in emergencies.
Some crew capsules land in the ocean to take advantage of the water’s cushioning. NASA’s Mercury and Apollo crew capsules landed in the ocean. These and other early spacecraft were capable of only ballistic reentry, making it especially important to reduce the forces of impact on the crew.
Some spacecraft in use today, such as Russia’s Soyuz and China’s Shenzhou, land on the ground. Rockets fire just before touchdown to lessen the impact. Shock-absorbing seats protect the crew. After landing or ocean “splashdown,” rescue teams help astronauts exit the spacecraft safely.
The space shuttle used its wings to glide to a runway and land like an airplane. Vehicles with this design are called spaceplanes. This method of landing reduces the forces experienced by the crew to about 1 1⁄2 g’s.
Conditions that delay a launch can also delay a landing. Departure from a space station may be delayed by weather conditions at the planned recovery site. For water landings, high wind and waves can be especially hazardous.
Kinds of spacecraft
Spacecraft are designed variously for specific uses and operating conditions. This section describes certain types of spacecraft.
Artificial satellites.
Any human-made object that continuously orbits Earth or another body in space is an artificial satellite. This broad category includes diverse spacecraft, from lightweight lunar probes to the International Space Station. Thus, spacecraft are usually categorized more specifically based on their mission. People often use the term artificial satellite to refer to small craft that monitor weather, take atmospheric measurements, facilitate navigation and communication, or otherwise support human activity from Earth orbit. For more information, see Satellite, Artificial.
Reusable spacecraft.
Spacecraft are extremely expensive to build, test, and launch. But until recently, most spacecraft and rockets were used only once. To make spaceflight cheaper and more efficient, engineers developed spacecraft that can be launched multiple times. Reusable rockets are also known as reusable launch vehicles (RLV’s). Rockets built for only one use are called expendable launch vehicles (ELV’s).
Rockets
are used to launch every kind of spacecraft. Rockets may also be used on their own for inexpensive scientific missions. Sounding rockets carry small payloads into space for a few minutes at a time.
Rovers
are vehicles that can maneuver across the surface of a moon, planet, or other body. Astronauts have driven rovers on the moon. Most rovers are robotic space probes.
Space probes
can explore the solar system’s harshest environments and most distant destinations. A probe is a robotic vehicle with no astronauts on board. Unlike crewed spacecraft, probes do not need to carry food or water, or maintain an interior environment to keep humans alive. Therefore, space probes are constructed in diverse sizes and configurations.
Probes collect various kinds of data. They typically make observations of temperature and radiation. Probes equipped with cameras can take photographs and videos. Many probes are equipped with spectrometers. A spectrometer is an instrument that analyzes light waves to identify a substance or determine its chemical composition. Probes may perform experiments on their surroundings, such as digging into surface dirt. Some probes may expose material from Earth to the conditions of space so that scientists can observe the effects. A probe’s motion reveals information about the conditions that surround it. Changes in its course and speed can provide information about atmospheric density and gravity fields.
Probes designed to land on their targets are called landers. Hard landers have cushioned instruments that can survive the impact of a hard landing. Soft landers touch down gently. Impact vehicles make no attempt to slow down as they approach the target. Penetrators ram deeply into the surface of a target. Orbital probes, also called orbiters, remain in orbit around their target.
Space stations
provide an environment where people can live and work in space for prolonged periods. Space stations are larger than most other spacecraft. They provide more amenities and offer more comfortable living conditions. Spacecraft transfer people to and from the station and restock the station with food, water, equipment, and medical supplies.
A space station has one or more docking ports to receive visiting spacecraft. Most docking ports consist of a rimmed doorway called a hatch. The docking port connects with a hatch on the visiting spacecraft to form an airtight seal. When the two hatches open, they form a pressurized tunnel between the station and the visiting spacecraft, which crew members can safely pass through.
Small space stations can be built on Earth and launched into orbit by large rockets. Large stations are assembled in space. Rockets carry modules (sections) of the station into orbit, where astronauts assemble them. Old modules can be replaced, and new modules can be added to expand the station.
During a space mission, astronauts may live in space for a few days to a few months. Some astronauts have lived in space for a year at a time. Today, long-term missions take place on space stations.
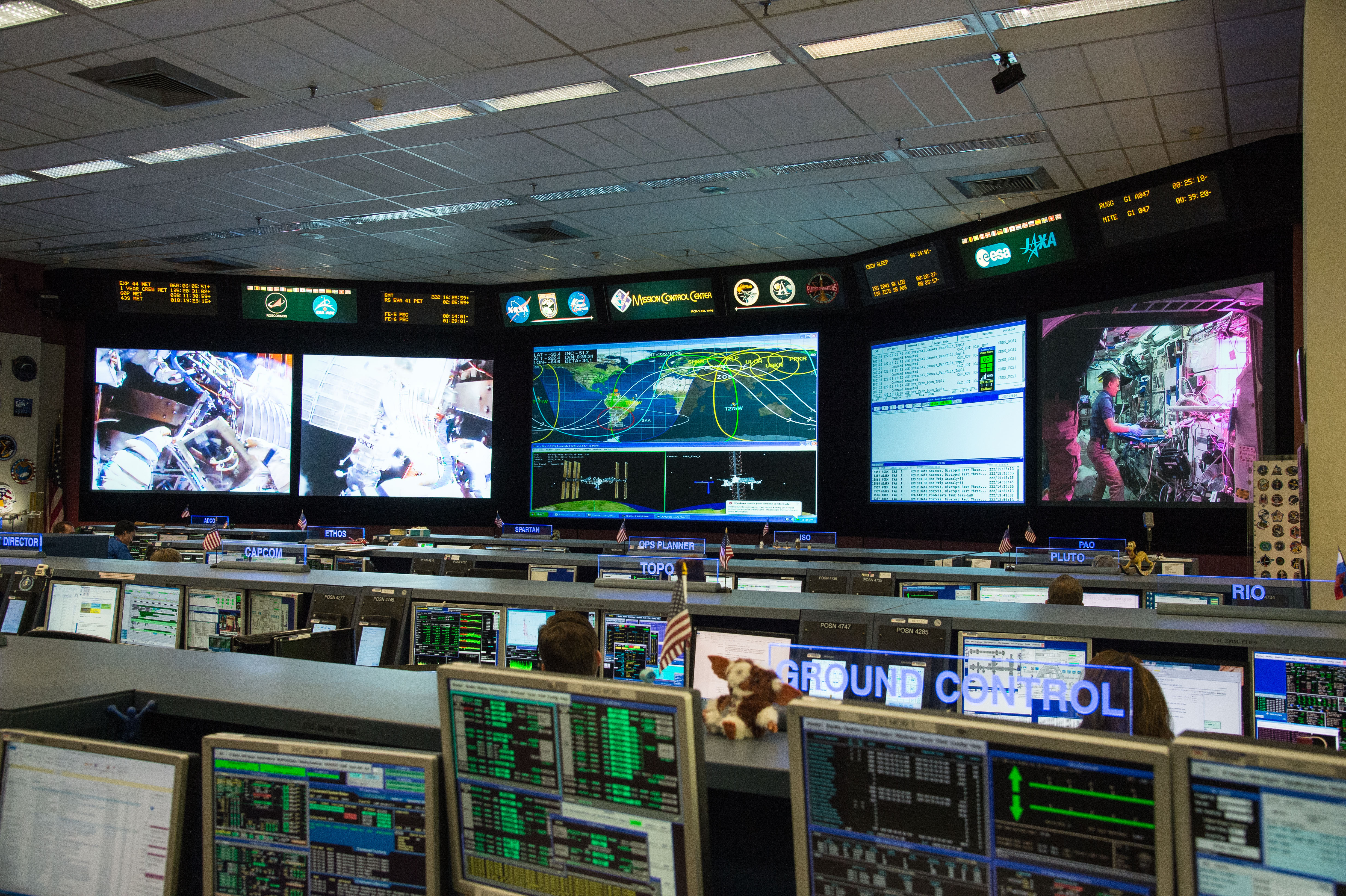
Living in space
Protection against the dangers of space
Conditions in space
differ significantly from the environment on Earth. Space has no air, and temperatures reach extremes of heat and cold. The sun and other sources produce dangerous radiation. Dust particles called micrometeoroids can damage vehicles. On Earth, the atmosphere serves as a natural shield against these threats. But in space, astronauts and equipment need other forms of protection.
Spacecraft usually have double hulls for protection against micrometeoroid impacts. A particle striking the outer hull disintegrates without damaging the inner hull. But orbiting debris left over from previous missions, called space debris, is of grave concern to space agencies. Although objects in Earth orbit are scattered far apart, they travel at high speeds. Because of their high velocity, even small objects can seriously damage a spacecraft.
Astronauts are protected from radiation in a number of ways. Missions in Earth orbit remain in naturally protected regions, such as Earth’s magnetic field. Filters installed on spacecraft windows protect the astronauts from ultraviolet rays. Scientists who monitor space conditions can alert crews if dangerous conditions arise. NASA keeps track of how much radiation astronauts have been exposed to throughout their career. Astronauts in danger of exceeding safe amounts of lifetime radiation are not assigned to long-duration missions.
A thermal control system regulates the temperature on a spacecraft. Electrical devices and the crew’s bodies give off heat and warm the cabin of the spacecraft. The thermal control system pumps fluids warmed by the cabin environment into radiator panels. These panels discharge the excess heat into space. The cooled fluids are pumped back into coils in the cabin.
Weightlessness.
In orbit, a spacecraft and its passengers continuously fall toward Earth. But a spacecraft in orbit travels forward at such high speed that as it falls downward, the planet’s surface curves away beneath it. Because the fall is constant, astronauts and objects aboard the spacecraft appear to weigh nothing. This condition is called weightlessness or microgravity.
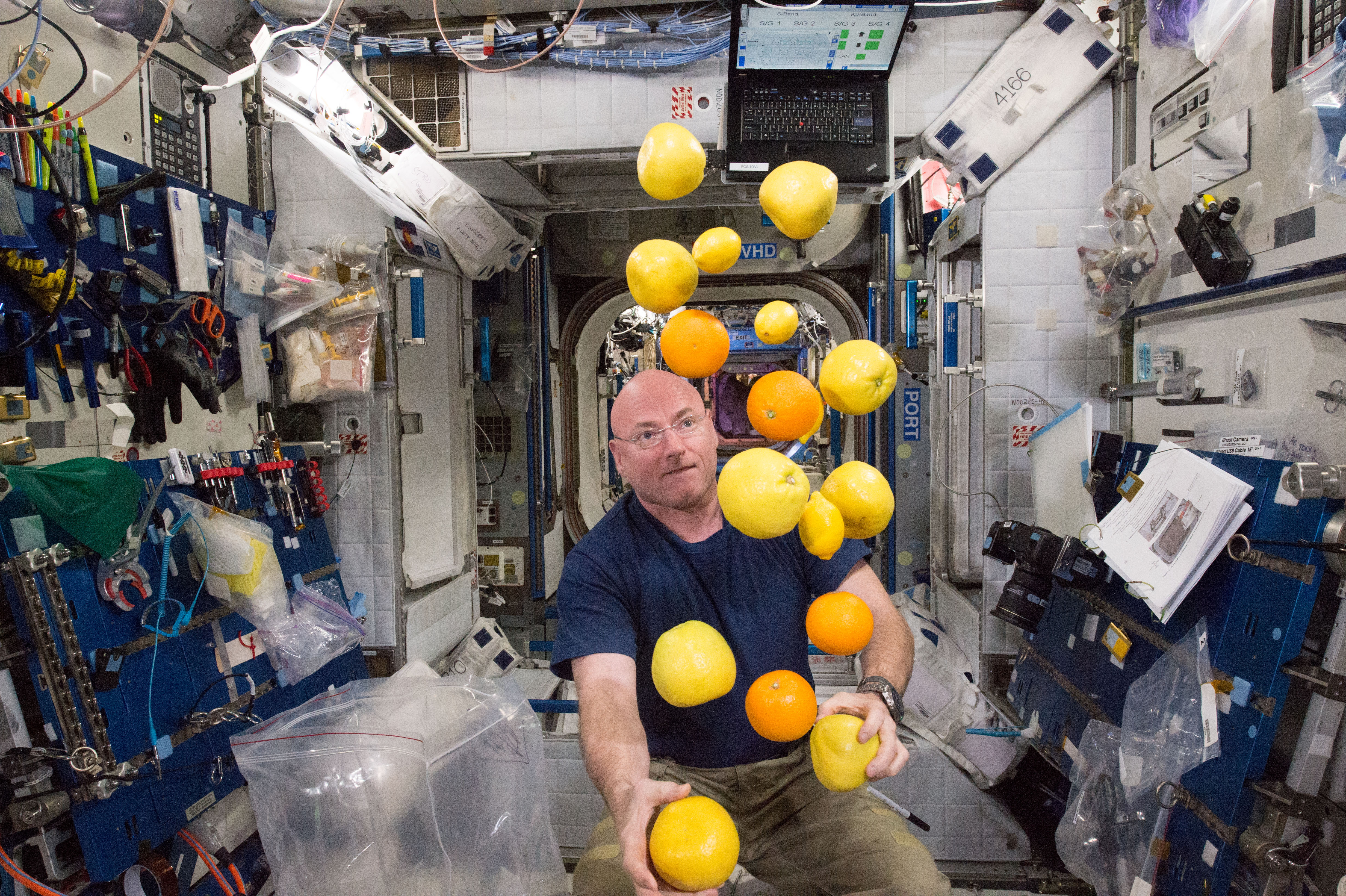
People sometimes call weightlessness “zero gravity.” Seeing videos of astronauts in weightlessness may lead people to believe there is no gravity in space, but this is incorrect. The force of gravity in orbit is almost as strong as it is on Earth. In fact, weightlessness can also be experienced on Earth. Roller coasters, descending elevators, and airplane flights can all produce the feeling of weightlessness.
Equipment behaves differently in microgravity than it does on Earth. Equipment and technology must therefore be designed particularly for use in space. For example, fuel does not drain from tanks in microgravity, so it must be squeezed out by high-pressure gas. Hot air does not rise in microgravity, so air circulation must be driven by fans. Particles of dust and droplets of water float throughout a spacecraft and only settle in filters on the fans.
The human body reacts to weightlessness in a number of ways. In the first several days of a mission, about half of all space travelers suffer from persistent nausea. Most experts believe that space sickness, also called space adaptation syndrome, is the body’s reaction to feeling weightless. Drugs to prevent motion sickness can provide relief from nausea. The condition generally passes in a few days.
Weightlessness also confuses an astronaut’s vestibular system (organs of balance in the inner ear). This effect makes it difficult to sense differences in direction. After a few days in space, the vestibular system disregards all directional signals. Soon after an astronaut returns to Earth, the organs of balance begin working normally again.
Over a period of days or weeks in weightlessness, an astronaut’s body experiences deconditioning. In this process, muscles grow weak from lack of use. Frequent strenuous exercise helps prevent deconditioning. Astronauts ride exercise bikes, use treadmills, and perform other types of physical activity.
After many months in space, a process called demineralization weakens the bones. Physicians believe that demineralization results from the absence of stress on the bones in a weightless environment. Vigorous exercise and a nutritious diet can minimize demineralization.
Meeting basic needs in space
The most important mission of a space station is to keep its crew alive. In space, expertly engineered equipment is required to fulfill even the most basic needs.
Breathable air.
Space stations maintain a mixture of oxygen and nitrogen similar to Earth’s atmosphere at sea level. Air is circulated through a series of filters to remove odors, contaminants, and carbon dioxide. Dehumidifiers remove moisture from the air and feed it into the station’s water purification system.
Eating and drinking.
The food on a spacecraft must be nutritious, easy to prepare, and convenient to store. Early astronauts ate freeze-dried foods—that is, frozen foods with the water removed. To eat, the astronauts simply mixed water into the food.
Loading the player...Washing hair in space
Today, astronauts enjoy similar freeze-dried foods along with ready-to-eat meals that more closely resemble convenience foods on Earth. Substitutions are sometimes necessary. Astronauts use tortillas to make sandwiches instead of sliced bread, which is too crumbly to eat in weightlessness.
Astronauts eat food out of the packaging or spread it on a base. Plates are not useful without the force of gravity weighing down the food. Beverages are usually packaged in pouches with straws, resembling sport drink pouches.
Astronauts can also grow vegetables on space stations. Fresh produce is a nutritious addition to astronauts’ diets. But produce is grown on space stations only in small amounts and is not a major food source. Resupply missions bring fresh fruits and vegetables from Earth.
On long missions, water must be recycled and reused as much as possible. Sweat, urine, water dirtied from washing, and water collected from the air are fed through purification systems to produce safe drinking water. Almost all wastewater on the ISS is recycled through the station’s water recovery system. Resupply vehicles deliver more water when it is needed.
Using the toilet.
Spacecraft do not have running water, so standard flush toilets are unavailable. Urine is captured in a funnel connected to the toilet’s collection tank. After use, astronauts clean the funnel with a sanitizing wipe. Solid waste is collected in plastic bags in a separate tank. While working outside the spacecraft, astronauts wear extra-absorbent diapers.
Hygiene.
Astronauts wash themselves with water or a liquid cleaning solution. They use towels to wash and dry. To wash hair, astronauts use a “no-rinse” shampoo.
Loading the player...Using the toilet in space
Brushing teeth in space is similar to brushing teeth on Earth. After brushing, most astronauts swallow their toothpaste.
Astronauts trim their own hair or give each other haircuts. Astronauts must shave and cut hair carefully to contain hair clippings. Small pieces of hair could easily contaminate the space station’s air and be inhaled or clog air filters. Usually, hair is vacuumed up as it is cut. When shaving, astronauts may use shaving cream to contain hair clippings.
Exercise.
Astronauts on long-duration missions exercise regularly. Space stations have exercise equipment such as treadmills and weight-training machines. Astronauts wear straps and backpacklike harnesses to stay anchored to the equipment.
Sleep.
Astronauts can sleep in sleeping bags with straps that press them to the soft surface and to a pillow. Most astronauts prefer to sleep floating in the air, with only a few straps to keep them from bouncing around the cabin. Astronauts may wear blindfolds to block the sunlight that streams in the windows periodically during orbit.
Recreation
on long space missions is important to the mental health of the astronauts. Many astronauts enjoy looking out spacecraft windows or photographing Earth from space. Astronauts also have access to several of the activities they enjoy on Earth. They may read books, listen to music, use the internet, and play video games. Exercise also provides relaxation.
Daily life in space
Space agencies’ mission control centers create daily schedules for astronauts. Flight directors and other mission control staff supervise the activities of astronauts aboard a space station. They schedule start and end times for equipment upgrades, maintenance tasks, scientific experiments, meals, exercise, free time, and sleep. A major task, such as work outside a spacecraft, may take up most of the day.
Construction and maintenance.
When a new space station needs to be assembled, or a new module needs to be added to an existing space station, astronauts serve as construction workers in space. Crews connect new sections to existing sections. They set up antennas and solar panels and attach power and air connectors. They also install and replace equipment inside the space station.
Working outside a spacecraft.
Some tasks, such as repairs, require astronauts to work outside the space station. Working outside a vehicle in space is called “spacewalking” or extravehicular activity (EVA). To prepare for EVA, astronauts put on space suits and move to a two-doored chamber called an air lock. They then release the air from the air lock, open the outer hatch, and leave the spacecraft. When the astronauts return, they close the outer door and let air into the air lock. Then they open the inner door into the rest of the spacecraft, where they remove their space suits.
A space suit is made from layers of flexible, airtight materials. Tight seals connect the pieces of the space suit. Equipment in a backpack provides oxygen and removes carbon dioxide and moisture. A radio enables the astronaut to communicate with other crew members and ground control. The helmet must allow good visibility while at the same time blocking harmful solar radiation. Gloves are a crucial part of the space suit. They must be thin and flexible enough for the astronaut to feel small objects and to handle tools.
Controlling inventory.
Keeping track of thousands of items aboard a space station is a major challenge. Some items are stored in lockers or briefcases. Other items are strapped to the walls. Small objects sent to the ISS often have dots of Velcro on them. The objects can adhere to corresponding Velcro patches on walls, work surfaces, and astronauts’ clothing.
Permanent space stations easily become cluttered and messy. Equipment, tools, and even pieces of food drift away and become lost. Objects that have been missing for months may unexpectedly drift into view.
Space station chores.
Astronauts regularly take out the trash. On long-duration missions, trash is collected in small trash bags. When these small bags are full, they are stashed in a bigger trash bag, usually kept in a storage area. Astronauts pack large trash bags into a vehicle that is ready to leave the space station. The bags are usually stored in a part of the vehicle that will be destroyed on reentry. Loading the player...
Cleaning up a mess in weightlessness
Vacuuming is an important chore on a space station. In weightlessness, dust does not settle on surfaces. It floats in the air and collects in air circulation equipment. Vacuuming helps maintain clean air throughout the station. On the ISS, astronauts have a vacuum cleaner that is worn like a backpack.
Astronauts do not do laundry. They wear clothes until they are dirty, then throw them away. Washing clothes would consume too much water. However, future long-term missions may require astronauts to wash clothes. Commercial companies are currently investigating how laundry detergent can be improved for use in microgravity.
Scientific research.
Astronauts experiment with the effects of weightlessness on various materials, plants, animals, and themselves. Many experiments aim to find ways to keep astronauts safer and healthier during space missions. Astronauts also test manufacturing techniques and other industrial processes that could someday be used in orbit.
Emergencies.
Astronauts train for emergencies often. Long-term missions include regular drills. Astronauts practice what to do when there is a fire, a toxic substance in the air, or a depressurization risk (a risk that the station’s air is leaking into space).
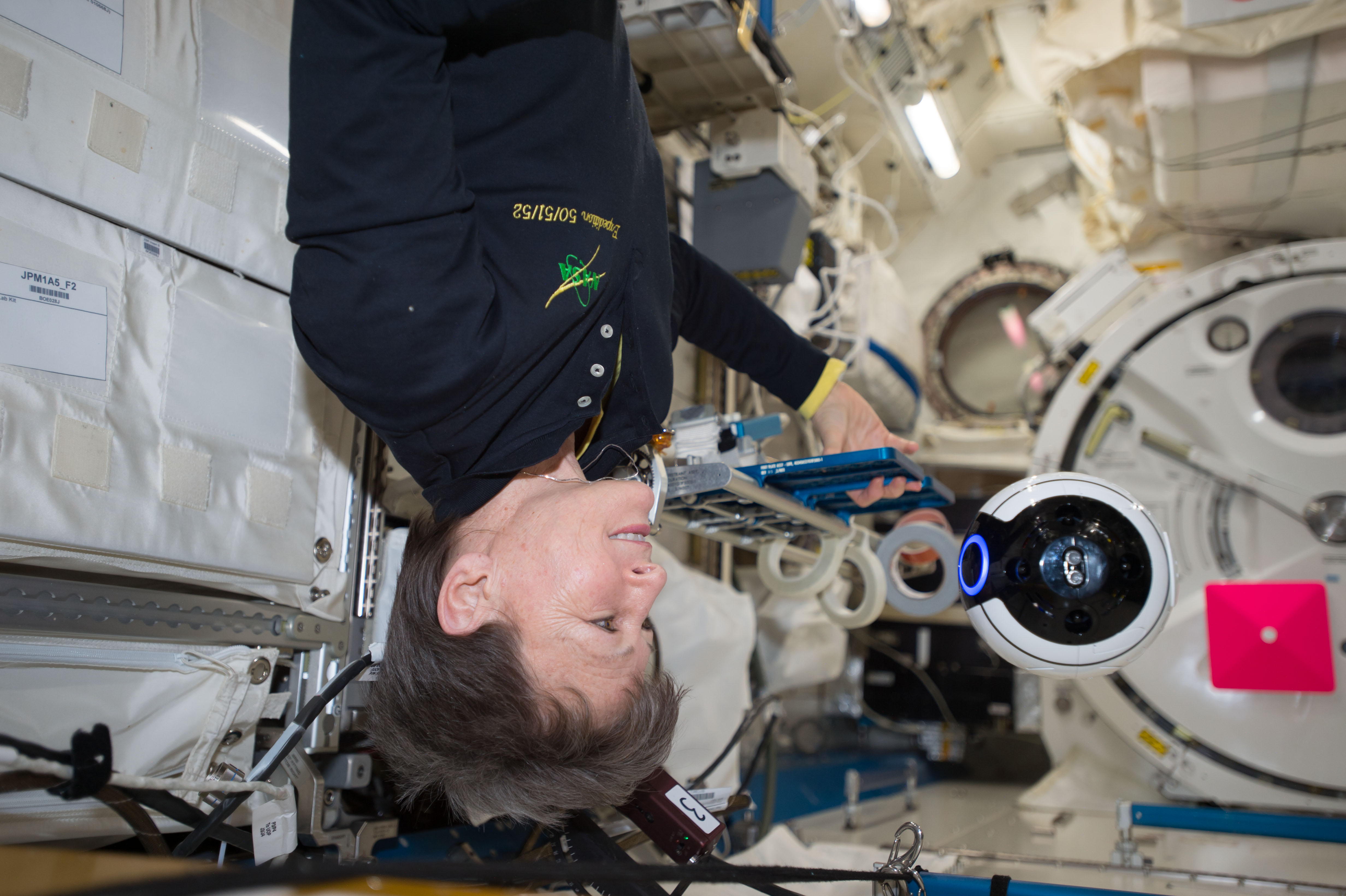
A space station has at least one vehicle docked at all times. If the crew needs to evacuate, they can use the vehicle as a “lifeboat” to return to Earth. Procedures change depending on how many astronauts are on the station and where escape vehicles are docked.
The smoke detectors on the ISS, situated in the air circulation system, can trigger a fire alarm. In the event of a fire, astronauts shut off ventilation to prevent smoke and fire from spreading throughout the station. They also turn off power in the affected area to minimize damage to electrical systems. They use fire extinguishers to put out the fire. Space agencies work to minimize the risk of fire by selecting nonflammable materials for mission equipment.
Astronauts also prepare for medical emergencies. Space stations are equipped with first-aid supplies, medications, a few medical devices, and instruction manuals for medical procedures. Before a mission, astronauts learn how to perform basic and advanced first- aid procedures, such as stitching a wound closed. In nonurgent situations, astronauts can contact doctors on Earth with their medical concerns.
Exploring our solar system
No two objects in the solar system are exactly the same. Unique discoveries can be made on each planet, moon, asteroid, or comet. Space exploration programs have overcome formidable technical challenges to send probes to these destinations. Some challenges have not yet been overcome.
Exploring Earth’s moon
Earth’s moon is the most famous destination explored by spacecraft. Its nearness to Earth has made it the target of many ambitious missions. The moon has been visited by spacecraft from many different countries. NASA’s crewed Apollo missions to the moon have become the most well-known space missions in history.
The moon’s close relationship with Earth makes it a good target for scientific study. Because the moon has no weather, scientific equipment there can last for a long time. Laser reflectors from NASA’s Apollo program and from the Soviet Union’s Lunokhod program remain intact on the surface. Scientists still use these reflectors to take measurements today.
Space agencies study the hazards of moon exploration to make future moon missions safer. Some doctors are concerned that inhaling regolith (gritty moon dust) could damage astronauts’ lungs. Regolith can also damage equipment, especially when a rocket launch blasts dust in all directions. In addition, the moon’s weak magnetic field and lack of atmosphere offer little protection from radiation and solar activity. With better solar activity forecasts, mission planners could schedule EVA’s during periods of low radiation. But astronauts on long-term trips to the moon would be exposed to unsafe amounts of radiation over time. Some people have suggested that astronauts should live in underground bases or even in the moon’s natural underground lava tubes.
Early lunar probes.
The first lunar probes were launched in 1959. These missions included Soviet Luna probes and NASA’s Pioneer 4. The Soviet Union began to test lunar hard landers in 1963. After many failures, they succeeded with Luna 9, launched in 1966. NASA’s Surveyor program made a series of successful soft landings beginning in 1966.
Also beginning in 1966, the United States sent five probes in the Lunar Orbiter series to photograph the moon’s surface. The Lunar Orbiters revealed the existence of irregular “bumps” of gravity in the moon’s gravitational field caused by dense material buried beneath the lunar surface. These areas of tightly packed matter were called mascons, short for mass concentrations. Knowledge provided by these probes helped NASA prepare for the crewed Apollo missions.
In 1970 and 1973, the Soviet Union sent remotely controlled rovers to the lunar surface. The rovers, Lunokhod 1 and 2, were advanced for the time. Their design resembled a large bucket with a lid, on wheels. The rovers conducted soil experiments and took other measurements on the surface. In total, the rovers traveled 32 miles (52 kilometers) and transmitted over 100,000 images of the lunar surface.
Soviet probes also returned lunar samples to Earth in the 1970’s. Over three missions, Luna landers obtained about 326 grams (11 1⁄2 ounces) of material.
The Apollo program.
NASA’s Apollo program was a series of missions to take astronauts to the surface of the moon in the late 1960’s and early 1970’s. Crewed and uncrewed Apollo missions tested spacecraft and other technology in Earth orbit. Apollo 8 was the first mission to carry astronauts into orbit around the moon. In total, 12 Apollo astronauts walked on the surface of the moon.
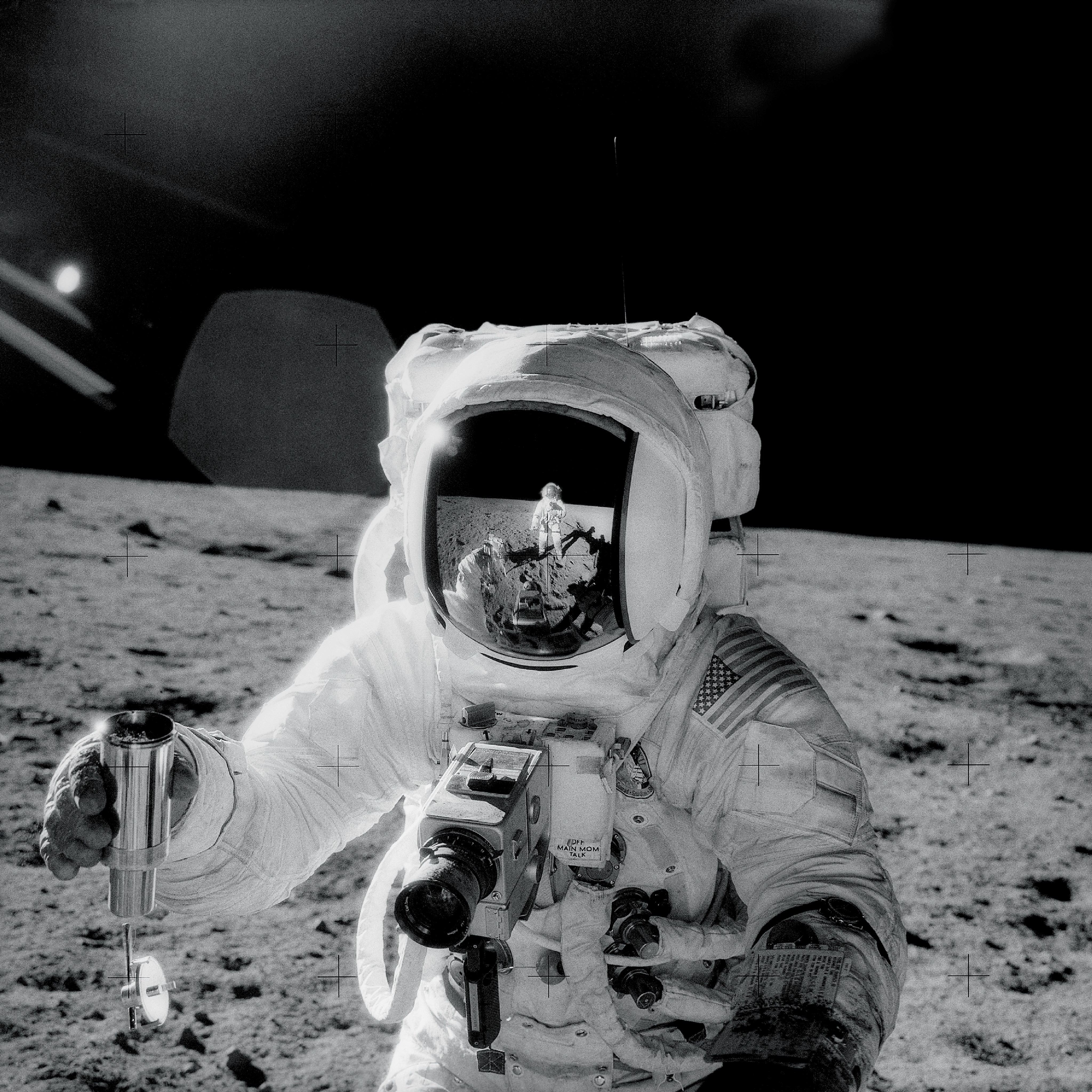
The major accomplishment of the Apollo program was overcoming the technological and engineering challenges involved in transporting human beings to the moon and back. Traditional pilot controls designed for steering airplanes would not be sufficient for maneuvering the Apollo spacecraft. Engineers developed innovative computer systems for guidance, navigation, and flight control. New, powerful rockets were built.
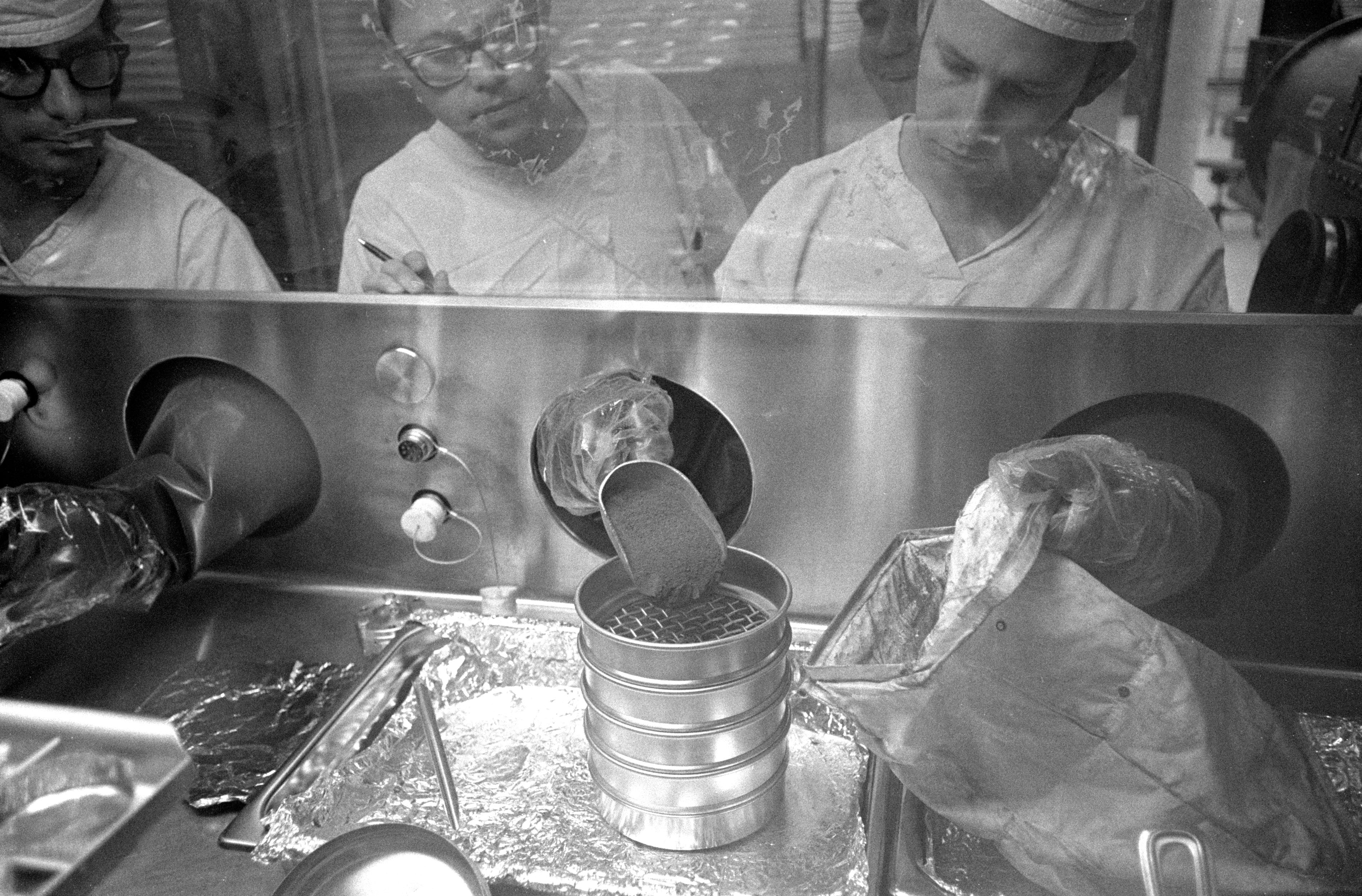
Apollo astronauts took many photographs and recorded other observations of the moon and Earth. They conducted EVA’s and scientific experiments on the surface of the moon. They set up seismometers to measure tremors (earthquakes), sometimes called moonquakes. Sensitive laser reflectors precisely determined the distance between the moon and Earth. Astronauts also collected samples. Apollo 11 was the first mission to bring samples from a different planetary body back to Earth. In total, Apollo astronauts collected 842 pounds (382 kilograms) of lunar samples.
During EVA’s, astronauts drove the electric Lunar Roving Vehicle (LRV). Driving on the moon’s surface, rather than walking, let astronauts explore a greater area and collect more samples. The LRV had some familiar features, such as seats with seatbelts, but was mostly unlike the cars driven on Earth. It was specially designed to work in low gravity and extreme temperatures. Its wheels were metal and it was steered using a T-shaped stick control. The LRV was folded up for transport to the moon. On the lunar surface, astronauts carefully unpacked the LRV. Then it unfolded mostly automatically.
Modern lunar probes.
Since the Apollo program, probes from many countries have reached the moon. Some of these probes were designed to study the moon with advanced modern instruments. Others were tests of technology developed in countries whose space agencies were relatively new.
In the 1990’s and 2000’s, several space agencies launched missions to study the moon’s surface and gather information about its composition. These missions include NASA’s Clementine and Lunar Prospector, ESA’s SMART-1 spacecraft, JAXA’s SELENE orbiter, China’s early Chang’e missions, and ISRO’s Chandrayaan-1 mission. Several of these missions found evidence of water ice near the moon’s poles.
NASA sent two missions to the moon in 2009. The Lunar Reconnaissance Orbiter (LRO) and the Lunar Crater Observation and Sensing Satellite (LCROSS) were launched on the same rocket but carried out separate missions. A section of the launch rocket was crashed into the lunar surface near the south pole. LCROSS then flew through the debris kicked up by the impact. Its sensors confirmed the presence of water ice in the debris cloud. LRO entered the moon’s orbit and mapped its surface and environment.
In the 2010’s and 2020’s, China and India advanced their lunar exploration technology. In 2013, China’s Chang’e 3 landed on the moon carrying a rover called Yutu. Yutu explored the moon for 31 months. In 2019, Chang’e 4 became the first spacecraft to touch down on and explore the side of the moon that cannot be seen from Earth. A satellite relays data from the lander to Earth. In 2020, Chang’e 5 returned lunar samples to Earth. In 2023, ISRO’s Chandrayaan-3 became the first mission to land near the moon’s south pole. Chandrayaan-3’s lander, called Vikram, deployed a rover called Pragyan.
Exploring the sun
The sun has an immense influence on the rest of the solar system. Changes in the sun’s magnetic field and radiation can have consequences for humans on Earth. Exploring the sun helps scientists understand this solar weather, and how it affects human technology and Earth’s environment. Studying the sun can also help astrophysicists understand stars in general. No other star can be studied as closely as the sun. Loading the player...
Parker Solar Probe Heat Protection
Solar events can disrupt radio communications and overload electric power systems. They could seriously harm astronauts outside the protection of Earth’s magnetic field. Such events are difficult to predict. Eventually, scientists hope to be able to predict these events consistently and reliably.
In the 1970’s, NASA astronauts used the Apollo Telescope Mount (ATM) to photograph the sun. The ATM was a solar telescope built into the Skylab space station. It was the first observatory to be operated by humans in orbit.
Solar probes have studied the sun from different vantage points. The Ulysses probe, developed by ESA and NASA, mapped the sun from an orbit that passed close to its north and south poles. NASA’s STEREO mission, a pair of orbital probes, monitored the sun from opposite sides at once. NASA’s Parker Solar Probe travels extremely fast and approaches the sun closely enough to enter the corona (outer atmosphere). Solar Orbiter, a probe developed by ESA with NASA collaboration, is able to study the sun over a wide range of latitudes. For information about more solar probes, see Sun (History of modern solar study).
Most solar probes study solar weather. Probes may carry instruments to study the solar wind, particles flowing continuously from the sun. They may study variations in the sun’s magnetic field, radiation, and temperature.
Any probe that approaches the sun closely will melt without protection against the sun’s extreme heat. Spacecraft must have durable heat shields. Instruments that operate outside the heat shield are made from metals with extremely high melting points. However, most solar probes do not closely approach the sun.
Exploring the inner planets
Mercury.
Very few probes have explored Mercury. No probe has landed safely on its surface. Scientists hope that technological improvements and lower launch costs will soon make more Mercury missions possible.
Scientists are interested in studying Mercury’s composition, magnetic field, and extreme temperatures. They also want to learn more about the effects of its proximity to the sun. In addition, astronomers have discovered exoplanets that resemble Mercury. An exoplanet is a planet that orbits a star other than the sun. It is not possible to study these planets up close. But studying Mercury could help scientists better understand exoplanets and their characteristics.
Mercury’s proximity to the sun makes the planet a challenging target. Near the center of the solar system, powerful gravity accelerates spacecraft toward the sun. Without carefully planned trajectories, probes would zoom past Mercury at high speeds. A probe scheduled to fly by Mercury must fight the sun’s gravity with rockets to slow down enough to collect data. An orbiter must decelerate even more. It must travel slowly enough for Mercury’s weak gravity to capture it.
The first spacecraft to reach Mercury was NASA’s Mariner 10. It conducted flybys of the planet in 1974 and 1975. The spacecraft’s trajectory was meticulously calculated. Mariner 10 was the first mission to use a gravity assist. The maneuver slowed the probe down so that it would require less rocket power to decelerate. See Mariner.
NASA’s MESSENGER probe was the first spacecraft to orbit Mercury. It took seven years and five gravity assists for MESSENGER to successfully enter orbit. The probe mapped Mercury’s surface and studied its composition, interior structure, and magnetic field.
Landing on Mercury would be even more difficult than entering orbit. Mercury has practically no atmosphere, so a probe heading for the surface would not be slowed by air resistance. Parachutes would be useless. A spacecraft aiming to land on Mercury would need to drastically slow itself with rockets to prevent a disastrous impact. Currently, no Mercury landers are scheduled.
The next mission to Mercury, BepiColombo, is expected to enter orbit in late 2025. BepiColombo’s two spacecraft were designed to follow up on discoveries made by Mariner 10 and MESSENGER. BepiColombo is a collaboration between ESA and JAXA.
Venus
has the hottest planetary surface in the solar system and a crushingly thick atmosphere. But when the solar system was young, Venus and Earth were similar. Exploring Venus could reveal what caused the two neighboring planets to evolve differently.
Venus was the first planet to be explored by space probes. NASA’s Mariner 2, the first probe to visit Venus, detected the planet’s thick clouds in 1962 (see Mariner). From the 1960’s through the early 1980’s, Soviet Venera probes vastly expanded knowledge of the planet. Venera 3 released an impactor to the planet’s surface. Venera 4, 5, and 6 descended into the atmosphere and found that it was mostly carbon dioxide. Venera 7 and 8 managed to land on Venus and transmitted temperature and pressure data from the surface. Venera 9’s photographs of Venus were the first images captured on the surface of another planet.
Although numerous early space probes visited Venus, they gathered limited data. This is partly because robotic technology was less dependable than it is today. But the biggest limitation was the harsh environment on Venus. Traditional electronics do not perform well in the extreme heat and pressure. No lander has survived longer than about two hours. For future missions, NASA engineers are testing electronics made with unconventional materials that can withstand Venus’s environment. Other rover prototypes operate mechanically rather than electronically. Such rovers could be powered by wind and operate using gears and springs.
All Venus probes since the mid-1980’s have been designed to operate in orbit. In the 1990’s, NASA’s Magellan probe used radar to map the entire surface of Venus. ESA’s Venus Express, launched in 2006, orbited the planet for over eight years. Venus Express gathered data on atmospheric chemistry. JAXA’s Venus Climate Orbiter, known as Akatsuki, entered orbit in 2015. Akatsuki closely studies Venus’s unusual weather patterns.
In addition to learning how Venus evolved, planetary scientists are interested in its current geology. The planet’s surface has many volcanoes, but it is not known if any volcanoes are still active. Scientists are also interested in the planet’s thick, complex atmosphere.
Space agencies have planned upcoming missions to study the planet’s atmosphere, surface, and interior, and to learn how these three regions relate to one another. NASA is planning to launch the DAVINCI+ and VERITAS missions to Venus in the late 2020’s. DAVINCI+ is designed to collect data from the planet’s atmosphere and surface. VERITAS is a planned orbiter. ESA plans to launch the EnVision orbiter to Venus in the early 2030’s.
Exploring Mars
In the distant past, Mars likely had lakes and streams, and a more robust atmosphere. On Earth, these characteristics helped microscopic life arise billions of years ago. People have long wondered if life could also be found on Mars. Mars has chemical elements that are important for life, such as carbon, hydrogen, oxygen, and nitrogen. Scientists have found substances on Mars that are similar to substances produced by living organisms. But no one has yet found proof of Martian life.
Scientists study Mars to uncover the planet’s history. The appearance and mineral characteristics of the Martian landscape can reveal ancient geological processes and chemical reactions. Many Mars missions are equipped to seek out evidence of liquid water. A “wetter” Mars may have been able to support life. Some scientists think there is hidden liquid water on Mars today. But most people think liquid water was present on Mars only in the past.
Planetary scientists also study the atmosphere and climate of Mars. They monitor seasonal changes in the Martian weather and polar ice caps. They investigate how dust storms form and grow. Engineers use this information to design equipment for Mars missions. Probes that can withstand the planet’s weather and harsh temperatures last a long time.
The first journeys to Mars.
Space programs have been sending probes to Mars for over 50 years. The U.S. and Soviet space programs carried out the earliest Mars missions in the 1960’s. The technology for interplanetary spaceflight was new. These early missions mainly aimed to survive the journey to Mars. Many failed. In 1965, NASA’s Mariner 4 captured the first close-up photographs of Mars (see Mariner). Mariner 9 entered Mars orbit in 1971. It was the first probe to orbit a planet other than Earth. In the same year, the Soviet probe Mars 3 made the first soft landing on Mars.
Orbital probes.
Many spacecraft have orbited Mars to capture high-resolution images and other data from the planet’s surface. Images from orbiters are used to create detailed maps of the planet’s geological features. NASA’s Mars Global Surveyor circled the planet in a low-altitude orbit from 1999 to 2006. The orbiter documented daily weather on Mars, helping scientists plan maneuvers for other spacecraft in the atmosphere and on the surface.
NASA’s Mars Odyssey, NASA’s Mars Reconnaissance Orbiter, and ESA’s Mars Express continue analyzing the surface today. These orbiters have powerful cameras. Using current technology, scientists can use the probes to photograph surface features as small as 1 meter (39 inches) across.
Several active orbiters are dedicated to studying the Martian atmosphere. Scientists think that long ago, Mars had a thick atmosphere. A thick atmosphere may have kept liquid water from freezing. But the Martian atmosphere today is thin. NASA’s MAVEN orbiter studies the upper atmosphere to learn how air molecules escape Mars. MAVEN may show scientists how a thick atmosphere disappeared over time. The United Arab Emirates (UAE) Hope probe gathers data from all layers of the atmosphere to help scientists understand Martian weather and climate. The MAVEN and Hope science teams share their data with each other to gain a more complete understanding of the atmosphere. The ExoMars Trace Gas Orbiter searches the atmosphere for gases that are present only in small amounts. Such gases could be produced by geological or biological activity. The Trace Gas Orbiter is a collaboration between ESA and Roscosmos.
Surface probes.
Technology for surface probes has improved over the past few decades. The first Mars rover, Sojourner, arrived on the planet in 1997. Sojourner was roughly the size of a microwave oven. The probe’s primary mission was to find out whether a rover could traverse Mars at all (see Mars Pathfinder). Today’s Mars rovers are as large as cars and carry many kinds of advanced scientific equipment.
Landers and rovers study Martian terrain closely, with finely tuned instruments. They may analyze minerals or look for signs of water. Surface probes can study the structure and interior of Mars. They may also observe weather phenomena, such as dust storms, from within. NASA’s InSight lander collected a wealth of data on tremors, sometimes called marsquakes.
Landing on Mars is famously tricky. The thin atmosphere does not provide much air resistance. Parachutes help somewhat, but because parachutes depend on air resistance, they cannot slow a heavy spacecraft to a safe landing speed. Most spacecraft also fire rockets toward the ground to slow their descent. Small rovers may use bouncy airbags to cushion their landing. Rovers heavier than about 2,200 pounds (1,000 kilograms) are too large to rely on airbags. NASA’s car-sized Curiosity and Perseverance rovers landed using a “sky crane” system. In this system, the spacecraft containing the rover descends most of the way to the surface in a protective aeroshell. Before it hits the ground, rockets slow the spacecraft to a hoverlike descent. The spacecraft opens and cables gently lower the rover to the ground.
Martian dust storms create problems for probes. The wind does not reach perilous speeds. But the storms cover the landscape with gritty dust. This dust can settle onto landers and clog mechanical equipment. It can coat solar panels and block out the sunlight that provides power to a probe. Sometimes, remote operators can maneuver probes to disperse the dust. At other times, the problem corrects itself: wind can also blow dust off equipment. Too much dust can end a probe’s mission by cutting off its source of power. Probes can be designed with alternate sources of electricity, such as nuclear power. Such probes may not need solar panels at all. But alternate sources of electricity are usually significantly more expensive.
Much of what scientists know about Martian chemistry and geology comes from data collected by landers. NASA’s Viking mission, which included two landers, conducted experiments in the 1970’s to detect evidence of living organisms on Mars. The experiments did not find any evidence of life. But they helped scientists learn more about the planet’s chemical composition. The Viking landers also took the first soil samples and the first clear images on the surface of Mars. NASA’s Pathfinder, the lander that carried Sojourner, captured more high-quality images.
In 2008, NASA’s Phoenix lander found frozen water in the far north of Mars. Before the Phoenix mission, scientists had some evidence of water on Mars. They knew of geological features and minerals that were formed by water long ago. The orbiter Mars Odyssey’s spectrometer had detected hydrogen, suggesting water ice was concealed just beneath the surface. But Phoenix was the first probe to directly find water. It scooped soil from the surface and analyzed it in a mass spectrometer. The analysis confirmed the presence of water ice.
A rover can explore more area than a stationary lander can. But Mars rovers travel very slowly. Sojourner’s maximum speed was less than 1⁄2 inch (1 centimeter) per second. NASA’s newest rover, Perseverance, can travel as fast as 1 5⁄8 inches (4.2 centimeters) per second on a smooth surface. Someone walking at a medium pace on Earth would still be about 30 times faster than Perseverance. Traveling slowly helps rovers conserve power. They also move slowly because rocky terrain on Mars can easily damage a rover. Rovers can move faster today than in the past because engineers have learned to design stronger, sturdier wheels. Newer rovers are also designed to better resist tipping. Navcams (navigation cameras) help rovers automatically steer around obstacles. But if a rover encounters unexpectedly difficult terrain, mission directors may change the rover’s route to avoid risking damage.
In 2004, NASA’s Spirit and Opportunity rovers landed on Mars. Spirit and Opportunity carried the same sets of instruments, but they landed in different locations. These “twin” rovers found evidence that Mars once had large amounts of liquid water (see Mars Exploration Rover Mission). The Chinese rover Zhurong arrived in 2021 carrying several instruments to study the planet’s geology and atmosphere.
A few rovers study Mars today. Curiosity has been exploring the Martian surface since 2012. It is the first rover to collect Martian rock samples using a drill. See Mars Science Laboratory. Perseverance, which arrived on Mars in 2021, collects samples and stores them securely in sealed tubes. A future mission could collect the samples and return them to Earth. Perseverance also searches for evidence of microbial life. See Mars 2020.
Human exploration.
Sending astronauts to Mars would be much more difficult than sending probes. Human Mars explorers would need water, nutritious food, breathable air, protection from the environment, a vehicle to return to Earth, and propellant to power the return vehicle. If all these resources were sent to Mars from Earth, they would require an immense launch vehicle, or multiple trips, consuming huge amounts of fuel.
Many engineers work on creative ways to make human Mars missions more efficient. For example, robots could be sent to Mars to prepare a site for a human mission. The robots could use dirt and rocks on Mars to build a habitat.
Probes are already helping scientists and engineers plan for human missions to Mars. For example, Mars Odyssey has studied the radiation environment around Mars to determine how astronauts could best be protected. Other probes have tested technology that could support humans on Mars. An experimental device on Perseverance converted carbon dioxide from the Martian air into oxygen. Only small amounts of oxygen were produced. But a larger version of the device could someday produce breathable air for astronauts or fuel for rockets.
Moons of Mars.
Mars’s potato-shaped moons, Phobos and Deimos, have not historically been considered important targets for exploration. However, they have occasionally been visited by spacecraft. Mariner 9 captured the first close-up images of the moons. The Soviet probe Phobos 2 also photographed Phobos.
Exploring the gas giants
The gas giants, Jupiter and Saturn, resemble miniature solar systems. Each has a system of rings and is orbited by dozens of moons. The moons vary widely in size and characteristics. Some are rocky like Earth’s moon. Others have oceans or volcanoes. Most experts think moons of Jupiter or Saturn are the most likely places to find extraterrestrial life in our solar system.
Jupiter and Saturn were most likely the first planets to form in our solar system. But astrophysicists do not agree on how and where they formed and evolved. Studying them helps scientists create more accurate simulations of the solar system’s past. It could also help scientists learn about other star systems. Astronomers have discovered over 1,500 gas giants orbiting other stars. Many of these planets are found extremely close to their star—much closer than Jupiter and Saturn are to the sun. Scientists do not know why these gas giants orbit so closely. They may form close to their star, or they may form far away and migrate over time.
Since the 1970’s, probes have visited Jupiter and Saturn. The gas giants’ distance from the sun is important to consider when designing spacecraft. By the time a spacecraft reaches Jupiter, the sun is distant and its light is dim. Probes traveling to the outer solar system need gigantic solar panels to collect enough light to produce electricity. Otherwise, they need a different source of power, such as nuclear energy.
Intense radiation belts surrounding Jupiter put spacecraft in danger. Radiation can cause errors in software and seriously damage hardware. Mission planners steer probes around the most intense areas of radiation, but orbital probes cannot avoid them entirely. Such probes are built with materials that can withstand high amounts of radiation. They also may have external components to shield computer systems from radiation. Newer Jupiter probes protect their sensitive electronics equipment in a walled metal box called a vault.
Probes have studied the gas giants from flybys and in orbit. A probe to Jupiter or Saturn would not be able to land. Probes sent into the atmosphere fall through the clouds until heat and pressure destroy them. In fact, mission teams use these extreme conditions to dispose of old probes. Over time, probes run out of propellant and become damaged by radiation. An old, deteriorated probe might become uncontrollable and crash into a moon. To prevent this from happening, a probe to Jupiter or Saturn is destroyed when its mission ends. Scientists do not want to accidentally disturb a moon where life may be developing. If a future probe found signs of life, scientists would need to be sure that it was native life, not Earth microbes from a wrecked spacecraft.
Jupiter.
Jupiter’s size makes it a compelling target for study. The planet is so large that it influenced the solar system’s history. Its gravity affected how and where other planets could form. Many scientists think Jupiter changed its position as the solar system formed, but it is uncertain what path it may have followed. Scientists can measure Jupiter’s chemical composition to learn how the planet evolved.
Planetary scientists also study Jupiter’s thick, complex atmosphere to understand its unique weather. Stripes of clouds run around the whole planet. Huge storms tear through the atmosphere. Some of them last for hundreds of years. Cyclones swirl in a geometric arrangement around the planet’s north pole.
Scientists do not know whether Jupiter has a solid core. The center of the planet cannot be directly studied, but scientists can learn about Jupiter’s core by examining how its gravity affects the atmosphere. Fluids deep within Jupiter sustain its enormous magnetic field, so measurements of the magnetic field can also give scientists clues about the planet’s interior.
The first missions to Jupiter studied the planet only from flybys. NASA’s Pioneer probes visited Jupiter in the 1970’s. In 1979, NASA’s Voyager 1 spacecraft discovered Jupiter’s rings. Spacecraft bound for other destinations sometimes collect data during Jupiter gravity assists. NASA’s New Horizons probe took high-quality photographs of Jupiter during a gravity assist in 2007.
NASA’s Galileo mission surveyed Jupiter and its moons in more depth. Galileo had two parts. The large main spacecraft studied Jupiter and its moons from orbit. The atmospheric probe directly sampled the planet’s atmosphere. Both arrived at Jupiter in 1995. The mission gathered rich data on the planet’s atmosphere, magnetosphere, and extreme radiation environment.
Galileo also worked with the Cassini spacecraft on a special team mission. During Cassini’s Jupiter flyby in 2000, the two spacecraft took measurements at the same times from different positions. In one experiment, Galileo measured Jupiter’s magnetosphere from the inside while Cassini observed it from the outside. Cassini also photographed Jupiter, its rings, and several moons.
The most recent Jupiter mission, NASA’s Juno probe, reached Jupiter in 2016. Juno studies Jupiter’s structure, atmosphere, and magnetosphere. It is expected to operate through 2025.
Saturn
is known for its robust rings. Planetary scientists study Saturn’s rings as an example to learn how rings form, evolve, and gradually disappear. But Saturn’s rings may help scientists answer even bigger questions. Saturn has several small moons that orbit near or within the rings. The moons’ gravity shapes the rings by gathering millions of pieces of ice and rock into bands around the planet. Scientists think these interactions are similar to the processes that occurred in the early solar system when planets were first forming. Observing these processes can help astrophysicists create and refine simulations of the early solar system.
Like Jupiter, Saturn has remarkable weather and climate. A jet stream at the planet’s north pole forms a hexagon. Occasionally, enormous storms form and produce white clouds that wrap around the northern hemisphere. Scientists study such phenomena to determine their causes and effects.
A few space probes visited Saturn in the 1900’s. Pioneer 11 flew by Saturn in 1979, and the Voyager probes flew by in 1980 and 1981. But the planet was not studied in detail until 2004, when the Cassini probe entered orbit around Saturn. Cassini’s mission was a collaboration between NASA, ESA, and the Italian Space Agency (ASI).
Cassini was one of the most productive space missions ever. The mission collected detailed data on Saturn’s magnetic field, gravity, temperature, and composition. Cassini observed a change of seasons and studied one of Saturn’s huge northern storms up close. It completed numerous flybys of Saturn’s moons.
Cassini also explored Saturn’s rings in depth. It studied the shapes, textures, and elements found in each ring. Scientists learned more about the relationships between Saturn’s moons and its rings. Cassini showed that one of Saturn’s rings is mostly made of material spurting from the moon Enceladus.
The final stage of Cassini’s mission was called the Grand Finale. This dramatic series of maneuvers allowed the spacecraft to obtain close measurements of Saturn’s magnetic field, take close-up photographs, and directly sample the atmosphere. Cassini closely orbited Saturn 22 times, swooping through the unexplored area between the planet and its rings. Each orbit brought Cassini closer to Saturn. It entered the planet’s atmosphere in late 2017. Cassini continued transmitting data from within the atmosphere until it disintegrated.
Moons of Jupiter and Saturn.
Past missions to the gas giants have usually observed their moons. But the earliest flyby missions focused heavily on the planets, and only collected basic data from the moons. Nevertheless, even basic data and low-resolution images showed that the moons had intriguing, unexpected characteristics. Missions to Jupiter and Saturn began to dedicate more time and resources to studying their moons. Today, several moons are considered some of the most important destinations for space exploration.
Galileo’s observations of Europa, a moon of Jupiter, and Cassini’s observations of Enceladus, a moon of Saturn, suggested that these moons have subsurface (underground) oceans. Such oceans may provide the conditions necessary for life to develop. This discovery thrilled scientists and space agencies. Other moons interesting to scientists include Saturn’s moon Titan and Jupiter’s moons Callisto, Ganymede, and Io.
Leading planetary scientists consider Enceladus an important place to explore. Enceladus has an icy shell that is thought to conceal an ocean of water. Cracks in the ice shoot out plumes of water vapor and other material at 800 miles (1,300 kilometers) per hour. The material shoots directly into space. A probe can sample the underground ocean without needing to dig, drill, or land on the surface at all.
Voyager photographs showed that Enceladus has a smooth, shiny surface. Not much else was known about the small moon until Cassini visited it in the 2000’s. Cassini detected cracks in the moon’s ice, and the material gushing out of them. Cassini flew directly through the plumes and found that they contained a mix of water vapor, carbon dioxide, methane, and other gases. But Cassini’s instruments were not designed for this kind of sample analysis. Scientists want to send a future probe to Enceladus with more specialized instruments. No such probe has yet been developed.
Another well-known “ocean world” is Jupiter’s moon Europa. Europa was first explored in depth by the Galileo probe. Galileo detected magnetic disturbances around Europa that suggested a vast amount of salty liquid water was hidden under the icy surface. Scientists suspect plumes of water vapor escape through cracks in the ice on Europa, as they do on Enceladus.
To gather proof of Europa’s ocean, NASA is preparing to send another spacecraft to Europa. The mission, called Europa Clipper, is designed to map Europa’s surface and probe its interior. With data from Europa Clipper, scientists hope to learn more about what lies beneath Europa’s ice.
The only moon that has been visited by a lander is Saturn’s moon Titan. Early Saturn probes, such as Pioneer and Voyager spacecraft, could not peer through this moon’s thick, hazy atmosphere. The Cassini mission, and the Huygens lander it sent to Titan’s surface, gathered much more information about the moon. Huygens collected data from within the atmosphere and took photos from the surface. The mission found that Titan has weather and surface features similar to Earth’s. It found lakes of liquid methane, and dunes made of sandlike grains. Loading the player...
Volcano on Titan
Several upcoming missions target moons of the gas giants. ESA’s JUICE (Jupiter Icy Moons Explorer) is to study Jupiter, Ganymede, Europa, and Callisto. JUICE’s science team aims to create detailed maps of the moons’ surfaces and to learn more about their interiors. JUICE launched in 2023 and will reach Jupiter in 2031. Europa Clipper is expected to launch in the mid-2020’s. NASA is also planning a Titan mission, Dragonfly, expected to launch in the late 2020’s. In addition, Juno’s mission has been extended through the mid-2020’s. The spacecraft’s extended mission includes multiple flybys of Europa, Ganymede, and Io.
Exploring the ice giants
Uranus and Neptune are the least explored planets in our solar system. Only one probe, Voyager 2, has visited these mysterious blue worlds. Scientists may not fully understand how our solar system formed and evolved until these planets have been studied more closely.
Uranus and Neptune are nicknamed “ice giants.” Their thick, clouded atmospheres are full of ice crystals. Astronomers estimate hundreds of exoplanets may be ice giants. Like Mercury, Uranus and Neptune could be used as models to help scientists form hypotheses about exoplanets. But Uranus and Neptune are not yet well understood.
NASA’s Voyager 2 visited Uranus in 1986 and Neptune in 1989. The probe captured spectacular photographs of the planets and collected data on their composition and magnetic fields. It discovered 10 moons of Uranus and 5 moons of Neptune. Photographs of Neptune’s moon Triton revealed a surface dotted with geysers. Scientists think cryovolcanoes (cold volcanoes that spew slushy ice) may be common on Triton.
Ice giants differ from both terrestrial planets and gas giants in size, temperature, and composition. It is not clear what caused the planets to evolve differently. In addition, little is known about the interior structure of the ice giants.
Scientists are particularly interested in studying these planets’ atmospheres. Huge storms are known to appear and disappear on Neptune. In well-known Voyager images, Uranus’s atmosphere appears smooth and still. However, in different seasons of the Uranian year, telescopes have spotted storms like those on Neptune. It is also unclear how Uranus’s sideways rotation affects its atmosphere. Scientists hope to send an atmospheric probe to Uranus in the 2030’s.
Exploring small solar system bodies
Small bodies in our solar system include dwarf planets, asteroids, and comets. Studying these objects can help scientists learn more about the solar system’s history. They can reveal information about how the planets formed and settled in their current orbits. Loading the player...
Collecting asteroid samples
In addition, many of these objects are rich in raw materials. Some experts think that with future technology, people could mine these raw materials and use them in construction projects in space.
The smallest objects in the solar system, including asteroids and comets, are difficult for probes to land on. Without strong gravity holding them to the ground, spacecraft can bounce uncontrollably off the surface. For these reasons, most missions to asteroids, comets, and dwarf planets have collected data from orbit or during flybys. As robotic technology improves, landers may become more common.
Dwarf planets
are unusual bodies that do not fit well into any other category. From Earth, dwarf planets appear small and distant. Exploring them with probes has revealed surprising and interesting characteristics.
Probes explored dwarf planets for the first time in 2015. NASA’s Dawn probe orbited Ceres, which orbits the sun in the asteroid belt. NASA’s New Horizons probe studied Pluto and its moons. These missions found evidence that dwarf planets may have had ancient oceans. Pluto may have a subsurface ocean today.
Asteroids.
In the early 1990’s, when NASA’s Galileo probe was on its way to Jupiter, it flew by the asteroids Gaspra and Ida to take photographs and collect data. Galileo’s photographs—the first ever close-up images of asteroids—made people realize how exciting it could be to study asteroids with modern technology.
Most asteroids in our solar system orbit the sun in the asteroid belt between Mars and Jupiter. But these “main belt” asteroids are not the only asteroids that scientists study. Many institutions, such as NASA’s Center for Near Earth Object Studies, study near-Earth asteroids. These asteroids’ orbits bring them close to our planet. Researchers study near-Earth asteroids so that people can be prepared in case Earth is in danger of being hit by a large asteroid. In 2022, NASA’s DART (Double Asteroid Redirection Test) spacecraft intentionally collided with an asteroid, thus changing the asteroid’s path through space. DART’s target asteroid was not a threat to Earth. Scientists planned the mission as only a test to find out whether a spacecraft collision could redirect an asteroid. This method could be used in the future to direct threatening asteroids away from Earth.
Other asteroids, called Trojan asteroids, follow the orbital paths of larger bodies. These asteroids are some of the oldest objects in the solar system. In 2021, NASA launched the Lucy mission, which is the first probe designed to study Trojan asteroids.
The first spacecraft to collect samples from an asteroid was JAXA’s Hayabusa probe. In 2005, Hayabusa landed on the asteroid Itokawa. The probe’s sample collection mechanism did not work correctly, so only a very small amount of dust was collected and returned to Earth. Later sampling missions were more successful. Hayabusa’s successor, Hayabusa2, collected over 5 grams (about 1⁄5 ounce) of material from the asteroid Ryugu in 2019, and NASA’s OSIRIS-REx probe collected a sample from the asteroid Bennu in 2020.
Comets.
Several ambitious, technically challenging missions have studied comets. In 2004, the Stardust probe gathered samples from a comet and returned them to Earth for study. Scientists found the samples to be rich in hydrocarbons, chemicals considered to be chemical precursors of life. In 2005, the Deep Impact mission slammed an impactor into a comet while a flyby craft recorded the crash. Both Stardust and Deep Impact were NASA missions.
In 2014, ESA’s Rosetta probe became the first probe to orbit a comet. Rosetta deployed a lander called Philae. Philae was equipped with harpoons to anchor it to the comet’s surface. The harpoons did not deploy correctly, and Philae bounced off the surface before landing in a crevice. However, both Rosetta and Philae were able to send data to Earth as the comet made its closest approach to the sun.
Exploring interstellar space
Because of the great distance between Earth and the edge of the solar system, few spacecraft have reached interstellar space. The two Voyager spacecraft entered interstellar space in the 2010’s. Their instruments have enough power to collect limited data from interstellar space, such as measurements of magnetic fields and gases. The Pioneer and New Horizons spacecraft are on trajectories that also will take them outside the solar system.
History of space exploration
In the 1600’s, the German astronomer and mathematician Johannes Kepler became the first scientist to describe travel to other worlds. He also developed the laws of planetary motion that describe how planets move in their orbits. The English scientist Sir Isaac Newton described the laws of gravity. These scholars’ contributions enabled scientists to design flight paths for spacecraft to reach orbit and travel to other worlds.
During the 1700’s, scientists realized that air got thinner at higher altitudes. They concluded that there was no air between Earth and other worlds. Without air, wings would be useless. Writers imagined fanciful techniques for travel to these worlds, such as bizarre vehicles that resembled trains or hot air balloons.
The dawn of the space age
In the early 1900’s, professional and amateur scientists analyzed the practical aspects of space travel. In 1903, the Russian high-school teacher Konstantin Tsiolkovsky wrote the first scientific paper on the use of rockets for space travel. Other Russian (and later, Soviet) thinkers, such as Yuriy Kondratyuk and Fridrikh A. Tsander, calculated efficient spaceflight trajectories and proposed future missions. Kondratyuk’s plan for a moon landing, with one vehicle in orbit and another landing on the lunar surface, was almost the same as the mission design used by NASA to land on the moon many years later. By the 1920’s, spaceflight was a common topic of discussion among Soviet scientists. Amateur rocketry groups met to discuss Tsiolkovsky’s ideas. Such groups were soon found across Europe.
Robert H. Goddard of the United States and Hermann Oberth of Germany also awakened wider scientific interest in space travel. Goddard published explanations of how rockets could be used to explore Earth’s atmosphere and the moon. Oberth created technical rocket designs and calculated the physics of multistage rockets. Goddard, Oberth, and Tsiolkovsky worked independently, but made many of the same discoveries. They are sometimes called the “fathers of spaceflight.”
The first space rockets.
In the 1920’s and 1930’s, engineers began to build the first modern rockets. Goddard’s team created the first liquid-propellant rocket in 1926. In 1933, with financial help from the Soviet government, a group of rocket designers built and launched the first liquid-propellant rocket in the Soviet Union. The group was led by an engineer named Sergei P. Korolev. After these successes, governments in both the United States and the Soviet Union began to invest more heavily in rocket development. But in the late 1930’s, Soviet rocket development was interrupted by the Great Purge, an organized effort by the Soviet dictator Joseph Stalin to remove certain people from positions of power. During this time, key scientists and engineers such as Korolev were imprisoned and sent to labor camps.
The most powerful rocket technology of the time was developed in Germany. During World War II (1939-1945), German rocket experts led by Wernher von Braun developed the V-2 guided missile (also known as the A-4). Thousands of V-2’s were fired against European cities, especially London, causing widespread destruction and casualties. The war made it clear that German rocket technology was the most advanced in the world. If the United States or the Soviet Union wanted to get ahead in rocket technology, they would need German scientists and engineers.
When World War II ended in 1945, the leading German rocket engineers went to work for the U.S. government to help develop military missiles. In exchange, they were not prosecuted for their role in the war.
The first artificial satellites.
In 1955, both the United States and the Soviet Union announced plans to launch satellites with scientific instruments on board. The satellites were to be sent into orbit as part of the International Geophysical Year, a period of international cooperation in scientific research beginning in July 1957. At the time, the Soviet rocket program was a secret. Many people in other countries doubted the Soviet Union would really be able to launch a satellite.
On Oct. 4, 1957, the Soviet Union launched the first artificial satellite. The satellite, named Sputnik, was about the size of a beach ball. Radio listeners around the world picked up Sputnik’s “beep-beep” signal.
The space race begins.
The world reacted to the launch of Sputnik with surprise, fear, and respect. Soviet Premier Nikita Khrushchev ordered massive follow-up projects that would continue to amaze the world. In the United States, leaders vowed to do whatever was needed to catch up to Soviet accomplishments. Thus the “space race” began.
More Soviet successes followed. A month after Sputnik, another satellite, Sputnik 2, carried a dog named Laika into space. The flight proved that animals could survive the unknown effects of weightlessness for at least a few hours. In 1959, several Soviet probes reached the moon.
The first U.S. satellite was Explorer 1, launched in 1958. United States satellites were smaller and lighter than their Soviet counterparts because American launch rockets were less powerful than those used by the Soviet Union. The Soviet Union’s rockets gave it an early lead in the space race. Because bigger rockets would be needed for crewed lunar flight, both the United States and the Soviet Union began major programs of rocket design, construction, and testing.
In 1958, the U.S. government established a civilian space agency called the National Aeronautics and Space Administration (NASA). NASA absorbed various existing civilian and military laboratories. The formation of NASA helped forge agreement among competing interests, including military branches, universities, the aerospace industry, and politicians. This centralized planning was key to the success of U.S. space exploration.
Soviet space activities, on the other hand, were coordinated by special executive commissions. These commissions tried to tie together military and industrial space centers, as well as competing experts and scientists. But the commissions did not always coordinate missions effectively enough to meet the complex challenges of the space race.
The first astronauts enter space
In 1958, scientists in the United States and the Soviet Union began designing spacecraft that could carry human beings. The possibility of sending humans into space was exciting, but no one knew how space travel would affect astronauts. Tests with animals showed that humans would most likely survive space travel. But some experts feared the psychological stress of launch, flight, and landing might drive an astronaut to terror or even unconsciousness.
The race to the moon dominated the space race of the 1960’s. In a 1961 speech, President John F. Kennedy called for the United States to commit itself to “landing a man on the moon and returning him safely to the earth” before the 1960’s ended. This goal was intended to show the superiority of U.S. science, engineering, and political leadership.
Vostok and Mercury.
The Soviet Union’s Vostok program and NASA’s Mercury program were the first efforts to send human beings into space. The Vostok capsule consisted of a spherical pilot’s cabin and a cylindrical service module, the section containing the propulsion system. The capsule was designed with an ejection seat for emergency escapes, and a life-support system with a mixture of oxygen and nitrogen.
The cone-shaped Mercury capsule would use parachutes to land in the ocean, where the water would provide extra cushioning. Mercury’s life-support system used pure oxygen at low pressure. If a booster malfunctioned during launch, the capsule and pilot would be pulled free of the booster by a rocket attached to the nose of the capsule.
While U.S. plans proceeded in the glare of publicity, Soviet developments took place in great secrecy. In 1960 and 1961, both nations conducted tests of their vehicles with no humans aboard. Many launches failed. Some test flights carried animals. In 1961, a cosmonaut trainee named Valentin Bondarenko burned to death in a pressure-chamber fire. Bondarenko’s death was the first fatal accident in a space program. Soviet officials kept the accident secret.
On April 12, 1961, a Soviet pilot named Yuri A. Gagarin became the first human being to travel to space. Gagarin orbited Earth aboard a Vostok spacecraft and returned safely. An automatic flight control system managed the spacecraft’s operations during the entire flight. The Mercury program made its first human spaceflight on May 5, 1961, when astronaut Alan B. Shepard, Jr., flew a -15-minute suborbital mission.
During another suborbital Mercury mission in July, astronaut Virgil I. (Gus) Grissom had to be rescued when his capsule flooded after splashdown in the Atlantic Ocean. On Feb. 20, 1962, John H. Glenn, Jr., became the first American to orbit Earth. He completed three orbits in less than five hours.
The first woman in space.
Many people believed women would eventually travel in space. Some people thought that women should train to become astronauts because they were smaller and lighter than men and would therefore require fewer resources. Others thought that in the future, traveling and even living in space would be commonplace. These people imagined women would be needed in space to perform jobs that were considered “women’s work,” such as caring for children.
Many Americans wanted NASA to send the first woman to space before the Soviet Union could launch a woman cosmonaut. Experienced women pilots from all parts of the United States volunteered to serve as astronauts. In 1962, Congress debated whether NASA should consider training women. At the time, NASA wanted astronauts to have experience as military test pilots, but this career was only available to men. Congress decided that because no women were expected to meet this requirement, NASA should not use its resources to train women as astronauts.
In 1963, Valentina Tereshkova of the Soviet Union became the first woman to travel in space. Tereshkova was one of a group of women selected by the government to train as cosmonauts. Most of these women, including Tereshkova, were accomplished skydivers.
The Soviet government had wanted to send the first woman to space to achieve a “first” in the space race. They wanted such a mission to show that in the Soviet Union, women were equal to men. Yet only one other woman, Svetlana Savitskaya, reached space as a Soviet cosmonaut.
Developing multiperson spaceflight.
On the first multiperson space missions, astronauts and flight controllers gained vital experience in enduring longer missions and handling emergencies. These missions were used to prepare for the tremendous challenges of sending human beings to the moon.
In 1961, the United States announced the Gemini program, which would send two astronauts into space in an enlarged version of the Mercury capsule. This announcement spurred Soviet planners to modify their Vostok capsule to carry up to three cosmonauts. The political pressure to upstage U.S. efforts was so intense that Soviet engineers sacrificed safety features, such as ejection seats, to enlarge the capsule.
Voskhod 1, the first spacecraft to carry multiple crew members, was launched into orbit in 1964. In 1965, during the Voskhod 2 mission, the Soviet cosmonaut Alexei Leonov became the first person to walk in space. After this success, however, the Voskhod 2 crew missed their target landing zone and came down in an isolated forest. The cosmonauts had to fend off wolves until they were rescued the next day.
Crewed Gemini missions began in 1965. In 1966, Gemini 8 linked up with a rocket in space. It was the world’s first docking of two space vehicles. The Gemini 8 spacecraft then went into a violent tumble, and the crew had to make an emergency splashdown in the western Pacific Ocean. Later Gemini crews conducted additional tests of docking and extravehicular activity.
Mission to the moon
The Gemini program helped NASA develop and test technology for sending crews to the moon. Throughout the 1960’s, robotic probes sent to the moon surveyed several possible landing sites.
The Apollo program.
In late 1968, NASA’s work on a lunar landing module was behind schedule. To reach the moon before the Soviet Union, NASA simplified the plan for its first lunar mission. It was decided that Apollo 8 would orbit the moon without landing on its surface. The orbital mission would test navigation and communication around the moon, and the astronauts would be able to scout out landing sites for a landing mission.
Some leaders were hesitant to approve the Apollo 8 mission. The change in plans would give the crew very limited time to prepare for the trip. The mission would need to use the powerful Saturn V rocket, which had not always worked perfectly in the past. Three astronauts had recently died in an accident and NASA was worried about safety.
Despite these concerns, Apollo 8 launched successfully in December 1968, carrying astronauts Frank Borman, James Lovell, and William Anders. Hundreds of thousands of people crowded nearby beaches to watch the launch. The crew traveled for three days to the moon and successfully entered lunar orbit. They carried a television camera to broadcast updates to the public during their journey. The success of the mission generated optimism and excitement for the Apollo program.
Apollo 11 was the first crewed moon landing. The first human beings stepped onto the lunar surface on July 20, 1969. Astronauts Neil Armstrong, Buzz Aldrin, and Michael Collins made up the Apollo 11 crew. They immediately became international heroes. After their return to Earth, they toured the world to meet with leaders and make public appearances.
Apollo 11 was followed by another successful moon landing, Apollo 12. But the following lunar mission, Apollo 13, almost ended in disaster when an oxygen tank ruptured. The rupture caused a series of life-threatening problems. Mission control helped the astronauts execute creative solutions that could bring them back to Earth alive. Apollo 13 has been called a “successful failure” because the crew survived despite the serious malfunction.
When the Apollo program ended, it was considered a momentous success. Over the course of the program, hundreds of research teams developed innovative technologies that were later used to improve the quality of life on Earth. For example, advances in microelectronics and new medical monitoring equipment were developed as a result of the Apollo program.
Soviet attempts to reach the moon.
The Soviet Union had a secret program to send humans to the moon, but it was hindered by management problems and technical issues. Soviet lunar missions were not planned by a central authority. Rivalry among different spacecraft design teams and other space organizations prevented cooperation. Vehicles exploded during test flights. The program included the development of the N-1 rocket, comparable to the American Saturn V rocket. After four launch failures in the early 1970’s, the program ended. Officials in the Soviet Union denied there was ever a Soviet equivalent to the Apollo program. In the late 1980’s, the Soviet Union finally acknowledged that the program had existed and failed.
The space shuttle era
After the Apollo program ended, the typical space mission changed. The space shuttle and the first space stations were developed. Space missions could last longer. New vehicles could accommodate larger crews. These larger crews included more scientists with different areas of expertise. They also included astronauts from countries other than the Soviet Union or the United States. International collaboration in space became frequent.
The Apollo-Soyuz Test Project.
In 1975, the United States and the Soviet Union held the first international piloted space mission. During the Apollo-Soyuz Test Project, a Soviet Soyuz capsule and a U.S. Apollo capsule completed a rendezvous in space. This mission demonstrated trust between the two countries. But it was also a technological success. Combining the independently developed technologies would be the first step in creating important multinational projects such as the International Space Station.
Space probes of the shuttle era.
After the Apollo program, NASA received a smaller government budget. The agency could launch fewer new space probes than in the past. Many exciting missions were proposed, but it was not possible to fund them all. NASA began to select missions based on a coordinated set of prioritized research topics recommended by planetary science experts. NASA and its advisers hoped that by coordinating scientific objectives across different missions, new missions would be sure to build on the knowledge gained by previous missions. This strategy for selecting missions continues today.
Meanwhile, in areas such as Asia and western Europe, more countries began to launch satellites and small probes. Smaller countries could mount such missions because the missions required fewer resources than human spaceflight programs did. France and Japan became the third and fourth nations in space respectively. Shortly afterward, China and the United Kingdom launched their own satellites. In 1975, the European Space Agency (ESA) was organized. Its member nations combine their resources to develop spacecraft and conduct scientific experiments. In the 1980’s, India and Canada founded national space agencies.
During this period, important missions sometimes included two nearly identical probes with similar trajectories. If one probe failed, the expensive mission would not be lost. A lost mission was not likely to be attempted again soon. In some cases, such as Voyager, repeating the mission would have been impossible. In the late 1970’s, NASA’s two Voyager probes launched on a trajectory to Jupiter, Saturn, Uranus, and Neptune while these planets were aligned on the same side of the sun. These planets would not align in this way again for another 176 years.
Early space stations.
To allow astronauts to stay in space longer and to conduct experiments that were more advanced, space agencies needed to build space stations. The first space stations were cylindrical, with a docking port at one end and solar panels sticking out from the sides. The stations were designed to hold enough air, food, and water to last for several months.
In 1971, the Soviet Union launched the first space station into orbit. Salyut 1 consisted of a single module with one docking port. Its crew—Georgi Dobrovolsky, Victor Patsayev, and Vladislav Volkov—spent 23 days aboard the station, making medical observations and performing experiments. On their return journey, the air leaked out of their Soyuz spacecraft. All three cosmonauts died. On future missions, Soyuz crews wore pressure suits for protection in case a similar accident occurred. The Soyuz underwent a major redesign. The redesigned Soyuz has been upgraded over time and remains a successful operational vehicle today. Additional Salyut stations were launched from 1973 through 1976.
In 1973, NASA launched the first U.S. space station, Skylab. Skylab was built from the empty third stage of a Saturn V rocket, with an attached air lock module, docking port, and solar telescope. The station suffered damage during launch, but its first crew successfully fixed the damage. Two more crews carried out Skylab missions. These astronauts continued to operate the station while conducting medical experiments, photographing Earth, and observing the sun.
In 1977, the Soviet Union launched Salyut 6 into orbit. Salyut 6 had two docking ports, one at either end of the main module. The additional port enabled a space station crew to receive a visit from a second crew or a resupply vehicle. Salyut 6 became the first space station to be resupplied and refueled. These capabilities extended the life of space stations and enabled crews to repair and upgrade them. Spare parts and new instruments could be sent to the stations as needed. Salyut 6 operated for almost five years. Individual missions lasted as long as six months. The station’s successor, Salyut 7, housed expeditions lasting up to eight months.
The space shuttle.
The U.S. space shuttle was the first reusable launch system. The shuttles launched like a rocket and landed like an airplane. They were designed to last for over 100 missions.
Advocates of winged spaceplanes pointed out that such vehicles could land on normal runways. Adding wings to a spacecraft increases the vehicle’s weight, but landing a winged spaceplane is easier and cheaper than splashdowns at sea. Ocean landings require many ships and aircraft, and salt water often damages the spacecraft.
The designers of the space shuttle faced major technological challenges. The shuttle’s main engines had to be reusable for many missions. The shuttle needed a flexible but reliable system of computer control. It would need to survive many reentries into Earth’s atmosphere, so a new type of heat shield was needed.
The shuttle’s first orbital mission was in 1981. The first four shuttle flights each carried only two pilots. The crew size was soon expanded to four, and later to seven or eight members. Besides the two pilots, shuttle crews included mission specialists (experts in the operation of the shuttle) and payload specialists (experts in the scientific research to be performed).
Space shuttles were used for many distinct types of missions. They carried artificial satellites, space probes, and other heavy loads into orbit around Earth. Probes bound for deep space, such as Magellan and Galileo, were released from the shuttle with a rocket attached. The rocket would then fire to send the probe on its interplanetary trajectory. In addition to launch operations, the shuttles could retrieve artificial satellites that needed servicing. Astronauts aboard the shuttle repaired the satellites and then returned them to orbit. Shuttle crews also conducted many kinds of scientific experiments and observations.
The space shuttle could carry many more passengers than previous launch vehicles. More scientists could go to space, and scientific research in space expanded. The shuttle also carried passengers who were not NASA astronauts or scientists. Citizens who participated in shuttle missions included representatives of the companies launching payloads, and two U.S. congressmen.
In 1986, the space shuttle Challenger disintegrated into a ball of fire 73 seconds after it launched. All seven crew members were killed. Shuttle missions were halted while officials researched the cause of the accident. NASA was heavily criticized for failing to prevent the accident. Investigators found that engineers had expressed concerns about the safety of the shuttle, but managers did not address these concerns. If NASA’s safety protocols had been more thorough, officials would have called off the launch.
Following the accident, shuttle designers worked to improve the vehicle’s safety. They made technical modifications and added a crew bail-out system. Officials mandated stricter safety reviews and more restrictive launch conditions.
The Soviet Union conducted a secret shuttle program, Buran, during the 1980’s. Buran resembled NASA’s shuttle, but Soviet engineers made many modifications. For example, Buran had no main engines on board. Instead, an expendable booster provided all its launching power. Buran completed uncrewed tests, but the program did not have enough funding to proceed. In 1993, work on the program ended.
Mir.
In 1986, the Soviet space station Mir was launched into orbit. Mir had two docking ports—one at each end—and four other hatches where laboratory modules were added later. Mir hosted many of the longest space missions. Cosmonauts lived on the station for months at a time.
After the Soviet Union broke apart in 1991, the new Russian space agency, Roscosmos, took over the operation of Mir. In 1995, NASA’s space shuttles began to dock with Mir. The station operated until 2001.
The International Space Station.
In 1984, U.S. President Ronald Reagan instructed NASA to build a large, permanent space station. This planned station would become the International Space Station (ISS). NASA, Roscosmos, CSA, JAXA, and ESA all contributed to developing and maintaining the ISS. The engineers who designed the ISS had to integrate module designs, computer systems, and other technologies from several different countries. The proposed design of the ISS changed frequently throughout the 1980’s and 1990’s.
The first ISS module, called Zarya, was launched into orbit in 1998. The station’s first mission, Expedition 1, began in 2000. Bill Shepherd of the United States and Yuri Gidzenko and Sergei Krikalev of Russia made up the Expedition 1 crew. Since 2000, there has been a crew living on the ISS at all times. Before each crew returns to Earth, they officially transfer command of the station to a new crew.
The Shenzhou spacecraft.
In the 1990’s, China began developing the Shenzhou, a spacecraft designed to carry astronauts. The Shenzhou resembles Russia’s Soyuz capsule. It is slightly larger than the Soyuz.
In 2003, China became the third nation to launch a person into space. The Chinese astronaut Yang Liwei orbited Earth aboard a Shenzhou craft for 21 hours. During a Shenzhou mission in 2008, two astronauts performed the country’s first spacewalk. Many missions in the Shenzhou program have worked toward evaluating the technology and procedures needed to operate a permanent space station.
Ending the space shuttle program.
In the early 2000’s, NASA planned to continue using the space shuttle for transportation until at least 2020. Then, in 2003, the space shuttle Columbia broke apart during reentry. The accident killed the seven-person crew. Once more, NASA’s management was heavily criticized. Investigators uncovered significant communication problems and organizational flaws within NASA. The shuttle program had been under intense pressure to meet deadlines and stay within budgets. The investigators concluded that this pressure had compromised the safety of the space shuttle.
In 2004, U.S. President George W. Bush announced that after construction finished on the ISS, the space shuttles would be retired. The final space shuttle mission took place in 2011. After this mission, NASA purchased seats on Soyuz spacecraft from Roscosmos to deliver astronauts to the ISS.
The commercial space era
As the space shuttle program ended, private space launch companies became more prominent in the United States. They worked to develop new vehicles and commercial launch services.
Developing new reusable spacecraft.
In the early 2000’s, space agencies and private companies worked to develop new reusable launch vehicles (RLV’s). NASA officials decided that it would be unrealistic to develop a new RLV quickly enough to be ready when the shuttle program ended. However, without the space shuttle, NASA had no way to carry astronauts to and from the ISS. The agency would have to pay for astronauts to travel on Soyuz vehicles. In 2008, NASA hired the American companies Orbital ATK and SpaceX to develop spacecraft that could reach Earth orbit.
In the meantime, NASA concentrated on developing the Crew Exploration Vehicle, also known as Orion. Orion is a capsule-shaped spacecraft for transporting crew and cargo. It could eventually carry astronauts to the moon or Mars. In 2011, NASA announced plans for the Space Launch System (SLS), a rocket designed to carry Orion into space.
Private companies developed diverse designs for reusable spacecraft. The American company Scaled Composites built a rocket-powered plane called SpaceShipOne. SpaceShipOne launched in the air from a carrier plane and landed on a runway. Boeing’s Starliner capsule and SpaceX’s Dragon are reusable capsules launched by rockets. After reentry, the Starliner capsule lands on the ground. Dragon capsules land in the water.
Some companies have also designed reusable boosters. SpaceX’s Falcon rockets have a fully reusable first stage. When the rocket’s stages separate, the used first stage lands upright on four legs. The company Rocket Lab has tested a different method of recovering boosters. As the used booster falls toward the ocean, a helicopter equipped with a grappling hook catches the booster in midair.
Commercial spacecraft take flight.
In the 2010’s, private companies contracted by NASA began carrying supplies and crew to the ISS. Dragon and Cygnus spacecraft deliver supplies to the ISS. The Cygnus expendable spacecraft was designed by Orbital ATK and is now built by the American company Northrup Grumman.
The development of commercial spacecraft enabled the era of space tourism to begin. Although Soyuz missions to the ISS occasionally included space tourists, these opportunities ceased in 2011. In that year, the space shuttle program ended, and extra Soyuz seats were filled by NASA astronauts. In 2021, several companies began launching short suborbital spaceflights for the purposes of space tourism.
Today, NASA’s Commercial Crew program contracts with private companies to take astronauts to the ISS. In 2020, the SpaceX Crew Dragon capsule became the first private spacecraft to take astronauts into orbit. The mission was the first crewed launch from the United States since the last shuttle mission.
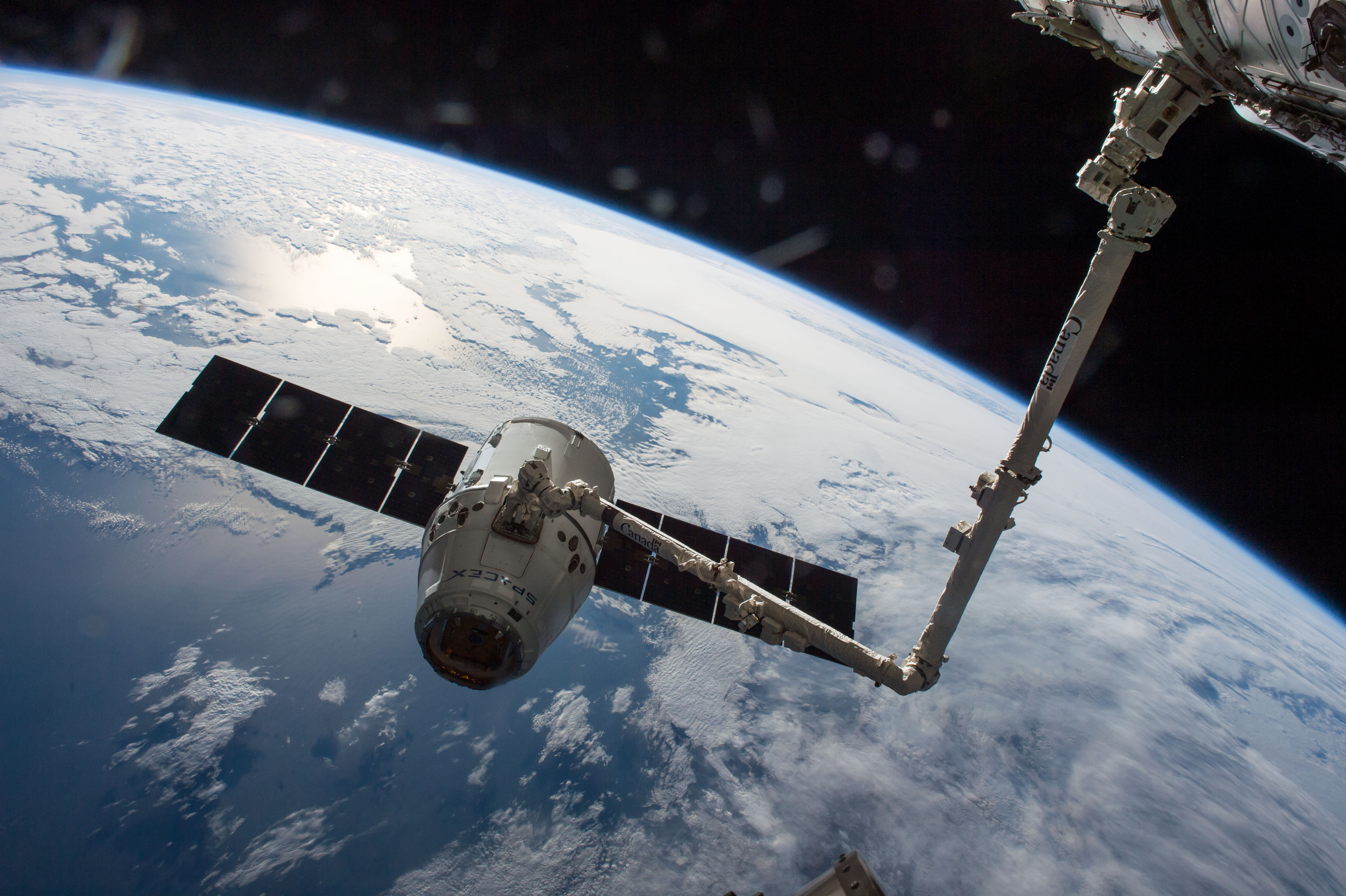
Space stations today.
In the 2010’s, the Chinese space program worked to develop the capabilities needed to support a permanent space station. Temporary space stations were launched into orbit and inhabited by astronauts. In 2022, construction was completed on China’s first permanent space station, Tiangong. This station consists of a core module, where astronauts live, and two science modules, where they conduct experiments. Tiangong can accommodate three crew members.
In 2014, the United States’ relationship with Russia became strained when Russia seized control of Crimea. The two countries’ relationship was further strained in 2022, when Russia invaded Ukraine. The ISS is expected to continue operating until 2030. NASA plans to collaborate with private corporations to maintain the station until it is de-orbited.
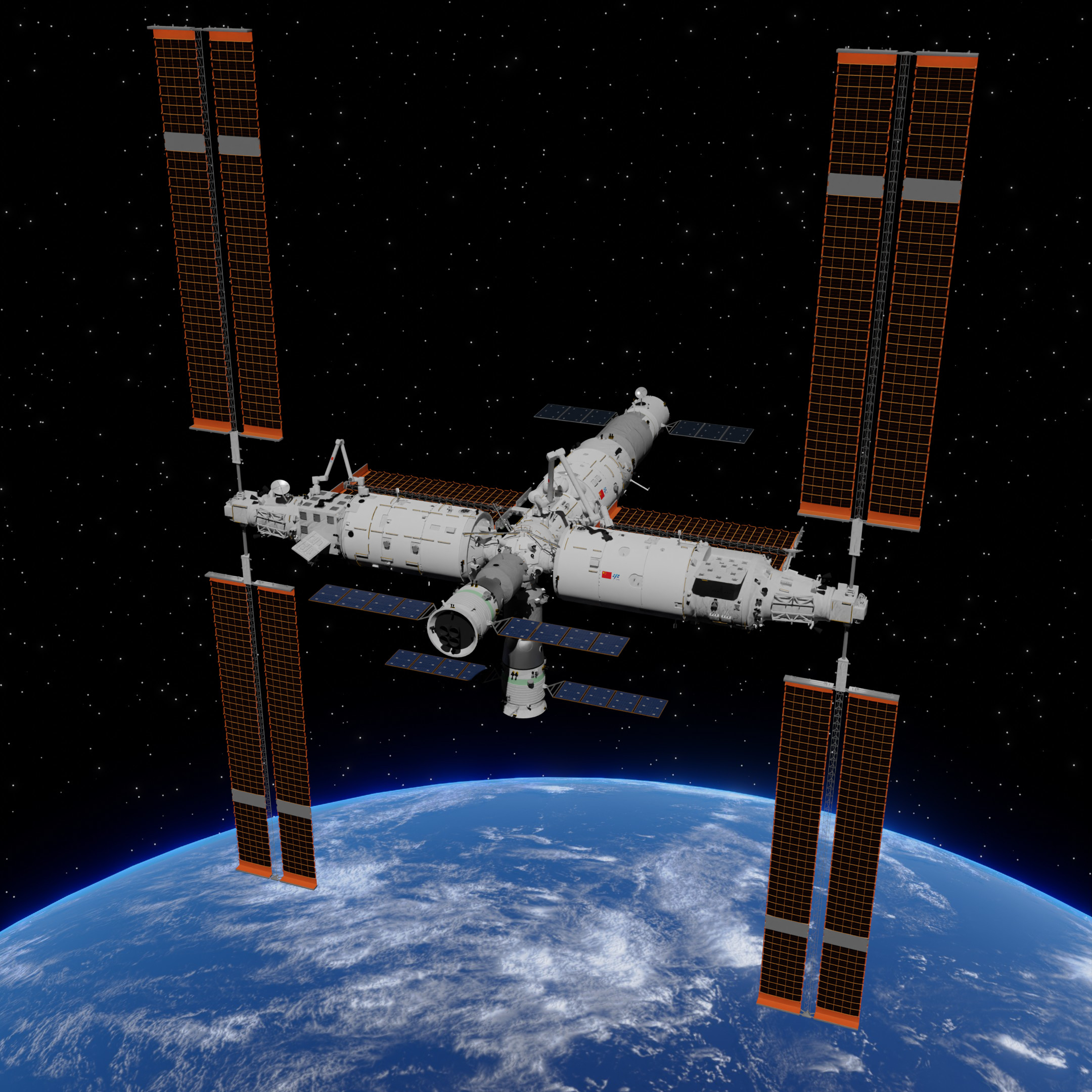
Careers in space exploration
Astronauts have the most well-known career in space exploration. But many more people work on Earth to make space missions possible.
Astronauts
work hard under dangerous, stressful conditions. Each space agency has its own required qualifications to become an astronaut. In general, astronauts must be highly educated in science or engineering. They must also be physically fit. Astronauts assigned to the ISS may need to learn a new language to communicate with their international crewmates.
Engineers.
Not every space mission involves an astronaut, but all space missions need engineers. Engineers design spacecraft and robotic equipment. They select construction materials to protect spacecraft from the hazards of space. Software engineers create computer programs to communicate with and operate spacecraft. Safety engineers equip spacecraft for emergencies.
Scientists
interpret images, measurements, and other mission data to discover new knowledge about space. Scientists also help decide the goals of a space mission. They design missions to collect data that will help resolve current scientific questions. The scientists working on a single space mission may have a wide variety of expertise. Geologists, chemists, and astrophysicists frequently work on space missions.
Other careers.
Space missions are complex undertakings. Successful missions require people with a wide range of specialties to work together. Doctors and nutritionists keep astronauts healthy in space. Technicians build spacecraft and remotely operate rovers. Project managers make executive decisions and coordinate the work of many teams of people to keep missions on schedule.
Plans for the future
Searching for life in our solar system.
Many people once thought that Earth was the only place whose current planetary conditions could support life. It was widely believed that if extraterrestrial life existed, it would be found in another star system on a planet that closely resembled Earth.
Then, scientists found evidence of oceans on worlds entirely different from Earth, such as Jupiter’s moon Europa. This discovery transformed the search for extraterrestrial life. If such “ocean worlds” are common in our solar system, they are also likely common in other star systems. If moons within the solar system are found to support life, millions of other star systems could also support life.
Recently, this exciting idea has influenced the planning of space missions. Several probes are preparing to explore moons in our solar system. The results of these investigations will likely determine the future of the search for extraterrestrial life.
New moon missions.
NASA’s Artemis program is a plan for future crewed moon missions. The stated goals of the program are to send astronauts to the surface of the moon and to gain experience for future missions to Mars. NASA has contracted several private companies, such as SpaceX, to develop technology for the Artemis program. The first Artemis mission, an uncrewed test flight, launched in 2022.
The China National Space Administration has also announced plans to develop a crewed moon exploration program. Future Chang’e missions have been planned for the 2020’s and the early 2030’s. These uncrewed missions would assess technology for a crewed lunar outpost.
NASA, ESA, JAXA, and CSA plan to develop a permanent space station in orbit around the moon. The station would provide a place for astronauts to prepare for lunar landing missions. Crewed missions on their way to Mars could stop at the station to fuel a spacecraft or pick up supplies.
Traveling to Mars.
NASA aims to send astronauts to Mars in the 2030’s or 2040’s. Many technical and logistical challenges will need to be overcome before humans can safely reach the planet.
Exploring other star systems.
Over the past few decades, astronomers have discovered thousands of exoplanets orbiting other stars. Some of these planets resemble familiar worlds like Jupiter or even Earth. Others are unlike any of the planets in our solar system. Scientists can study these planets using telescopes. But because these planets are many light-years away, it is difficult to obtain precise information about them. A probe to another star system could study exoplanets close up. Space agencies and nonprofit organizations have begun to create early designs for such probes. But great developments in technology are needed to enable travel to other star systems. It is likely that the first of these probes will not be launched for several decades or more.