X rays are one of the most useful forms of energy. Their main uses have been in the field of medicine. X rays can pass through flesh and produce images of what lies beneath the skin. X rays also have many uses in science. For example, researchers who investigate materials use X rays to determine the arrangement of atoms in crystals. Astronomers study X rays generated by stars and other heavenly objects to learn about the objects’ structure and temperature.
The German physicist Wilhelm C. Roentgen discovered X rays in 1895. He called them X rays because at first he did not understand what they were. X is a scientific symbol for the unknown.
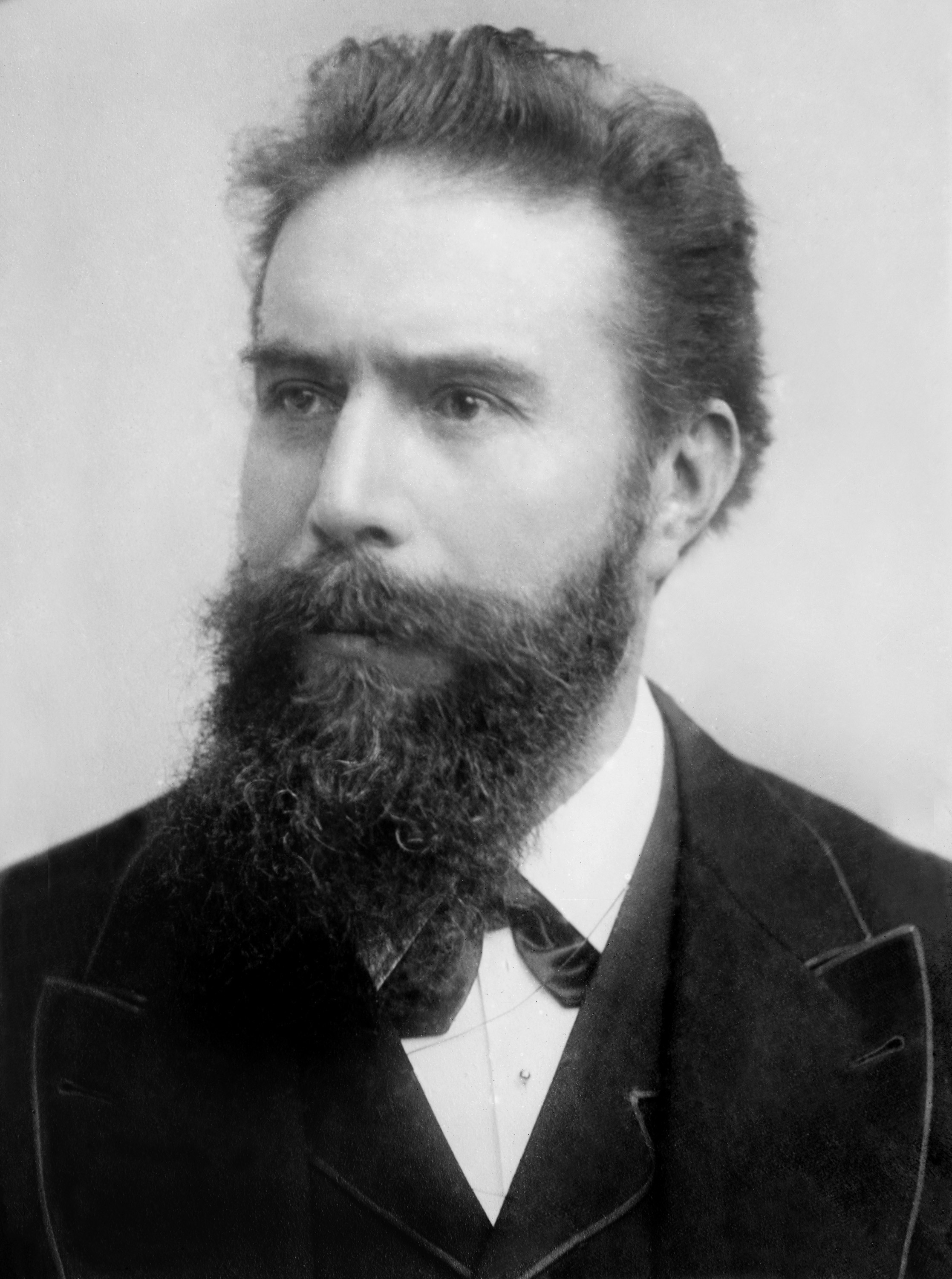
Scientists now know that X rays are one of several forms of electromagnetic radiation. Another form of such radiation is visible light. The various forms can be distinguished by their wavelength. Wavelength is the distance between successive crests of their waves. From the shortest wavelength to the longest, electromagnetic radiation consists of gamma rays, X rays, ultraviolet rays, visible light, infrared rays, and radio waves. Wavelength is related to energy. The shorter the wavelength, the higher the energy. Thus, X rays have a shorter wavelength, but more energy, than do waves of visible light.
X rays can produce biological, chemical, and physical changes in the substances they enter. For this reason, X rays can be dangerous. In human beings, an overdose of X rays may cause cancer, skin burns, anemia, or other serious conditions. But physicians also use X rays to treat cancer. X rays kill cancer cells more readily than they kill normal cells.
In X-ray machines used in medicine and science, a special tube that resembles a television picture tube produces X rays. Scientists also use intense, narrow beams of X rays generated by devices called synchrotrons. In nature, hot stars and other heavenly objects emit (send out) X rays.
Uses of X rays
In medicine,
health workers use X-ray machines to make radiographs (X-ray pictures) of the bones and internal organs of the body. Radiographs help physicians detect injuries and diseases, such as broken bones or tumors, inside a patient’s body. Dentists take X-ray pictures to reveal cavities in teeth.
Because X rays can harm living tissue, health workers who use them in diagnostic work give their patients only as much radiation as necessary. In addition, the workers shield parts of the body not being examined. For example, a dental technician lays a lead apron on a patient’s body before taking X rays of the patient’s teeth.
A health worker produces a radiograph by passing a beam of X rays through the patient’s body onto a sheet of photographic film or a special plastic that is sensitive to X rays. The patient’s bones absorb more rays than do the muscles or internal organs. As a result, the bones cast the sharpest shadows on the film or plastic. Because a radiograph is a photographic negative, the shadows of the bones show up as light areas. Other parts of the body allow more X rays through than the bones do. As a result, they cast shadows in varying shades of gray.
In another process, called digital imaging, detectors measure the X rays that pass through the body. The detectors send this information to a computer. The computer converts the data into an image that is displayed on a monitor.
A machine called a computed tomographic scanner or CT scanner uses X rays to produce cross-sectional images of a patient’s body. The machine shoots a pencil-thin beam of X rays at the body from many angles. Detectors measure the rays that pass through and send the measurements to a computer. The computer then uses the measurements to create the images.
In industry,
inspectors use X rays to examine products made of various materials, including aluminum, steel, and other metals. Radiographs reveal cracks and other defects inside the products. Workers also use X rays to check the quality of mass-produced products, such as computer chips and other small electronic devices. Scanners at airports use X rays to check baggage for weapons or bombs.
In crystal research,
X rays help scientists determine the arrangement of atoms that make up crystals. The atoms in crystals are arranged in planes, with regular spacing between the planes. Crystallographers (scientists who study crystals) pass a beam of X rays through a crystal. The planes act as tiny mirrors. Each crystal diffracts (spreads out) the rays into a geometric pattern. Each type of crystal produces a different diffraction pattern. See X-ray crystallography. Mathematical analysis of such patterns can reveal the precise geometric arrangement of atoms in different crystals. Scientists also use X rays to study the structure and makeup of complex biological molecules known as enzymes and proteins.
In astronomy,
scientists study X rays emitted by extremely hot objects. These objects include tremendous clouds of gas, supernovae (exploding stars), the centers of galaxies, and disks of gas that orbit black holes. A black hole is an invisible object with such powerful gravitational force that not even light can escape its surface. An X ray’s energy depends on the energy of its source. For this reason, astronomers can use information gathered by the detectors to determine the temperatures of the various sources. Because the atmosphere absorbs X rays approaching Earth from cosmic sources, scientists use devices mounted on spacecraft to detect the rays.
Loading the player...X rays from the Crab Nebula
Characteristics of X rays
Wavelength.
X rays have longer wavelengths than gamma rays. But X rays have shorter wavelengths than ultraviolet rays. Scientists working in different fields define the ranges of wavelength differently. In general, however, the ranges for X rays span a band from about 0.01 to 10 nanometers (nm). One nanometer is one billionth of a meter, equal to 1/25,400,000 inch. By comparison, the wavelengths of visible light range from about 400 to 700 nm.
Some scientists refer to X rays with wavelengths shorter than 0.1 nm as hard X rays. Such X rays have great penetrating power. Longer X rays are known as soft X rays. Many scientists who use X rays in their work measure wavelengths in angstrom units. One angstrom unit equals 0.1 nanometer.
Penetrating power.
The penetrating power of X rays is a result of their high energy. Because the energy of X rays is second only to that of gamma rays, only gamma rays have greater penetrating power than X rays.
Any form of electromagnetic radiation will penetrate matter until something in the matter absorbs its energy. In the case of X rays, the absorbing objects are negatively charged particles of matter known as electrons. In an atom, one or more electrons orbit a central nucleus. The nucleus consists of one or more positively charged protons and an approximately equal number of neutrons. Neutrons have no net charge. In a complete atom, the number of electrons equals the atom’s atomic number—the number of protons in the nucleus. Elements whose atoms have high atomic numbers therefore absorb more X rays than do elements with low atomic numbers.
Lead, which has an atomic number of 82, absorbs more X rays than do most other substances. For this reason, lead is often used to make X-ray shields. The metal beryllium, with an atomic number of only 4, absorbs few X rays. This metal is therefore used to make small exit windows in X-ray tubes.
The penetrating power of X rays has affected the design of mirrors used in X-ray telescopes. Only X rays that strike a mirror almost parallel to its surface will reflect strongly from it. The mirror reflects these X rays at a small angle called a grazing incidence angle. The reflection of a ray at a grazing incidence is like the skipping of a stone off the surface of a pond. To focus X rays via grazing incidence, X-ray mirrors have a curved design. They consist of tapered tubes that are nested within one another. The rays enter the mirror almost parallel to the axis of the tubes and reflect off the inner surfaces of the tubes.
The ability of X rays to change substances that they penetrate is also a result of their high energy. When an electron in an atom absorbs energy, it moves to an orbit farther from the atom’s nucleus. If the electron absorbs enough energy, it actually leaves the atom. If the atom had been complete—with equal numbers of protons and electrons—it becomes an ion (electrically charged atom) when the electron leaves it. The process in which an electron absorbs energy and leaves an atom is known as ionization. This process affects the ability of the atom to maintain its position and function in a substance. Chemical bonds may be broken. The shape of molecules may be changed. Thus, if enough ionization occurs in an object—or in living tissue—damage results.
How X rays are produced
In X-ray tubes.
An X-ray tube works by accelerating a beam of electrons to high speeds and directing them into a piece of solid material called a target. The target material absorbs most of the energy of the electrons and converts it to heat. But some of the energy becomes X rays by either of two processes. One process produces what physicists call bremsstrahlung << BREHM shtrah lung >> —the German word for braking radiation. The other process generates characteristic X rays.
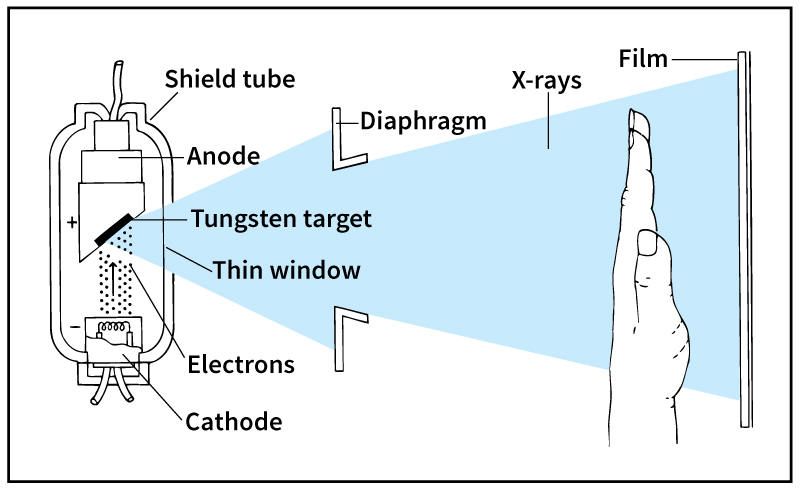
Bremsstrahlung.
The process that produces bremsstrahlung makes use of a fundamental characteristic of charged particles that are in motion: When such a particle changes direction, it emits electromagnetic radiation. The wavelength of the radiation depends on the energy of the particle. It also depends on how sharply the particle’s path bends. The higher the energy and the sharper the bend, the shorter the wavelength.
In an X-ray tube, electrons in the beam change direction after the beam has entered the target. The bending occurs because objects with opposite electric charges attract each other. When an electron in the beam passes near a target nucleus, protons in the nucleus attract the electron. Targets are made of materials whose atoms have high atomic numbers. Their nuclei thus have large numbers of protons. As a result, the attraction between the electron and the protons is strong. The path of the electron therefore bends sharply.
Characteristic X rays.
The second process begins when an electron in the beam transfers much of its energy to an electron in a target atom. The electrons in the target atom orbit the nucleus in groups called shells. Electrons with the lowest energy are in the shell closest to the nucleus. Electrons with successively higher energies are in shells that are farther and farther from the nucleus. In the target atom, the electron that absorbs the energy is usually in the closest shell. It absorbs so much energy that it leaves the atom.
After this electron departs, another electron in the target atom tends to take its place. But the replacement electron is in a shell that is farther from the nucleus than the departed electron had been. Therefore, to replace the departed electron, the replacement electron must give up the difference in energy between the two shells. It does so by emitting electromagnetic radiation in the form of an X ray. The energy of the X ray is characteristic of the target atom. That is, target atoms of a given chemical element emit X rays that have the same energy.
Synchrotron radiation.
The most intense beams of X rays produced in machines come from synchrotrons. A synchrotron consists of straight and curved sections arranged in a ring. In the straight sections, radio waves accelerate a beam of electrons or other charged particles to high speeds. Electromagnets in the curved sections guide the beam around the ring. This method of guidance works because a charged particle will change its direction when it crosses a magnetic field (a region in which the force of magnetism can be detected). When a particle changes direction, it also emits electromagnetic radiation known as synchrotron radiation.
Scientists built the first synchrotrons in the 1940’s to study subatomic particles. In those machines and in similar machines built since then, the particle beam strikes a target. The resulting impacts break particles away from the target or produce new particles. Special devices detect the particles that fly away from the points of impact. Physicists then study the information provided by the detectors. Synchrotron radiation plays no role in such experiments. In fact, it is unwanted because the machine must make up for the energy that is radiated away.

However, synchrotron radiation has proved useful to crystallographers and other scientists who investigate the structure of matter. It has been so useful that, since the 1970’s, scientists have built machines just to produce synchrotron radiation in the ultraviolet and X-ray ranges. There are now about 50 such synchrotron sources in the world.
The radiation comes from insertion devices (ID’s) mounted in some of the straight sections. An ID consists mainly of two sets of several permanent magnets. Each set is arranged so that magnetic south poles alternate with magnetic north poles. One set is mounted above the beam path. The other is below the path. When an electron beam passes through the magnetic fields, the electrons change direction again and again. Each time they do so, they emit synchrotron radiation.
In outer space,
the main sources of X rays are extremely hot objects. The X rays originate in the vibrations of atoms. The atoms of any object vibrate at various speeds. Each time an atom vibrates, some of its energy of motion is transferred to one or more electrons. The electrons then return to their former energy levels by emitting electromagnetic radiation. The more rapid the vibration, the shorter the wavelength of the emission. The object therefore emits radiation at various wavelengths. But some of these wavelengths carry more of the total energy that is emitted by the object than do the other wavelengths. The higher the temperature, the shorter are the wavelengths that carry the most energy.
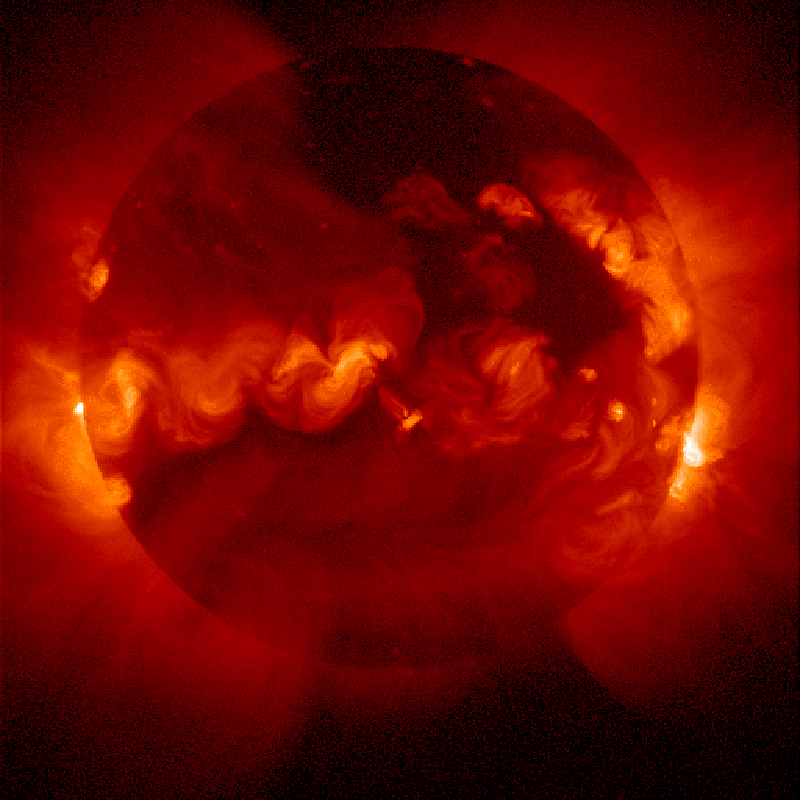
Celestial objects with temperatures of about 1 million °C have peak wavelengths in the ultraviolet range. But they are also powerful emitters of X rays. One leading type of X-ray emitter is an X-ray binary. An X-ray binary consists of two stars that orbit each other at close range. One of the stars is a normal star. The other is a neutron star, the smallest and densest kind of star known. The tremendous gravitational force of the neutron star pulls matter off the normal star. This matter forms a large disk around the neutron star. Friction heats the disk to more than 1 million °C. As a result, it emits X rays.