Uranium is a radioactive chemical element. It is a silvery-white metal that turns dark gray when exposed to air. Uranium has the second largest atom found in nature. Only the atom of the extremely rare element plutonium is larger. Uranium is the source of energy used to generate electric energy at all large commercial nuclear power plants. A chunk of uranium the size of a softball can release more energy than a trainload of coal that weighs 3 million times as much. Uranium also produces the tremendous explosions of certain nuclear weapons.
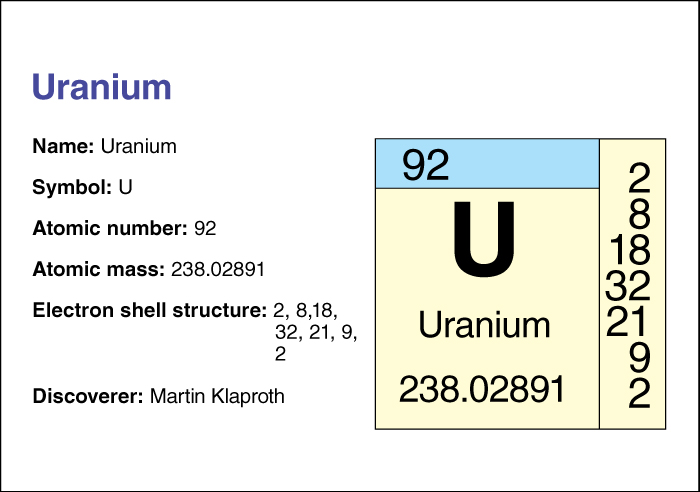
Like most metals, uranium can be bent and shaped. Many uses of uranium are based on its high density. A piece of uranium is 1.5 times as heavy as a piece of lead of the same size. Engineers put uranium to use in a number of applications. They use uranium in gyrocompasses for aircraft, as a counterweight for ailerons and other control surfaces of aircraft and spacecraft, and as a radiation shield. The uranium used in these applications has an extremely low level of radioactivity. Scientists also use uranium to determine the age of rocks and ground water, and of deposits of travertine (a form of limestone) at archaeological sites.
For information on the position of uranium on the periodic table, see the article Periodic table.
Uranium occurs chiefly in rocks, usually in extremely small concentrations. On average, uranium accounts for about 3 pounds of every million pounds of Earth’s crust and only 3 pounds of every billion pounds of seawater.
In 1789, the German chemist Martin H. Klaproth discovered uranium. He found it in pitchblende, a dark, bluish-black mineral. Klaproth named uranium after the planet Uranus, which had been discovered in 1781. In 1841, French chemist Eugène Péligot separated pure uranium from pitchblende.
Sources of uranium
Pitchblende, the first uranium ore discovered, is the most important variety of uraninite. Other major ores include uranophane, coffinite, and carnotite. Sandstone, shale, and phosphate may contain valuable deposits of uranium ores. Granite usually has small amounts of uranium.
In the early 2020’s, the world’s mining companies had about 6,700,000 tons (6,100,000 metric tons) of uranium reserves that could be mined at a reasonable cost. World production of uranium is about 53,000 tons (48,000 metric tons) a year. Kazakhstan leads all countries in production. Canada, a leading uranium producer, mines uranium from the Athabasca Basin of northern Saskatchewan. The chief deposits of uranium ore in the United States are in Arizona, Colorado, New Mexico, Texas, Utah, and Wyoming. Smaller deposits exist in Montana, Nebraska, and Washington.
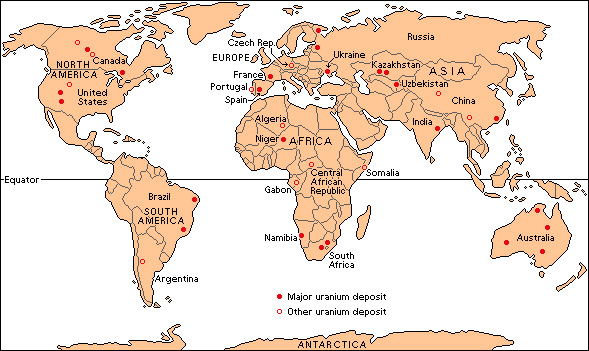
Uranium isotopes
In nature, uranium occurs in three isotopes (forms). Each has an atomic number (number of protons in the nucleus) of 92. Each isotope, however, has a different number of neutrons and so differs in its atomic mass number (total number of protons and neutrons in the nucleus). The lightest natural isotope has 92 protons and 142 neutrons, for a total of 234 nuclear particles. The name of this isotope, U-234, comes from the chemical symbol for uranium—U—and the atomic mass number. The other two natural isotopes of uranium are U-235 and U-238. These isotopes have 143 and 146 neutrons, respectively.
U-238 makes up about 99.28 percent of all natural uranium. U-235 accounts for approximately 0.71 percent of all natural uranium; U-234, only about 0.006 percent.
U-235 is the only natural isotope of uranium whose nucleus can easily be made to undergo fission—that is, to split into two nearly equal parts. The fission process releases the nuclear energy used in power plants and weapons.
Properties of uranium
Uranium has a relative atomic mass of 238.02891. An element’s relative atomic mass equals its mass (amount of matter) divided by 1/12 of the mass of an atom of carbon 12, the most abundant form of carbon. At 25 °C, uranium’s density is 19.05 grams per cubic centimeter (see Density). Uranium melts at 1132 °C and boils at 3818 °C. It belongs to the group of elements known as the actinide series (see Element, Chemical [Periodic table]).
Uranium combines readily with other elements. In nature, uranium always occurs in chemical compounds with oxygen. In most surface and ground waters, uranium is combined with oxygen, carbon dioxide, phosphate, fluoride, or sulfate. In addition, uranium reacts with acids to form compounds called uranyl salts. All uranium compounds are highly poisonous.
Radioactivity.
All isotopes of uranium are radioactive. Their nuclei decay (break apart), releasing particles and energy, chiefly alpha particles, beta particles, and gamma rays. When an isotope decays, it turns into another isotope. A succession of decays eventually changes uranium into a lead isotope that is not radioactive.
Scientists measure the rate at which an isotope decays in terms of its half-life. The half-life of an isotope is the length of time after which only half the atoms of what began as a sample of that isotope would still be atoms of that isotope.
Uranium isotopes have long half-lives. The half-life of U-238 is about 41/2 billion years. U-235 has a half-life of about 700 million years; U-234, about 250,000 years. Much of the internal heat of Earth is thought to be a result of radiation given off by uranium.
Fissionability.
A U-235 nucleus can split into two fragments when struck by a neutron. When this nucleus splits, it releases energy. It also releases two or more neutrons. These neutrons, in turn, can cause other U-235 nuclei to break apart. When the other nuclei undergo fission, they also release energy and neutrons. Under certain circumstances, this process can continue in a self-sustaining series of fission events called a chain reaction.
A U-238 nucleus rarely breaks apart when struck by a neutron. Usually, U-238 nuclei merely absorb neutrons that strike them.
How uranium is mined and processed
Mining uranium.
Mining companies use three chief methods to remove uranium from the ground: (1) in situ solution mining, (2) open-pit mining, and (3) underground mining.
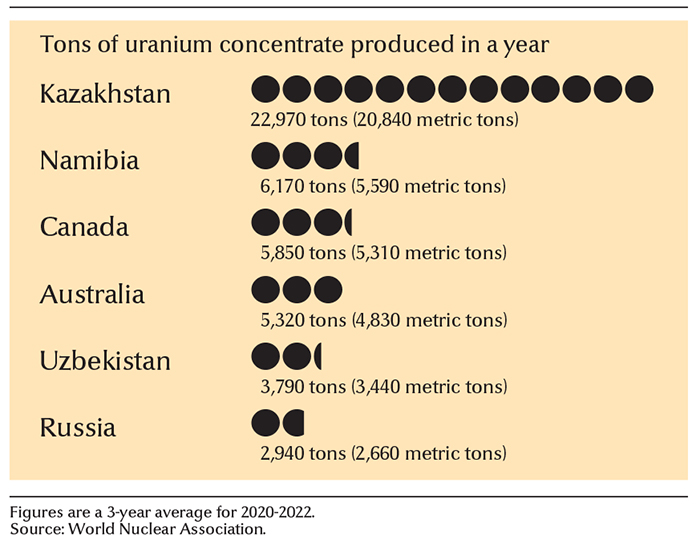
In situ solution mining
begins with the pumping of a special solution through holes drilled into the earth. The solution dissolves oxides of uranium. The solution containing the oxides is then pumped into tanks at the surface.
In almost all cases, the holes used for in situ solution mining have already been drilled as part of an effort to locate rich deposits of uranium. During the exploratory process, prospectors lowered radiation detectors into the holes.
Open-pit mining
uses explosives to break up rock and soils that cover uranium deposits near the surface of the earth. Miners dig blast holes, then fill them with the explosives. After the explosions, huge power shovels clear away the rubble. Smaller shovels then dig out the uranium ore.
Underground mining
is used if the uranium ore lies far beneath the surface. Mining companies dig tunnels into the deposits. The miners drill into the tunnel walls to install explosives that loosen the ore. The miners then load the ore into buckets, which are hoisted to the surface.
Refining and processing uranium ore.
The ore from the mine goes to a mill, where the uranium is concentrated. At the mill, workers use sulfuric acid or carbonate solutions to produce a uranium salt called yellowcake. The salt is purified to an oxide, also called yellowcake. At a conversion plant, the oxide undergoes a chemical reaction with fluorine. This reaction produces uranium hexafluoride.
The uranium hexafluoride goes to an enrichment plant, where U-235 and U-238 are separated from each other. This separation produces enriched uranium, which has a higher percentage of U-235 than does natural uranium. Most nuclear reactors at power plants in the United States use fuel that contains about 2 to 4 percent U-235. Nuclear weapons and the reactors for nuclear-powered ships require uranium with much higher concentrations of U-235.
Enriched uranium for reactors goes to a fuel-fabrication plant, where the uranium hexafluoride is converted to uranium dioxide. The uranium dioxide is compressed into cylindrical pellets that are used as fuel.
Separating uranium isotopes.
Scientists have invented several methods for separating uranium isotopes. Enrichment plants use two of these methods, gaseous diffusion and centrifugal enrichment. A third method, laser isotope separation, is still experimental.
The gaseous diffusion method
is used in the United States. In this process, a pump forces molecules of uranium hexafluoride gas through barrierlike structures. These structures have millions of tiny holes in them.
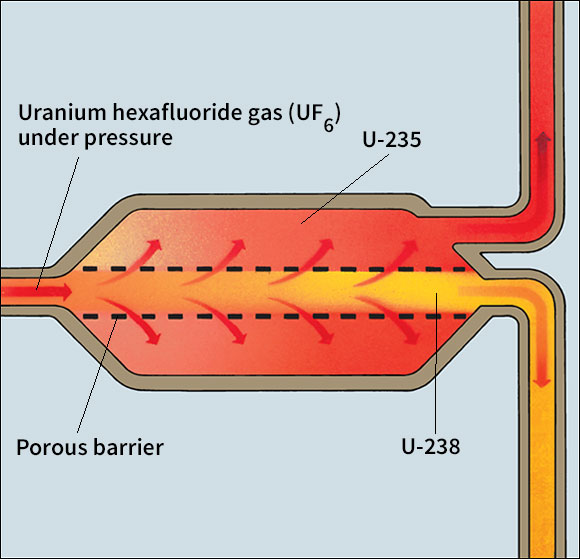
Lighter gas molecules pass through the holes in the barriers more rapidly than do heavier molecules. The lighter molecules contain U-235 atoms. As a result, gas that passes through the barrier contains a higher percentage of U-235 than did the original gas. The increase in concentration is extremely small, however. The gas must pass through the barrier several thousand times to produce enriched uranium for a power plant.
The centrifugal method
is used in several plants in Europe and Japan. The centrifuge in this process consists of vertical cylinders that spin rapidly. Pumps force uranium hexafluoride gas into each cylinder through a stationary vertical tube in the center of the cylinder.
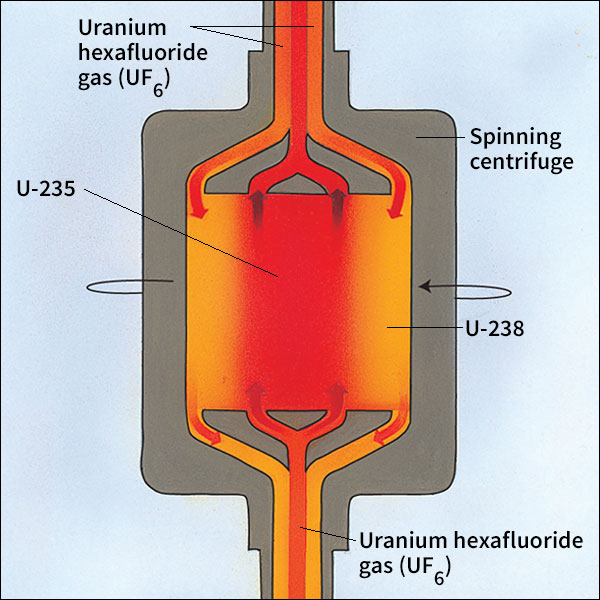
The spinning of a cylinder forces almost all the gas outward to the curved walls. In addition, a scoop connected to the bottom of the stationary tube helps create a vertical flow in the gas. Differences in temperature within the cylinder also contribute to the flow of the gas.
Due to these three influences—the spinning of the cylinder, the action of the scoop, and the temperature differences—the gas flows in a complex pattern. As a result, the gas near the bottom of the cylinder becomes more concentrated in U-238 than does the gas at the top.
The scoop at the bottom removes waste gas, which has a relatively high concentration of U-238. A scoop at the top removes enriched gas, which has a relatively high concentration of U-235. The process repeats until the desired concentration of U-235 is obtained.
Laser isotope separation
uses a combination of laser light and electric charge to separate uranium isotopes. A laser produces a thin beam of light that has a very narrow range of frequency (rate of vibration of the light waves).
In atomic vapor laser isotope separation (AVLIS), a beam of electrons heats a piece of uranium at the bottom of a closed container. The heat changes the uranium into vapor (gas). A laser beam then pulses into the vapor. The frequency of the beam is tuned so that electrons in atoms of U-235 can absorb the light, but U-238 electrons cannot.
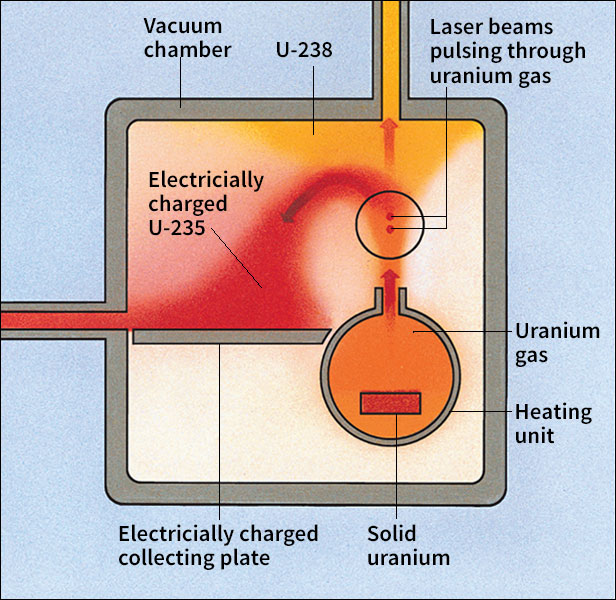
When a U-235 electron absorbs this light, it gains enough energy to leave the atom. This process changes the electrical balance of the atom. An electron carries a negative electric charge. A nucleus carries one or more positive charges. In a normal atom, the number of positive charges is the same as the number of negative charges. Thus, when an electron leaves an atom, the atom acquires a positive charge. Scientists say that the atom becomes a positive ion. Thus, the laser light ionizes U-235 atoms, but not U-238 atoms.
The hot vapor rises. Negatively charged collector plates near the top of the container attract the positive U-235 ions. Because the collector plates are cooler than the gas, the U-235 condenses (changes from a gas to a liquid) on them. The U-235 liquid drips off the collector plates into special containers, forming a solid mass called a splat. The splats are collected, then purified and oxidized for use as nuclear fuel.
Meanwhile, the electrically neutral U-238 travels past the charged plates. It then condenses on a waste plate near the top of the container.
In another laser technique, an electric unit heats a piece of uranium, producing a vapor. Two laser beams work together to ionize U-235 atoms in the vapor. A positively charged plate collects U-235 ions, while a vapor of U-238 atoms exits through an opening in the top of the container.
Laser isotope separation uses much less electric energy than does gaseous diffusion. In addition, the separation equipment costs much less than centrifuge equipment. Government-sponsored companies in France, Japan, and the United States are experimenting with laser isotope separation.
History of the use of uranium
People have used uranium and its compounds for almost 2,000 years. Colored glass produced for a mosaic mural about A.D. 79 contains uranium oxide, and glass manufacturers continued to use this compound as a pigment until the 1800’s. People who painted or glazed china also employed uranium as a pigment. In addition, the metal was used in the processing of photographs.
In 1896, French physicist Antoine Henri Becquerel discovered that uranium is radioactive. His achievement marked the first time that any element had been found to be radioactive.
In 1935, Arthur J. Dempster, a Canadian-born physicist, discovered U-235. German chemists Otto Hahn and Fritz Strassmann used uranium to produce the first artificial nuclear fission in 1938. In 1942, Italian-born physicist Enrico Fermi and his co-workers at the University of Chicago produced the first artificial chain reaction. They used U-235 as the fissioning material. Fermi’s work led to the development of the atomic bomb.
Scientific research also led to peacetime uses of uranium. In 1954, the U.S. Navy launched the Nautilus, the first submarine powered by nuclear fuel. In 1957, the first nuclear power plant in the United States began to operate in Shippingport, Pennsylvania, near Pittsburgh.
Since the early 1970’s, uranium-fueled nuclear power plants have been an important source of energy. About 30 countries now have such plants. Several of these countries continue to build them.