Weather is the state of the atmosphere at some place and time. We describe the weather in many ways. For example, we may refer to the temperature of the air. We report whether the sky is clear or cloudy. We describe how hard the wind is blowing. We say whether it is raining or snowing. At any given time, the weather is fair in some places, while it rains or snows in others. In some places it is warm. In others, it is cold.
Earth is not the only planet with a variety of weather conditions. All of the planets in the solar system larger than Mercury have enough of an atmosphere to support weather systems. In addition, the moons Titan and Triton have such an atmosphere. The remainder of this article discusses the weather on Earth.
Loading the player...Tornado
The weather affects our lives every day. For example, it can have an impact on what type of clothing we wear. It can influence how we spend our free time. Weather also affects agriculture, transportation, and industry. Freezing temperatures can damage crops in citrus-growing regions, causing a rise in the price of oranges. Winter snows often create hazardous driving conditions. Thick fog may slow traffic on the roads. Fog may also cause delays at airports. Our use of air conditioning during heat waves and heating during cold weather means that utility companies must supply more power at those times. Severe weather, such as tornadoes, hurricanes, and blizzards, can damage property and take lives.
Scientists who study the atmosphere and the weather are called meteorologists. Because of weather’s importance, meteorologists have developed ways to forecast weather conditions. Forecasts for the next 12 to 24 hours are correct more than 80 percent of the time. Long-range forecasts for the next week or month are less accurate. These forecasts indicate general trends. For example, they may say whether temperatures are expected to be warmer or colder than normal.
Closely related to weather is climate. Climate is the weather of a place averaged over a length of time. Scientists determine a region’s climate by examining its average monthly and annual temperature and precipitation. They also study a region’s vegetation. Earth’s surface is a patchwork of climate zones. For example, in various parts of Earth, we find deserts; tropical rain forests; prairies; and forests of cone-bearing trees. We also find frozen, treeless plains; and coverings of glacial ice. Unlike changes in the weather, which can occur in minutes, climate changes take many years.
What causes weather
Weather takes place in the atmosphere, the layer of air that surrounds Earth. Air is a mixture of gases and tiny suspended particles.
The most plentiful gas is nitrogen. Nitrogen accounts for about 78 percent of the air we breathe. The second most plentiful gas is oxygen, at 21 percent. The remaining 1 percent consists of a variety of gases. In spite of their low concentrations, some of these gases play vital roles. For example, the atmosphere contains little water vapor. This vapor is an invisible gas produced when water evaporates. Yet, without water vapor there would be no clouds, no rain, no snow, and no plants or animals.
Most of the tiny particles floating in the atmosphere are too small to be visible. They are solid or liquid and come from a number of sources. Sources of particles include the wind erosion of soil, volcanic eruptions, and the release of pollutants by smokestacks and automobile tailpipes.
Most weather occurs in the lowest portion of the atmosphere, called the troposphere. The troposphere extends from Earth’s surface up to an altitude of about 6 to 12 miles (10 to 19 kilometers). Three key factors that determine the weather in the troposphere are air temperature, air pressure, and humidity.
Air temperature
is a measure of the energy of motion of the air’s gas molecules. The factors most responsible for the heating and cooling of the atmosphere are radiation arriving from the sun and radiation flowing from Earth.
The sun continually sends energy into space as electromagnetic radiation. One kind of solar radiation is visible light. The other forms of solar electromagnetic radiation are invisible to human beings. They include infrared (heat) rays and ultraviolet rays. About 30 percent of the solar radiation that reaches the atmosphere is reflected back into space, mostly by clouds. The atmosphere and Earth’s surface absorb the remaining 70 percent, becoming warmer.
The warmed Earth cools by radiating infrared rays. Some of this radiation travels directly into space. The atmosphere absorbs almost all the remainder as it streams off the surface of the planet. This absorption of radiation is known as the greenhouse effect. It makes the air near Earth’s surface about 59 Fahrenheit degrees (33 Celsius degrees) warmer than it would be otherwise.
The atmosphere also sheds heat energy by radiating infrared rays. Some of this infrared radiation flows down to the surface. The remainder travels out into space.
Air temperature varies from day to night and from season to season because of changes in the amount of radiation heating Earth’s atmosphere. For example, days usually are warmer than nights. The reason is that Earth receives the heating rays of the sun only during the day. At night, infrared radiation from the planet streams off into space, and the air temperature drops.
Air temperature also changes with the seasons. Except near the equator, summers are warmer than winters. In the summer, the sun is higher in the sky. When the sun is higher above the horizon, the intensity of the sunlight striking Earth’s surface increases. Days are longer. More hours of sunlight in summer also mean more solar heating. At the equator, however, temperatures remain constant the year around.
Altitude also affects air temperature. Within the troposphere, the air temperature generally drops 3.5 Fahrenheit degrees per 1,000 feet of elevation (6.5 Celsius degrees per 1,000 meters of elevation). Thus, it is usually colder on top of a mountain than in the surrounding lowlands.
Air pressure
is the weight per unit of area of a column of air that reaches to the top of the atmosphere. As you move higher, there is less and less air above you. For this reason, air pressure decreases with increasing altitude. Air pressure is, on average, highest at sea level. It drops to about half its sea-level value at an average altitude of about 18,000 feet (5,500 meters).
Air pressure also changes from place to place across Earth’s surface. Part of this change is due to differences in land elevation. Most of the remainder is caused by changes in air temperature. Cold air is dense. That is, it has more air molecules per unit volume. As a result, it exerts high pressure. Warm air is less dense and exerts low pressure.
Regions where air pressure is high experience fair weather. Regions where air pressure is low experience cloudy, stormy weather. Generally, the weather stays fair or improves if air pressure rises. If the air pressure falls, however, the weather may turn cloudy and rainy or snowy.
Air moves from areas where the air pressure is high toward areas where the air pressure is low. This movement of air is what we call wind.
Humidity
is a measure of the amount of water vapor in the air. There is an upper limit to this amount. Air that contains its maximum amount of water vapor is described as saturated. The amount of water vapor the air can hold increases as the air temperature rises. The capacity decreases as the temperature falls. Thus, saturated warm air has more water vapor than saturated cold air.
Weather reports describe the amount of water vapor in the air in terms of the relative humidity. Relative humidity compares the amount of water vapor in the air with the amount of water vapor at saturation. It is expressed as a percentage. If the relative humidity is 50 percent, the amount of water vapor in the air is half of what it would be if the air were saturated. Lowering the air temperature increases the relative humidity.
If the relative humidity is 100 percent, the air is saturated. When air becomes saturated, water vapor begins to condense into droplets of water. Condensation is the opposite of evaporation. It is a change from a gas to a liquid. If the air is cold enough, at saturation the water vapor develops into tiny ice crystals.
If condensation occurs on a cold surface, such as the outside of a window at night, dew or frost forms. Dew and frost do not fall from the sky like rain or snow. Rather, they form when air in contact with a cold surface is chilled to saturation. The same process occurs when small drops of water appear on the outside of a cold soft-drink can on a hot day. The temperature to which air must be cooled to reach saturation and produce dew is known as the dew point.
Loading the player...Dew
When saturation occurs within the atmosphere, water vapor condenses into droplets or into ice crystals that form clouds. A cloud in contact with Earth’s surface is known as fog. Most clouds occur within the troposphere. Because air temperature drops with increasing altitude within the troposphere, high clouds are cold. They consist mostly of ice crystals. The crystals give these clouds a fuzzy appearance. Low clouds are warmer. They generally are composed of droplets. These clouds appear to have sharper edges.
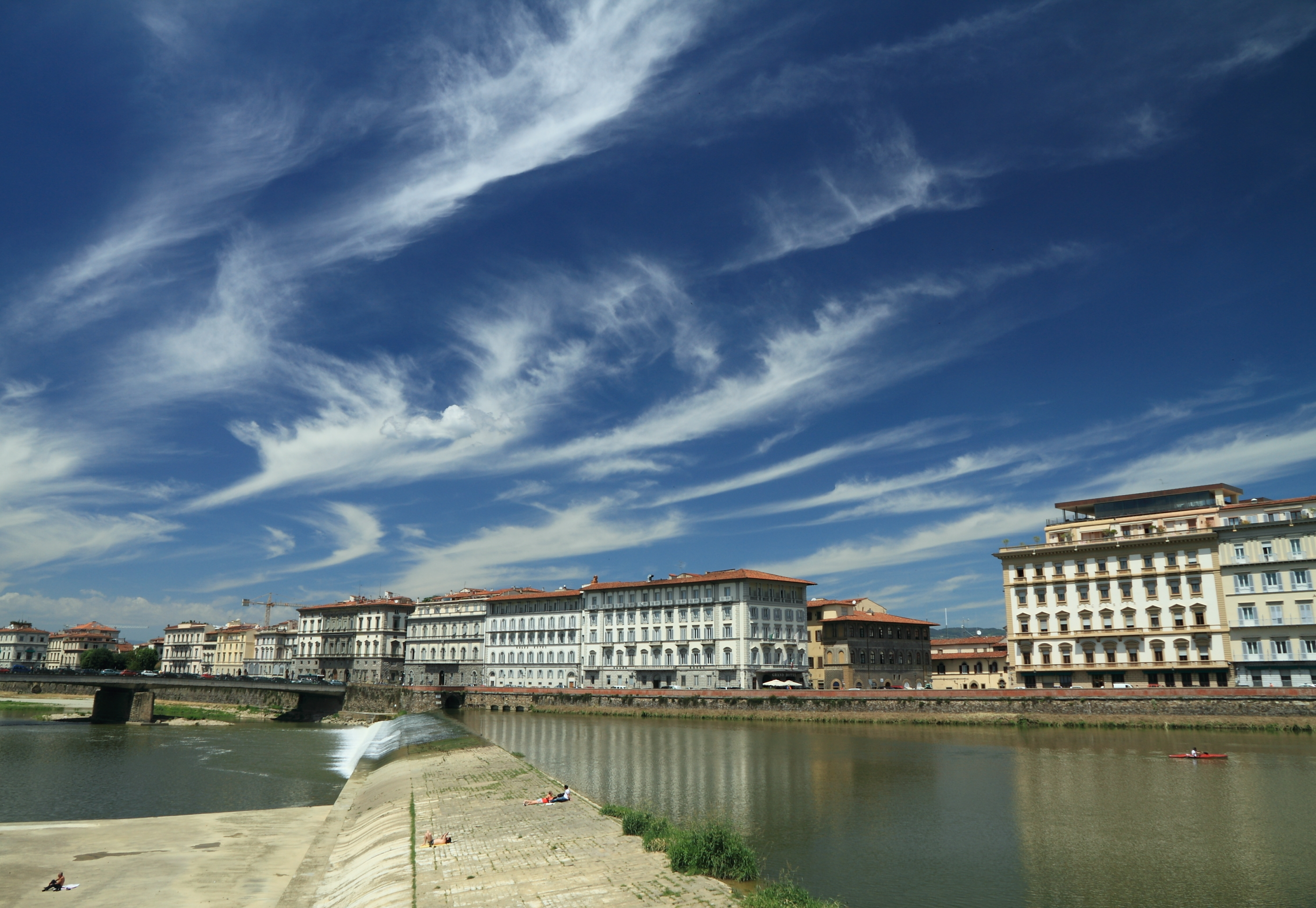
Clouds form where air moves upward. As air ascends, it encounters lower pressure. Air responds to lower pressure by expanding. Whenever gases expand, they cool. As air cools, its relative humidity increases until it reaches saturation and clouds form.
Where air moves downward, clouds usually do not develop. Descending air is compressed. It warms up. Its relative humidity decreases. Saturation is not possible, and so clouds do not form.
Weather systems
Meteorologists classify weather systems according to their size and how long they last. The two largest and longest-lasting types of systems are planetary-scale systems and synoptic-scale systems. Planetary-scale systems are the belts of winds that circle the globe. They may blow in the same direction for weeks at a time. Synoptic-scale systems cover a portion of a continent or ocean. They last up to a week or so. The term synoptic comes from a Greek word meaning a general view.
Two briefer and smaller types of systems are mesoscale systems and microscale systems. Mesoscale systems may last an hour or less. They are so small they may affect the weather of only part of a city. Examples include thunderstorms and sea breezes. Microscale systems, such as tornadoes, last only minutes. They affect an area not much larger than a few football fields.
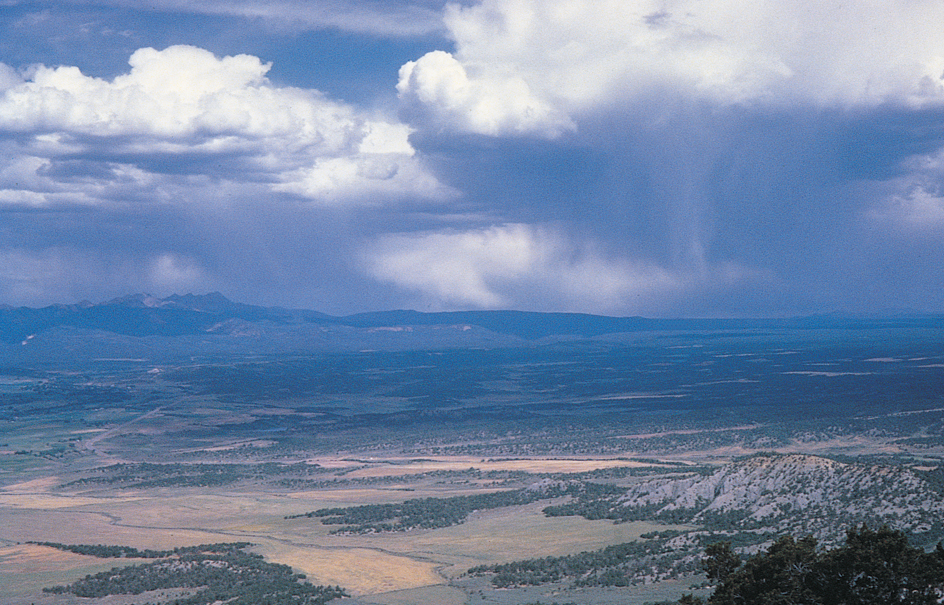
Planetary-scale systems.
Suppose that Earth did not rotate and that the noon sun was always directly above the equator. Air temperatures would be highest at the equator. Temperatures would decrease toward the poles. Cold air is denser than warm air. Thus, air pressure would be higher at the poles and lower at the equator. Because air moves from areas of high pressure to areas of low pressure, cold air would sweep toward the equator. There it would push the warm air upward. In the upper atmosphere, the warm air would move toward the poles, cool, and sink over the poles. Thus, the planetary-scale circulation of wind would consist of two huge cells, one in each hemisphere.
The real Earth rotates. Rotation of Earth on its axis causes winds that blow thousands of miles or kilometers to shift direction gradually. This shift is known as the Coriolis effect. The Coriolis effect causes winds in the Northern Hemisphere to shift to the right. It causes winds in the Southern Hemisphere to shift to the left. In the Northern Hemisphere, for example, winds blowing southward shift to the west. Winds blowing northward shift to the east.
Rotation of the planet also causes winds near Earth’s surface to split into three belts in each hemisphere. These three belts are the trade winds, the westerlies (winds from the west), and the polar winds. The trade winds blow near the equator, between 30° north latitude and 30° south latitude. The westerlies blow in the middle latitudes between 30° and 60° north and south of the equator. The polar winds blow in the Arctic and Antarctic, from 60° latitude toward the poles.
Trade winds north of the equator blow from the northeast. South of the equator, they blow from the southeast. The trade winds of the two hemispheres meet near the equator, causing air to rise. Rising air cools. Its relative humidity therefore increases. Thus, a band of cloudy, rainy weather circles the globe near the equator.
Westerlies blow from the southwest in the Northern Hemisphere. They blow from the northwest in the Southern Hemisphere. Westerlies and trade winds blow away from the 30° latitude belt. Over broad regions centered at 30° latitude, surface winds are light or calm. Air slowly descends. Air warms as it descends. Its relative humidity decreases, making clouds and precipitation unlikely. As a result, fair, dry weather characterizes much of the 30° latitude belt.
Polar winds are easterlies (winds from the east). They blow from the northeast in the Arctic and from the southeast in the Antarctic. The boundaries where cold polar easterlies meet the mild westerlies are known as the polar fronts. A front is a narrow zone of transition, usually between a mass of cold air and a mass of warm air. Storms develop and move along the polar fronts. They bring cloudiness, rain, or snow.
Seasonal changes take place in Earth’s wind patterns. Wind belts shift toward the poles in spring and toward the equator in fall. For example, during the fall, the polar front in the Northern Hemisphere often moves from Canada down to the continental United States.
Planetary-scale winds control the direction of movement of smaller-scale weather systems. For example, in the tropics, trade winds steer hurricanes and other weather systems from east to west. In middle latitudes, westerlies move weather systems from west to east. The westerlies are particularly vigorous near the top of the troposphere just over the polar fronts. There, they form corridors of strong winds known as jet streams. The jet stream over a polar front supplies energy to developing storms. Then it moves them rapidly along the front.
Synoptic-scale systems
include air masses, fronts, lows, and highs. The movement of these systems causes the day-to-day changes in the weather of Europe, the continental United States, and other regions in the middle latitudes.
Air masses.
Across North America, warm air masses move north and northeastward. Cold air masses move south and southeastward. Maritime polar air is cool and humid. This air mass forms over the North Pacific and North Atlantic. It brings low clouds and precipitation to the Pacific Northwest, New England, and eastern Canada. Continental polar air dry and cold in winter and dry and mild in summer. This air mass forms in north-central Canada. Arctic air is dry and much colder than continental polar air. it forms over the snow-covered regions north of about 60° latitude in the Northern Hemisphere. The movement of Arctic air to the south causes the bone-numbing cold waves that sweep across the Great Plains and Northeast in winter.
Most of the maritime tropical air that invades North America originates over the Gulf of Mexico and the tropical Atlantic. This warm, humid air mass brings summer heat waves to areas east of the Rocky Mountains. Continental tropical air forms over the deserts of Mexico and the southwest United States. In summer, this hot, dry air mass surges over Texas and other parts of the American Southwest.
As an air mass travels, its temperature and humidity can change. For example, air over the Pacific Ocean west of North America is mild and humid. If that air mass moves eastward, it is forced up the slopes of the coastal mountain ranges. Air temperature drops. The relative humidity increases to saturation. Clouds form, and rain or snow develops. As the air travels down the opposite slopes of the mountain ranges, the air temperature rises. The relative humidity decreases. Clouds thin out or vanish.
This process repeats with each mountain range the air mass encounters as it moves eastward. By the time it reaches the Western Plains, the air mass has become drier and milder. This modified air mass is known as Pacific air. It brings mild, dry weather to much of the central and eastern regions of the United States and Canada.
Fronts
form where air masses meet. A front is a narrow zone of transition between air masses that differ in density. In most cases, the air masses differ in temperature. The fronts are either warm or cold.
A front that stalls is known as a stationary front. The weather along a stationary front often consists of cloudiness and light rain or snow.
Cold fronts move faster than warm fronts. As a result, a cold front may catch up to and merge with a warm front. The warm air is lifted off Earth’s surface. The merged front is known as an occluded front. An occluded front sometimes moves slowly and causes several days of cloudiness and light precipitation.
Lows
are areas of low air pressure. The winds in a low pressure system bring contrasting air masses together to form fronts. For this reason, lows are sometimes described as the chief weather-makers of regions in the middle latitudes. Scientists use the term cyclone to refer to a synoptic-scale low-pressure area. They also use the term to mean a hurricane in some parts of the world.
Viewed from above in the Northern Hemisphere, surface winds in a low-pressure area blow in a counterclockwise and inward direction. In the Southern Hemisphere, they blow clockwise and inward. Surface winds converging in the low cause air to rise, cool, and reach saturation. Clouds and precipitation develop. Air ascends mostly along fronts that develop as winds in the low bring cold and warm air masses together.
In North America, lows generally travel from southwest to northeast. They may complete a journey from Colorado to New England in three or four days. As a rule, temperatures are lower to the left (north) of the path followed by the low-pressure area and higher to the right (south). In winter, the heaviest snows usually fall about 90 to 150 miles (150 to 250 kilometers) to the north and west of the moving low-pressure area.
Highs
are areas of high air pressure. They are also known as anticyclones. A high, which brings fair weather, often follows in the wake of a low. Viewed from above in the Northern Hemisphere, surface winds in a high blow in a clockwise and outward direction. In the Southern Hemisphere, they blow counterclockwise and outward. As winds blow out and away from a high, air descends near the center of the system. Descending air warms. The relative humidity decreases.
Highs are either warm or cold. Warm highs form in the latitudes below the polar fronts. They are characterized by high temperatures and low relative humidities. Such highs are massive weather systems that extend from Earth’s surface to the top of the troposphere. In the summer, a warm high sometimes stalls over land. If the high remains stationary for several weeks, it can cause a drought.
Cold highs form in the latitudes above the polar front. They are shallow masses of cold, dry air. They develop mostly in the winter over snow-covered regions, such as northern Canada, Alaska, and Siberia. Cold highs that move into the middle latitudes can bring fair but cold weather.
Mesoscale and microscale systems
result from the development and movement of synoptic and planetary-scale systems. Mesoscale systems may last an hour or less and affect only part of a city. They include thunderstorms and sea breezes. A tornado is an example of a microscale system. A microscale system is the smallest and briefest of significant weather systems.
Measuring the weather
No single country can constantly measure and report on conditions in every part of the atmosphere. For this reason, the world’s nations must cooperate to monitor the weather effectively. To this end, they founded the International Meteorological Organization in 1873. It was renamed the World Meteorological Organization (WMO) in 1950. Members of the WMO participate in the worldwide observation of the atmosphere. They exchange weather data and forecasts. Weather observations come from many different sources. The sources include land-based observation stations, radar systems, weather balloons, airplanes, ships, and satellites.
Land-based observation stations.
Observation stations use a variety of instruments to monitor the state of the atmosphere. An electronic thermometer checks air temperature and registers the highest and lowest temperature of the day. A hygrometer measures the amount of water vapor in the air. A barometer shows the air pressure. A weather vane indicates the direction of the wind. An anemometer monitors wind speed. Rain gauges measure rainfall or snowfall.
Weather radar.
Some observation stations use radar to track storms. Weather radar can operate in either a reflectivity mode or a velocity mode.
In the reflectivity mode, weather radar locates areas of rain or snow. The system sends out a radar signal. The signal consists of microwave energy pulses. If the radar signal encounters rain, snow, or hail, the falling precipitation reflects some of that signal back to the radar. The reflected radar signal is called a radar echo. The radar echo appears as a blotch on a television-type screen. As the radar rotates, it generates a map of radar echoes that represents the pattern of precipitation surrounding the radar. In the reflectivity mode, weather radar can detect precipitation more than 250 miles (400 kilometers) away.
In the velocity mode, weather radar determines the circulation of air from the motion of raindrops, snowflakes, or dust particles. This radar is also known as Doppler radar. It uses the Doppler effect to calculate how the air in a weather system is moving.
Doppler effect
The Doppler effect is the change in frequency of sound or radiation waves caused by the motion of the source of the waves relative to their observer. For example, the pitch (frequency) of a train whistle seems higher as a train approaches. It sounds lower as the train moves away. Similarly, as raindrops, snowflakes, or dust particles move through the atmosphere, the radar signals they reflect change in frequency. The radar monitors these frequency changes. It then uses them to calculate the speed at which the drops, flakes, or particles are advancing or receding.
Doppler radar enables meteorologists to monitor the motion of air in a weather system, rather than merely track areas of precipitation. As a result, Doppler radar has improved their ability to provide warning for severe weather. For example, meteorologists can use Doppler radar to detect the development of a tornado before it descends from its parent thunderstorm and strikes Earth’s surface. In the velocity mode, radar can detect the speed of precipitation or dust particles more than 120 miles (190 kilometers) away. A network of more than 150 Doppler radar stations across the United States began operation in 1997. This network, called NEXRAD, helps forecast severe weather.
Weather balloons, airplanes, and ships.
To obtain information on the state of the atmosphere above Earth’s surface, meteorologists use an instrument package called a radiosonde. The radiosonde is carried aloft by a weather balloon. It measures changes in temperature, pressure, and humidity. A small radio transmitter beams these data back to a weather station. Once the balloon reaches a high enough altitude, it bursts. A parachute then carries the instrument package back to Earth’s surface.
Meteorologists use a special antenna to track a radiosonde. With such tracking, they measure wind speed and direction at different altitudes within the atmosphere. Such an observation is known as a rawinsonde. Radiosonde and rawinsonde observations are made every 12 hours.
Meteorologists sometimes use a dropwindsonde to obtain atmospheric measurements over the oceans. A dropwindsonde is a radiosonde attached to a parachute and dropped from an aircraft. As the instrument package falls toward the sea, it radios measurements back to the aircraft. These measurements include temperature, pressure, and humidity.
Ships also report on weather conditions at sea. Some launch weather balloons. Others release special ocean buoys that record and transmit information about weather at sea level.
Weather satellites
Weather satellites offer advantages over surface weather stations. They can observe weather over a broad and continuous field of view. Surface stations are widely spaced and may not observe some weather systems directly. Furthermore, satellites provide valuable data from the oceans, which cover about 70 percent of the globe. Land-based weather stations provide little information about these vast regions.
Sensors aboard weather satellites detect two types of radiation signals coming from the planet. One signal consists of reflected sunlight. These satellite images, which resemble black-and-white photographs of the planet, reveal cloud patterns.
The second signal recorded by weather satellites is infrared radiation (IR). The intensity of the infrared radiation emitted by an object depends on the object’s temperature. For example, low clouds and fog are relatively warm. They give off more intense infrared radiation than do high clouds, which are relatively cool. Thus, an IR image reveals not only cloud patterns but also cloud temperatures. Objects continually emit infrared radiation. As a result, weather satellites can record IR images at any time—day or night.
There are two main types of weather satellites—geostationary and polar-orbiting. A geostationary satellite orbits about 22,300 miles (35,900 kilometers) above the equator. It travels eastward at the same rate as Earth rotates. Thus, a geostationary satellite remains above the same point on the equator. Because geostationary satellites orbit at such a high altitude, they can record images that cover a wide area. For example, two of them cover most of the United States and Canada. Most satellite images shown on televised weather reports come from this type of satellite.
A polar-orbiting satellite follows a north-south path that takes it over the polar regions. Because the satellite does not rotate east as Earth does, the rotation of Earth causes the satellite to pass over different areas of Earth each orbit. Polar-orbiting satellites travel at a much lower orbit than geostationary satellites. As a result, they record more detailed images.
Weather forecasting
After meteorologists have gathered weather data from around the world, they can try to predict the development and movement of future weather systems. To forecast the weather, meteorologists use such tools as weather maps and mathematical models.
Weather maps.
Meteorologists use weather observation data to create weather maps that represent the state of the atmosphere at a particular time. To capture the three-dimensional nature of weather, they draw maps for conditions at Earth’s surface and at various levels within the atmosphere. By examining a sequence of weather maps, forecasters can determine how the weather changes through time. Then they can make predictions about the future state of the atmosphere.
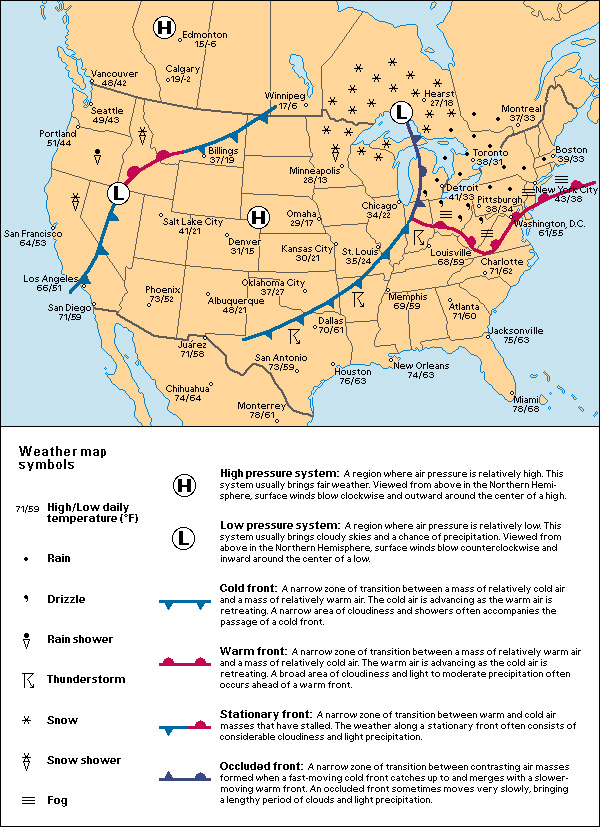
Mathematical models.
Since the mid-1950’s, weather forecasters have used mathematical models of the atmosphere processed by computers. These models improve the accuracy of their predictions. A mathematical model of the atmosphere consists of a set of equations intended to approximate the atmospheric processes that drive the development and movement of weather systems. Mathematical models are based on scientific laws. Through the years, scientists have developed increasingly sophisticated models. Improvements in computer technology have enhanced meteorologists’ ability to use mathematical models effectively. Computers can process enormous amounts of observation data and perform a multitude of calculations rapidly.
A mathematical model begins with the current state of the atmosphere, as determined by the most recent weather observations. The model uses these data to predict the state of the atmosphere for a specific time interval—for example, the next 10 minutes. Using this predicted state as a new starting point, the model then forecasts the state of the atmosphere for another 10-minute period. This process repeats over and over again until the model produces short-range weather forecasts for the next 12, 24, 36, and 48 hours.
The accuracy of weather forecasts generated by mathematical models declines steadily over time for two main reasons. First, the weather observation data initially fed into the model can never provide a complete picture of the present state of the atmosphere. Not all the data are reliable, due to both technical and human error. Data are missing from vast stretches of the atmosphere over the oceans. Second, mathematical models of the atmosphere are only approximations of the way the atmosphere actually works. Errors in the models tend to grow with each repetition.
Meteorologists understand the limitations of mathematical models. They base their forecasts on observations of how the weather has changed over the past several days and on their understanding of atmospheric processes. Experience and even intuition play important roles. So does the cautious interpretation of the output of mathematical models.
Meteorologists also use mathematical models to produce long-range weather forecasts. Long-range forecasts include 6- to 10-day forecasts and monthly (30-day) and seasonal (90-day) outlooks. Long-range forecasts and outlooks typically are less accurate than short-range forecasts. But they can provide an indication of general trends, such as whether conditions will be wetter or drier than normal.
Reporting the weather
The National Weather Service of the United States, the Meteorological Service of Canada, and weather agencies in many other countries issue weather forecasts and hazardous weather alerts to the public through regional forecast offices. The public accesses this information through radio and television broadcasts, newspapers, and the Internet.
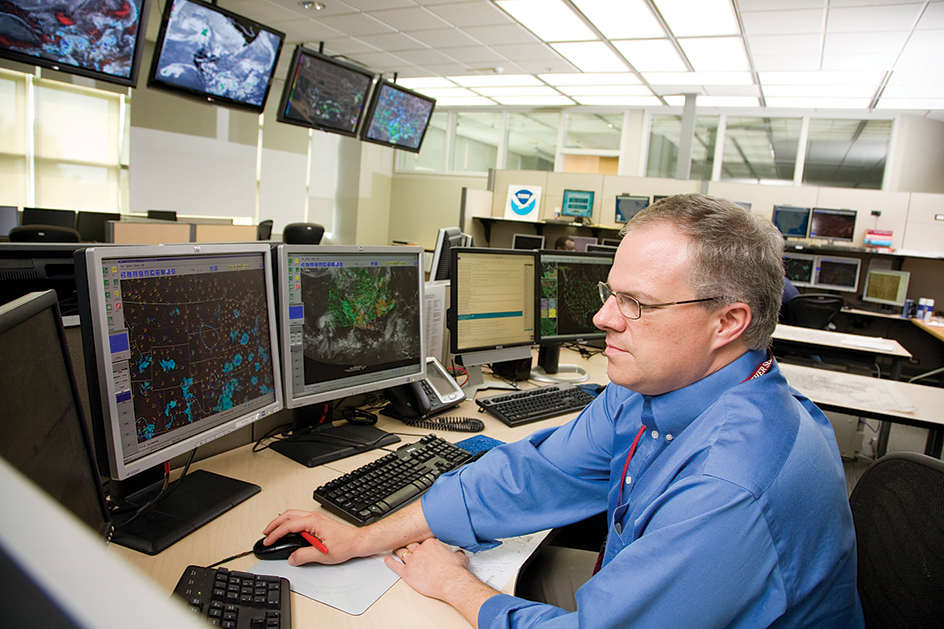
When hazardous weather threatens, forecasters can issue different types of alerts. The U.S. National Weather Service, for example, issues outlooks, watches, warnings, and advisories. An outlook provides notice of a general weather trend. For example, the outlook for spring flooding due to expected snowmelt is available many weeks in advance. A weather watch is issued when hazardous weather is possible based on current or predicted atmospheric conditions. A weather warning applies when hazardous weather is taking place nearby. Watches and warnings are issued for severe thunderstorms, tornadoes, floods, hurricanes, and winter storms, such as blizzards and ice storms.
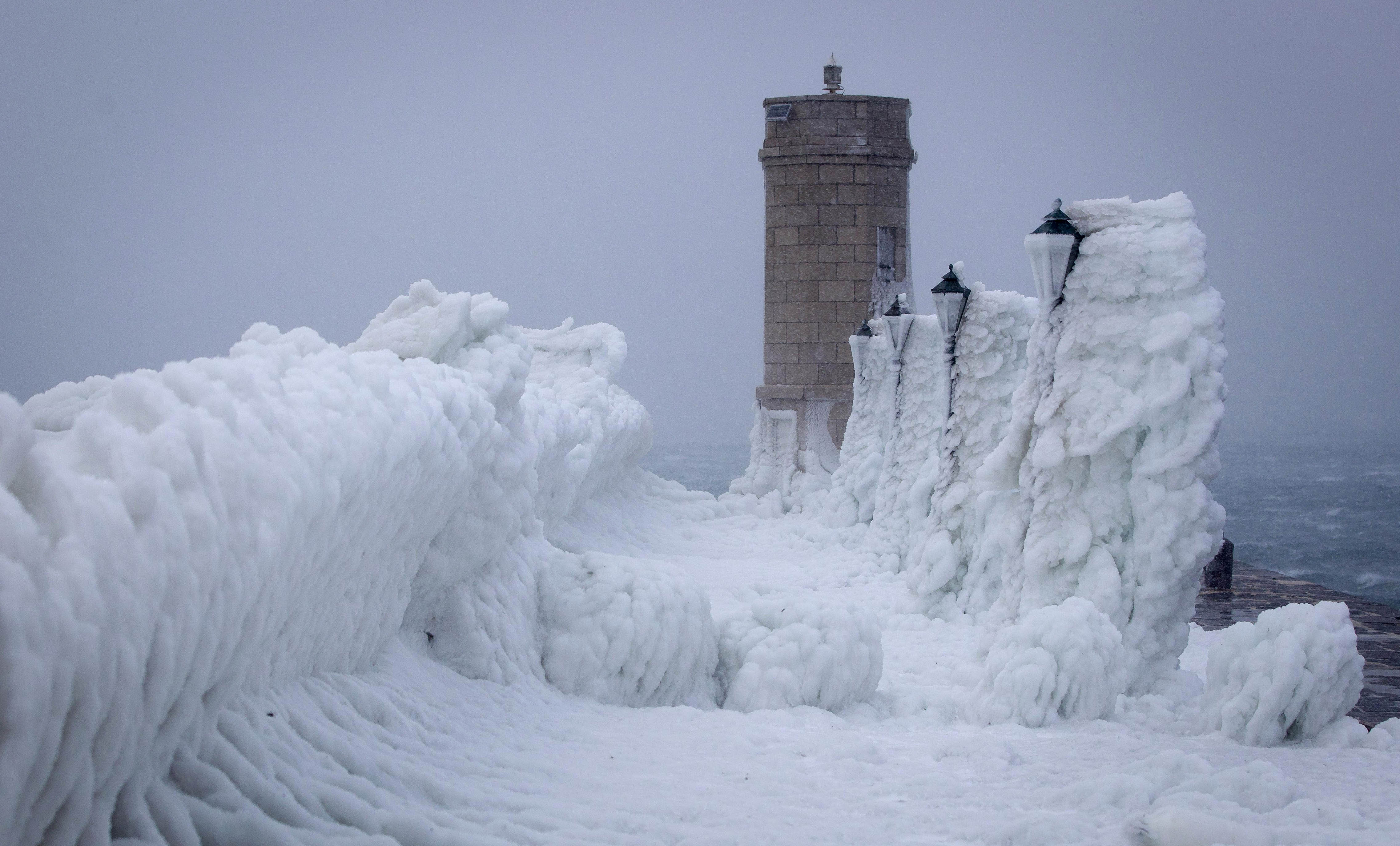
Weather advisories refer to expected weather hazards that are less serious than those covered by a warning. An example is a winter weather advisory.
Weather advisories are also issued for low wind chill temperatures and for a high heat index. Wind chill is a measure of the cooling power of a combination of low air temperature and strong winds. Even if the air temperature remains constant, the human body loses increasing amounts of heat to the environment as wind speed increases. At low wind chill temperatures, people need to take special precautions to prevent frostbite (the freezing of skin tissue) and hypothermia (a dangerous drop in body temperature).
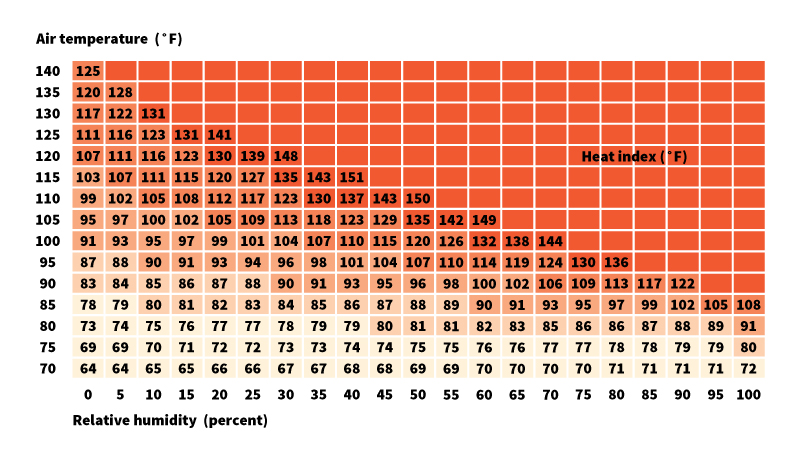
Heat index is a measure of the stress produced by a combination of high air temperature and high relative humidity. During hot and muggy weather, the human body may not be able to release sufficient heat to prevent hyperthermia (a dangerous rise in body temperature). High humidity reduces the rate at which perspiration evaporates from the skin’s surface. The cooling that accompanies this evaporation represents one of the body’s main ways to release heat.
Private weather forecasters provide weather information tailored to a special need. For example, prior to pouring concrete, a building contractor may consult a private forecaster to find out the probability of rainfall during specific hours of the day. Department stores may hire a private forecaster to advise them of prospects for hot weather to ensure the stores have enough air conditioners and fans in stock.
How people affect the weather
Human activities affect the weather both intentionally and unintentionally. For example, the construction of cities creates areas that are drier and warmer than the surrounding countryside. Cities are drier because they have storm sewer systems that carry off rainwater and snowmelt.
Cities are warmer for several reasons. The use of storm drainage systems means that less solar radiation is used to evaporate water. Instead, more radiation heats the city surfaces and air. The brick, asphalt, and concrete surfaces of city buildings, sidewalks, and streets readily transmit the heat they absorb. As a result, they raise urban air temperatures even more. In addition, cities themselves generate heat from a number of sources. These sources include motor vehicles and heating and air conditioning systems.
Large urban areas also affect the weather in the areas downwind of them. Smokestacks and automobile tailpipes in cities emit water vapor and tiny particles that stimulate the formation of clouds. Heat energy rising from a city also spurs the growth of clouds. Thus, the weather downwind from many large urban areas is cloudier and rainier than the weather upwind from those same areas.
Urban and industrial areas also produce air pollutants. These pollutants include carbon monoxide, nitrogen oxides, and hydrocarbons. Although improved controls on factories and motor vehicles have reduced the amount of these gases released into the atmosphere, air quality problems persist. For example, many large cities still have problems with smog. Smog is a mixture of gases and tiny particles that reduces visibility and poses health hazards.
Smog and other air pollution problems are particularly serious in areas where winds are light and a temperature inversion occurs in the lower atmosphere. In a temperature inversion, warm air overlies cold air. As a result, the air temperature rises with increasing altitude. That is the opposite of the usual situation in the troposphere. Under such circumstances, smokestack and tailpipe emissions do not rise and disperse. Emissions may build up to unhealthy concentrations.
Snowflake formation
From time to time, scientists have tried to alter the weather. They have tried to modify hurricanes, suppress hailstorms, and clear fog. Most of these efforts have not worked. Today, scientists focus their weather modification efforts primarily on cloud seeding. Cloud seeding is an attempt to stimulate the natural precipitation-forming process.
Most clouds do not produce rain or snow. This is because few clouds have just the right combination of tiny ice crystals and supercooled water droplets (droplets that remain liquid even at subfreezing temperatures). In such clouds, ice crystals grow at the expense of water droplets and eventually become snow crystals. If the temperature is below freezing all the way to Earth’s surface, precipitation falls as snow. If the air temperature is above freezing, the snow crystals melt into raindrops.
Careers in weather
Students interested in meteorology as a career should take advanced courses in mathematics, computer science, physics, and chemistry. Most entry-level jobs in meteorology require at least a bachelor of science degree. Many require a master’s degree. A limited number of colleges and universities offer degrees in meteorology. The armed forces of the United States and some other countries offer meteorological training as well.
Meteorologists specialize in a number of different areas. For example, research meteorologists study some subfield of meteorology, such as tropical weather systems. Regional forecasters prepare weather forecasts for portions of one or more states or provinces. Consulting meteorologists provide weather information tailored for specific industrial, business, or government needs. Broadcast meteorologists have skills in both meteorology and television or radio broadcasting. This type of meteorologist informs the public of current and expected weather conditions. Specialists called synoptic meteorologists analyze weather observations, interpret the output of computer models, and monitor weather systems. Physical meteorologists study the physical and chemical properties of the atmosphere. Dynamic meteorologists focus on creating models of atmospheric circulation.
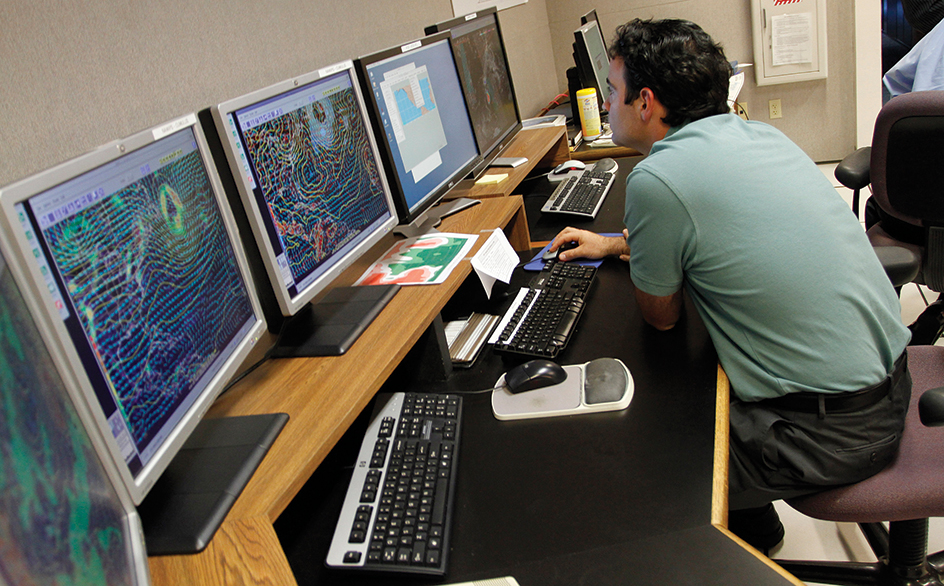