Time is both a useful and familiar concept and an enduring mystery. Keeping track of time helps us order our activities and coordinate them with other people. People use watches and clocks to determine when to attend school and work, to arrange meetings and other events, and to keep airlines, buses, and train systems running smoothly. Telling time ranks among the most important skills that children learn at an early age.
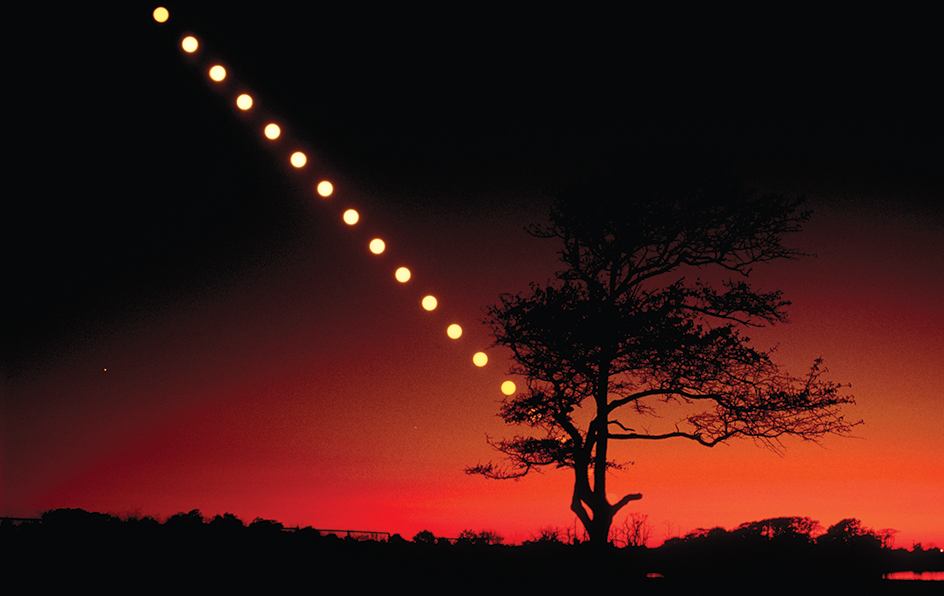
We can measure time more accurately than any other quantity. In fact, much of modern technology could not function without precise timekeeping and synchronization (coordination in time). The worldwide navigation network called the Global Positioning System (GPS), for example, uses time signals broadcast by satellites to precisely determine a unit’s location. For GPS units to function reliably, clocks on the satellites must vary from one another by one billionth of a second or less. Other devices that require precision synchronization include cellular telephone and computer networks, radio and television systems, radar equipment, electric power grids, and many scientific instruments.
The nature of time, on the other hand, remains among the deepest mysteries. Scholars have described time as something that flows or as a way of ordering events in sequence. Both of these ideas, however, only describe our experience of time. They do not explain what time really is. Throughout history, scientists and philosophers have struggled to better understand and define time.
The first two sections of this article, Measuring time and Time in today’s world, describe historical and modern methods for keeping track of time. The last section, Our understanding of time, discusses ideas from science and philosophy about the nature of time.
Measuring time
Nearly all methods of measuring time involve observing periodic (regularly repeating) events. Such events include cycles of motion in the heavens, actions performed by mechanical clocks, and changes in nature.
Astronomical cycles.
Even before people developed writing, they probably kept time by observing motions in the heavens. The rising or setting of the sun can serve to mark the passage of a day—that is, one rotation of Earth. The phase (apparent shape) of the moon varies in a cycle that lasts about 291/2 days, the basis of a month. Our view of the stars changes slightly each night, with different constellations becoming visible each season. A full cycle of these constellations marks a single year. Studying heavenly motions enabled early civilizations to properly time agricultural activities and religious rituals.
The use of the day as a basic unit of time gave rise to two slightly different systems of timekeeping. These systems are: (1) solar time and (2) sidereal time.
Solar time
measures the apparent motion of the sun. In most places, the sun appears to reach its highest point in the sky once each day, an event called local noon. One solar day equals the time between two consecutive local noons.
Some of the earliest timekeeping devices, called sun clocks or shadow clocks, used the movement of shadows to measure solar time. More than 5,000 years ago, the ancient Egyptians erected pillarlike monuments called obelisks. An obelisk’s shadow changed position and length as the sun moved across the sky, enabling people to track the time of day. More advanced sun clocks called sundials included markings that revealed the hour of day based on the position of an object’s shadow.
Sidereal time
measures the apparent motion of the stars. One sidereal day is the time it takes a certain star to reach its highest point in the sky two times in a row.
A sidereal day basically measures one complete rotation of Earth. A solar day lasts slightly longer because, in addition to rotating, Earth moves around the sun in its orbit. This motion requires Earth to complete slightly more than a full rotation before the sun appears in the same position in the sky. For this reason, a sidereal day measures about 4 minutes longer than does a solar day.
Artificial clocks.
Timekeeping methods based on the sun and stars have several limitations. Sun clocks work only when the sun is shining, and many kinds require regular adjustment for changes in the sun’s apparent motion. The sun and stars also move too slowly to be of much use in measuring intervals shorter than an hour. As civilizations began to require more precise and convenient timekeeping, people developed artificial clocks.
Clocks that measure change.
The first artificial clocks measured some change that proceeded at a fairly constant rate. The water clock, used as early as 3,500 years ago, consisted of a container that water flowed into or out of at a steady rate. People measured time by comparing the changing water level to marks on the container. Simpler clocks for everyday use included candles marked at regular lengths and oil lamps with marked reservoirs. As the candle burned down or the oil burned away, the marks indicated how much time had passed.
Most of these clocks probably remained accurate only to about half an hour per day at best. Some complex water clocks, however, kept more accurate time by using special devices to regulate the flow of water.
Clocks that count repeated actions.
The first fully mechanical clocks, probably built in the late 1200’s, relied on the periodic motion of weight-driven gears. Because these clocks were complex and costly, most towns had only one. These clocks remained accurate to about fifteen minutes per day and were often reset daily to solar noon.
Accuracy increased greatly with the development of pendulum clocks in the late 1600’s. These clocks used the swinging of a pendulum, which the Italian scientist Galileo had earlier discovered to be surprisingly regular. Early pendulum clocks remained accurate to about ten seconds per day, while later, more complex models kept even better time.
Clockmakers continued to design and improve clocks and watches. By the 1800’s, most families could afford at least one timepiece, and fairly accurate timekeeping became widespread. In the late 1920’s, clockmakers began using natural vibrations of the mineral quartz as a basis for simpler, more accurate timepieces. Still common today, quartz watches and clocks can remain accurate to better than one second per day.
In the late 1940’s and 1950’s, scientists developed atomic clocks that count fundamental vibrations of atoms. The best atomic clocks remain accurate to trillionths of a second per day, not gaining or losing a second in tens of millions of years. See Atomic clock.
Natural processes.
Some natural processes preserve a record of periodic events. These “natural clocks” enable us to measure the timing of events in the past.
Radioactive decay.
Atoms of a radioactive element decay (break down) into atoms of another element. Because this decay occurs at a known rate, scientists can sometimes determine how old a sample is by measuring the amount of radioactive material it contains.
For example, some of the carbon in living things is a radioactive isotope (form) called carbon-14. Carbon-14 decays into nitrogen at a known rate, but living things constantly replenish the carbon 14 in their bodies. When an organism dies, it stops taking in new carbon-14. Thus, by analyzing the amount of carbon-14 left in a sample, scientists can often estimate how long ago an organism died.
The formation of rock.
Over billions of years, natural processes, such as the flow of lava and the deposit of sediment (tiny bits of solid material), lay down new layers of rock. These layers preserve a record of time in Earth’s past often referred to as the geological record.
Geologists use various methods to estimate the age of rock layers. By observing lava flows and sediment deposits, they can estimate how long it would take for a certain thickness of rock to develop. They can determine the ages of certain rocks using some kinds of radioactive decay.
Changes in living things.
Living things can also preserve a record of time. Many organisms grow in cycles that last a year or even a day. In colder climates, for example, trees often feature prominent growth rings produced by rapid growth during the warm months and slow growth or no growth during winter.
Evolution (the gradual development of living things) also serves as a natural clock. An organism’s DNA (deoxyribonucleic acid) includes genetic information encoded in a sequence of chemical units called base pairs. Over time, evolutionary processes cause changes in the sequence of base pairs that occur at a predictable rate. By comparing DNA from two related organisms, therefore, biologists can determine how long ago the organisms diverged (began to evolve separately) from a common ancestor.
Time in today’s world
Advances in communication and transportation have created an increasingly global society. Keeping this society running smoothly requires a standard system of timekeeping that people anywhere can use easily.
Units of time.
People once used the day as the fundamental unit of time. They measured this time by Earth’s rotation and divided it into hours, minutes, and seconds. Standards changed when atomic clocks provided a more accurate and stable means of keeping time.
In modern society, the second serves as the fundamental unit of time. By international agreement, one second equals exactly 9,192,631,770 cycles of a particular vibration of an atom of cesium-133, an isotope of the element cesium. Atomic clocks count these vibrations to keep the time of day. The second forms the basis for other familiar units of time. Sixty seconds make up a minute. An hour consists of exactly 60 minutes, or 3,600 seconds. Twenty-four hours, or 86,400 seconds, make up one day.
Most nations employ a 24-hour clock, with noon marked as 1200 and midnight as 2400 or 0000. In the United States, the military, the police, airlines, and many other groups use this system. However, most people in the United States favor a 12-hour scale, with the first hour of the day ending at 1:00 a.m. and 11:00 p.m. beginning the last hour of the day. To avoid confusion over whether to use a.m. or p.m., people often refer to noon as 12:00 noon and midnight as 12:00 midnight.
Local and standard time.
Traditionally, people determined time locally, using the sun. Each town set a main clock to noon when the sun reached its highest point overhead. Because of the sun’s apparent east-to-west motion, towns separated by a hundred miles could have local times that differed by several minutes.
People relied on local time until after the development of the telegraph and the railroad in the 1800’s. Trains could travel between places with noticeably different local times in a couple of hours, and telegraphs crossed these distances nearly instantly. Using a different local time for each place made it nearly impossible to maintain communications and railroad schedules.
In the United States and other countries, the railroad industry promoted the use of standard time. In this system, all towns in a certain region, called a time zone, share the same time. Further, the time in two adjacent time zones differs by exactly one hour. Time signals sent by telegraph would enable distant places to synchronize their clocks, replacing the practice of setting clocks by the sun. Railroads in the United States adopted this system in the 1880’s, and despite objections from a variety of people, it quickly became the informal standard for most of the country. In 1918, the U.S. Congress formally enacted the system of time zones used in the United States today. Around the same time, nations around the world began to adopt standard time systems.
International time zones.
As standard time became widespread, governments and shipping companies worked to develop a uniform system of international time zones. Encouraged by the development of radio communications and airplane travel, nearly all nations had adopted the current system by 1950.
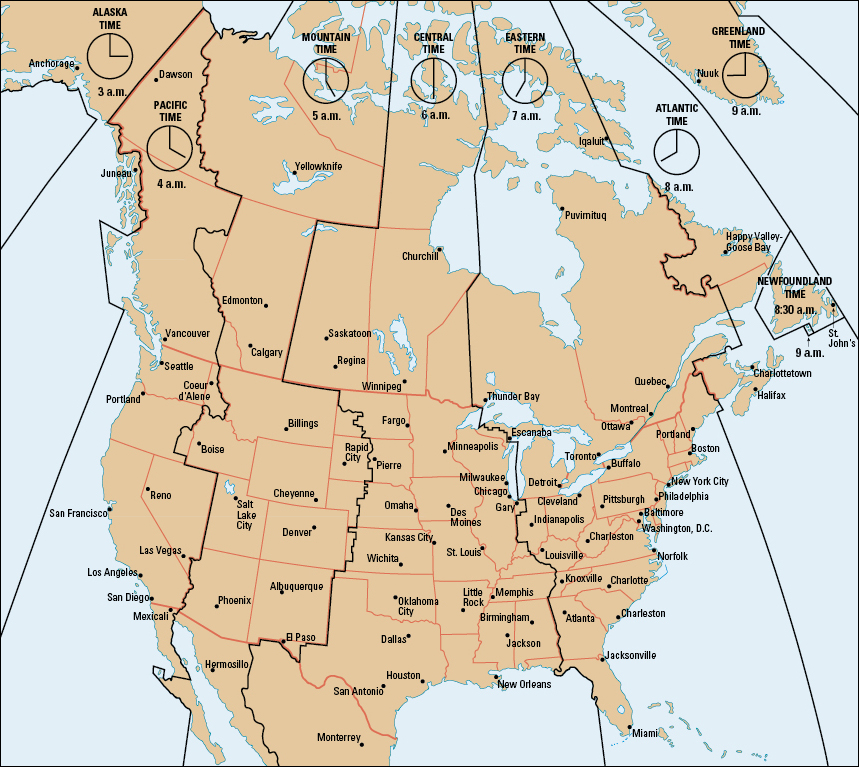
The international system of time zones takes as its reference point the prime meridian, the north-south line designated as 0° longitude that runs through Greenwich, England. All other places derive their time from the time at the prime meridian. Moving east, each successive zone adds one hour to the time. Traveling west, each zone subtracts one hour. Standard time zones generally span about 15 degrees of longitude each. However, their borders zigzag somewhat to avoid dividing individual communities or regions into different time zones. Some nations deviate from the standard system.
A line called the International Date Line helps the international system of time zones to function properly. Under the system, a traveler flying west subtracts one hour from the clock for each time zone crossed. If the traveler moved fast enough, eventually the clock would return to the previous day. To counteract this problem, travelers add a full day when crossing the International Date Line from east to west. Likewise, they subtract a full day when passing in the opposite direction. The International Date Line runs opposite the prime meridian, roughly following the meridian of 180° longitude.
Coordinated Universal Time,
abbreviated UTC, serves as the standard international time. By definition, UTC is the time at the prime meridian as measured by atomic clocks. Other time zones derive their time by adding or subtracting a whole number of hours to UTC.
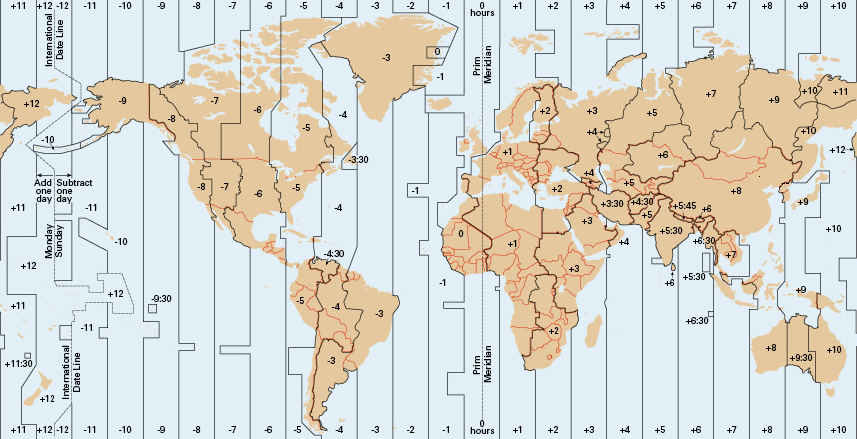
Although UTC relies on atomic clocks rather than the motion of the sun, scientists work to keep UTC synchronized with solar time. They occasionally add or subtract a leap second to make sure that UTC and solar time never differ by more than 0.9 second.
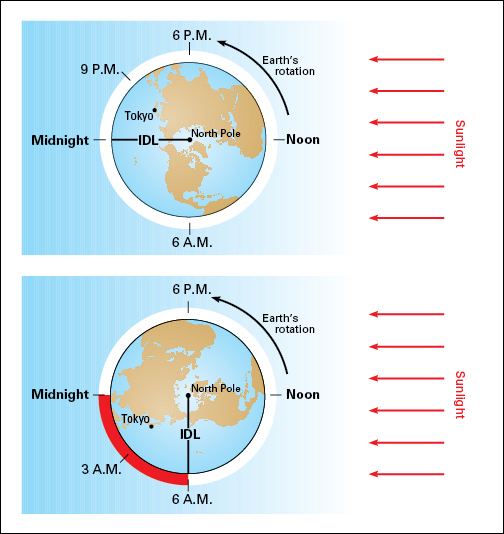
Our understanding of time
As you read this sentence—right now—you are experiencing the present. Now, the event of reading the last sentence is already fading into the past, and new events are becoming part of the present.
The simple act of reading the previous paragraph reveals three important features of our experience of time. First, we sense that the present is somehow special. The present moment seems more vivid or even more real than other times. Second, we tend to think of time as moving or flowing, with present events continuously updating themselves. Third, we seem able to remember the past but not the future. As you read the second sentence, you remembered the first sentence but had no memories of the sentences that followed.
A fourth aspect of our experience of time is that time often appears to flow at a varying rate. For example, an hour of a dull class may seem much longer than an hour of playing outside, even if a clock shows that both activities lasted the same amount of time.
These seemingly simple characteristics lead to difficult questions about time. For instance, do moments of time “flow” by, and if so, how? Why does time seem to proceed in only one direction, and is it possible to move backward in time? Are the past, present, and future really different? The search for answers to such questions has helped scholars to refine their ideas about time.
Time in classical physics.
To aid in his studies of motion, the English scientist Sir Isaac Newton assigned various precise properties to time. In doing so, Newton became perhaps the first person to propose a detailed formal model of time. Newton considered moments of time to be similar to the numbers on a number line. In such a model, one moment comes after another in sequence. At least one moment exists between any other two moments, and time has no beginning or end. Despite our perceptions, Newton proposed that the “flow” of time is uniform—that is, that one hour lasts as long as any other. He warned against confusing our perception or measurement of time with the passage of time itself.
Newton’s view relied on two seemingly reasonable assumptions that later proved controversial. First, it assumed that motion did not affect the passing of time. For example, suppose Alice and Bob left school at the same time. Alice went to soccer practice, while Bob visited the library. They met again at dinner. In Newton’s view, Alice and Bob would experience the same amount of time between school and dinner despite their separate paths.
Second, Newton’s view assumed that all observers could agree on whether two events were simultaneous—that is, whether they happened at the same time. If one person observed Alice scoring a goal just as Bob dropped a book, then all other witnesses would agree that the two events were simultaneous.
In some ways, Newton’s view of time resembles a deck of cards. Each card represents the universe at one moment and contains all the simultaneous events occurring at that moment. When the cards are placed in order, the deck represents our experience of time. Just as the deck can be divided easily into cards, all observers can agree on the moment at which each event happens.
Time in relativity theory.
In the early 1900’s, the German-born physicist Albert Einstein developed a new branch of physics called relativity theory. His work revised many basic ideas about time. Perhaps most importantly, relativity theory holds that space and time are not fundamentally separate features of reality. Instead, they compose a four-dimensional array of events called space-time. In space-time, observers measure space and time differently depending on their state of motion. See Relativity (Special relativity); Fourth dimension.
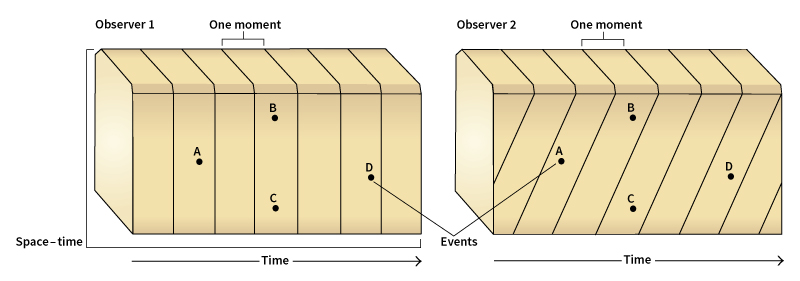
Relativity theory overturned Newton’s assumptions. First, it showed that the amount of time between events depends on an observer’s motion through space. If Alice and Bob leave school, go their separate ways, and meet again for dinner, they will not necessarily experience the same amount of time between school and dinner.
Second, observers can disagree on whether events are simultaneous. Consider Alice’s goal and Bob’s dropping of the book. A person in one state of motion—perhaps sitting on the sidelines—may witness the two events occurring simultaneously. A person flying overhead in an airplane, on the other hand, might see Alice score the goal before Bob drops the book. A person flying in the opposite direction could see the goal being scored after the book is dropped. In relativity theory, all three observations can be correct. The observers’ motion merely causes them to experience time differently.
At relatively low speeds, motion has an extremely small effect on time, so Alice, Bob, and the others probably would not notice the difference. However, experiments have confirmed the predictions of relativity theory. For example, atomic clocks traveling on supersonic jet planes tick more slowly than do clocks on the ground. In a sense, time seems to pass more slowly for a moving object than for an object at rest. This “stretching” of time, called time dilation, can result from motion or from the gravitational pull of a massive object.
If time in classical physics is like a deck of cards, the space-time of relativity more closely resembles a freshly baked loaf of bread. Just as the deck can be divided into cards, the loaf can be cut into “slices” representing moments. However, different observers can disagree as to which events happen at each moment, so there is no single correct way to slice the bread.
Time travel.
Relativity theory appears to allow for various kinds of time travel. Imagine that Alice left Earth for a round trip in a high-speed rocket. Because of time dilation, Alice would age slower than would Bob, who stayed on Earth. Depending on the rocket’s speed, Alice might experience only 10 years of travel but return to find 50 years had passed for Bob. In a sense, Alice would have “traveled” into Bob’s future.
According to relativity theory, travel into the past may also be possible. The theory states that gravity represents a curvature in space-time. The mathematics of relativity allows for many kinds of space-time. Some theoretically possible space-times can become so curved that an observer moving into an event’s future could eventually arrive at times in the event’s past. Other potential space-times offer a traveler even greater freedom. With enough energy, an observer in such a space-time could travel from any event to any other event, regardless of whether the destination lies in the past, present, or future.
Scientists do not know whether travel into the past is possible in our space-time, but the idea poses challenges for our understanding of reality. Suppose, for example, that Bob traveled into the past and killed his grandfather, preventing one of his parents from being born. Bob would not have been born either, but then who would have killed the grandfather? Scientists and philosophers call this famous dilemma the grandfather paradox. The paradox does not imply that time travel is impossible, but it does seem to prevent a time traveler from performing certain actions while visiting the past.
Arrows of time.
The basic laws of physics do not require that time proceed in a particular direction. If a particle can move from left to right, for example, it can also move right to left. When the first motion is viewed with time reversed, it appears identical to the second motion. In basic physics, these two scenarios cannot be distinguished. The same laws hold true whether time proceeds in one direction or the other.
In our everyday lives, on the other hand, time seems to proceed in a set direction. Imagine watching a film in which a dropped teacup shatters into fragments. This might strike you as a common scene. Now picture the film playing in reverse. You probably would be surprised to see the fragments assemble themselves into a teacup. Although the same basic laws hold true for both actions, experience tells us that the action in the second film violates time’s usual progression.
How does time’s apparent one-way flow arise from basic laws that do not favor a particular direction? This question ranks as one of the most enduring mysteries of time. In studying this puzzle, scientists and philosophers have identified many processes like the breaking of a teacup that seem to proceed in only one direction. These arrows of time indicate the direction in which time proceeds and help explain what distinguishes the past from the future.
Many arrows of time appear in our daily experiences. The arrow of memory arises from our ability to remember our past but not our future. The arrow of knowledge extends this distinction to facts outside our personal experience. For example, we can know with near certainty that dinosaurs lived 200 million years ago, but we have no idea what creatures will thrive 200 million years from now. The observation that causes precede their effects gives rise to the arrow of causality. We tend to believe that we can change the future but not the past. This distinction is sometimes called the arrow of change.
Other arrows of time come from the sciences. Perhaps the most important arrow arises from the second law of thermodynamics. According to this law, the entropy of a closed system will always increase. Entropy is a measure of the amount of a system’s energy that is unavailable for work. In a sense, the available energy of a system “runs down” as entropy increases. The system may also appear to grow more disordered.
The arrow of entropy explains why ice water warms to room temperature, gas spreads throughout its container, and cream mixes into coffee. In all of these processes, entropy increases with time. The arrow of entropy can also explain why shattered fragments do not assemble themselves into teacups. Such a scenario would result in an overall decrease in entropy, violating the second law of thermodynamics.
Scientific investigation has revealed other arrows of time. Light and other radiation spread outward from a given source but never come back together, giving rise to the arrow of radiation. The cosmological arrow arises from the fact that the universe continually expands.
Scientists and philosophers do not know for sure how the various arrows of time relate to one another. Some scholars have suggested that most or all of the other arrows derive from the arrow of entropy.
The past, present, and future.
In common experience, the present seems somehow more real than the past or future. The past feels somewhat real in the sense that we can remember it and observe its effect on the present. However, it does not surround us as the present does, and we do not experience it as vividly. To most people, the future seems even less real because we cannot know it and our choices may alter it. These observations have led some scholars to conclude that only the present and perhaps the past can be said to exist.
Relativity theory casts doubt on the distinction between past, present, and future. In relativity theory, observers cannot always agree on which events make up a single moment. Thus, it makes little sense to insist that a unique moment called the present exists. What one observer experiences as the present can include events from another observer’s past, present, and future.
In fact, relativity theory appears to imply that the past, present, and future actually exist as an unbroken four-dimensional “block” of space-time. In this view, the future is somewhat like a distant city to which we are traveling—it exists even though we have not reached it yet. Scholars are not quite sure how to reconcile this idea with the widely held notion that our actions and choices can alter the future.